Chapter 17 Differences in Neuromuscular Characteristics between Male and Female Athletes
INTRODUCTION
Joint stability may be defined as the state of a joint remaining or promptly returning to proper alignment through an equalization of forces.71 This requires a synergy between bones, joint capsules, ligaments, muscles, tendons, and sensory receptors.83 The static components of joint stability include the ligaments, joint capsule, cartilage, friction, and the bony geometry of the articulation.35,48 These components are typically assessed through joint stress testing and have commonly defined clinical joint stability.71 These static components provide the foundation for joint stability during functional activities by guiding joint arthrokinematics. However, during physically demanding tasks such as running, jumping, and cutting, these components may not provide the restraint necessary to prevent joint injury. During these tasks, stability is provided by dynamic components that include the neuromuscular control of the skeletal muscles crossing the joint.71 The functional joint stability accomplished through the integration and complementary relationship between the static and the dynamic components of joint stability is referred to as dynamic joint stability.
Dynamic joint stability is influenced by the neuromuscular control of the muscles crossing the joint. Relative to joint stability, neuromuscular control is the unconscious activation of the dynamic restraints occurring in preparation for and in response to joint motion and loading for the purpose of maintaining and restoring functional joint stability.71 Neuromuscular control of joint stability is a complex interaction between components of the nervous system and those of the musculoskeletal system and typically is accomplished through two different control systems, feedback and feed-forward control.23 In a system that uses feedback control, sensors continually measure the parameter of interest based on an optimal value. A deviation from this optimal value will initiate an error signal. In response to this error signal, the system will trigger a compensatory response. Feed-forward systems also require measurement of a parameter, but measurement occurs only intermittently. The sensory components of this system are designed to measure a potential disturbance or change in the parameter of interest. Once a potential disturbance has been detected, the system initiates an error signal. In response to this error signal, the system institutes commands to counteract the anticipated effects of the disturbance. The commands instituted by this system are largely shaped by previous experience with similar disturbances. Feed-forward control systems are considered to be anticipatory in nature compared with feedback control systems, which are characterized by responses only to current stimulus. Both are essential for optimal maintenance of dynamic knee stability.
NONCONTACT ANTERIOR CRUCIATE LIGAMENT INJURIES AND FEMALE ATHLETES
The role of dynamic joint stability in the prevention of knee injuries has been a focus of research at the University of Pittsburgh and the Neuromuscular Research Laboratory since 1995 in response to the demonstrated differences in noncontact anterior cruciate ligament (ACL) injury rates between male and female athletes. Female athletes suffer ACL injuries at a significantly higher rate than male athletes in matched sports.1–3,9,15,17,26–28,59,63 The majority of these injuries occur through a noncontact mechanism of injury in which no external forces are applied directly to the knee joint.10,57,64 The forces that cause the injury are applied to the knee joint through ground reaction forces and internal soft tissue and muscle forces. An examination of gender-specific characteristics is an important first step in determining potential risk factors and prevention of these injuries. Researchers have investigated numerous potential risk factors for noncontact ACL injuries including anatomic, hormonal, environmental, neuromuscular, and biomechanical characteristics. The primary and current focus in female noncontact ACL injury prevention (risk factor identification and training) is on the neuromuscular and biomechanical factors of joint stability owing to their potential for modification through intervention programs.12,29,31,50,52,62,70,87
PROPRIOCEPTION
Proprioception is the afferent information arising from the internal peripheral areas of the body that contribute to postural control, joint stability, and conscious sensations. These include the conscious submodalities of proprioception: joint position sense, active and passive kinesthesia, the sense of heaviness or resistance, and appreciation of movement velocity. Proprioception is an important component to establish and maintain functional joint stability.45,67 Alterations in the acquisition, processing, and integration of proprioceptive information can affect functional joint stability. The presence of knee joint proprioception deficits in females may contribute to their increased rate of ACL injury, because the lack of proprioception inhibits recruitment of the dynamic stabilizers that prevent anterior tibial translation.
Critical Points PROPRIOCEPTION
The proprioceptive characteristics of male and female athletes were examined in 34 collegiate-level athletes.75 Knee joint proprioception was measured by assessing the threshold to detect passive motion with a custom-built testing device (Department of Engineering, University of Pittsburgh). The device was capable of rotating the knee joint at 0.5°/sec, which is necessary to reduce the input of musculotendinous mechanoreceptors. Subjects were tested in a seated position while wearing a pneumatic sleeve on their tested lower leg, a blindfold over their eyes, and headphones with white noise in order to reduce cutaneous input, visual input, and auditory input, respectively (Fig. 17-1). Participants were instructed to signal (with a button signal) when joint motion was sensed. Direction of movement was randomized across six trials (three in each direction), with data averaged for each direction. The amount of rotation before the participants’ signal was recorded as the threshold to detect passive motion. Testing was performed at a knee flexion angle of 15° with movement into both flexion and extension. This position was chosen because most noncontact ACL injuries occur with the knee close to full extension.10,57,65 Reliability of the device was demonstrated to be high.47
No differences were observed between male and female athletes when the knee was rotated into flexion, but a significant difference was found when the knee was rotated toward full extension (Fig. 17-2). The ACL becomes tauter as the knee extends, and although the female subjects in this study had greater knee joint laxity, this may provide evidence for the increased risk of ACL injury (noncontact) in female athletes. The decreased ability to detect motion toward a dangerous position10,57,65 of full extension could prevent preactivation of protective muscle forces such as the hamstrings.
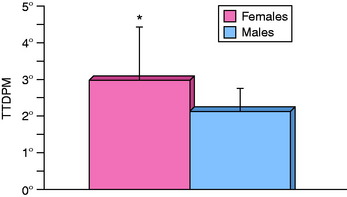
FIGURE 17-2 Gender differences in TTDPM (*P = .039).
(From Rozzi, S. L.; Lephart, S. M.; Gear, W. S.; Fu, F. H.: Knee joint laxity and neuromuscular characteristics of male and female soccer and basketball players. Am J Sports Med 27:312–319, 1999.)
Although proprioception differences exist between genders, differences also exist in proprioception abilities within the female athletic population.44 A comparison of the threshold to detect passive motion was conducted between female gymnasts and a group of healthy, age-matched participants. The instrumentation and methodology of testing were similar to that of the gender comparison study described previously. Gymnasts recognized movement significantly faster than the nongymnasts (Fig. 17-3). It is recognized that the superior kinesthetic awareness observed in the gymnasts may be due to one or multiple factors:
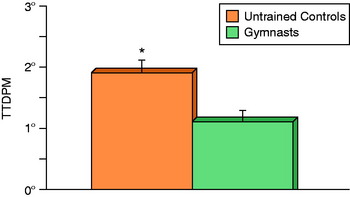
FIGURE 17-3 Gymnasts demonstrate superior TTDPM compared with untrained controls (*P = .011).
(From Lephart, S. M.; Giraldo, J. L.; Borsa, P. A.; Fu, F. H.: Knee joint proprioception: a comparison between female intercollegiate gymnasts and controls. Knee Surg Sports Traumatol Arthrosc 4:121–124, 1996.)
BALANCE AND POSTURAL STABILITY
Postural stability is the ability to maintain the body in equilibrium by maintaining the projected center of mass within the limits of the base of support.81 Similar to joint stability, maintenance of postural stability is a dynamic process that involves establishing an equilibrium between destabilizing and stabilizing forces.56 Sensory information for this stability is derived from vision, the vestibular system, and somatosensory feedback.38 Somatosensory feedback is also necessary for dynamic knee stability and is incorporated into the sensorimotor system in order to maintain stability both in dynamic tasks and in static posture.38,71 Although researchers have cautioned against extrapolating data from static conditions to dynamic athletic situations, postural stability testing can provide valuable information regarding sensory integration, processing, and motor planning.85
The sensorimotor system provides the sensory information and action components that influence the perception, cognition, and action processes that are paramount to dynamic knee stability.71 Sensorimotor processes involved in dynamic knee stability include the neurosensory and neuromuscular mechanisms involved in the acquisition of a sensory stimulus, the conversion of the stimulus to a neural signal, transmission of the signal to the central nervous system (CNS), processing and integration of the signal within the CNS, and the motor responses resulting in muscle activation for joint stability during locomotion and functional tasks.46 Accurate sensory information is critical to this system.71 Without accurate sensory information, the proper corrective actions cannot be planned and executed.
Overall, the authors’ research demonstrates that female athletes have superior balance than male athletes. A study was conducted that measured and compared the balance of male college-aged athletes and female National Collegiate Athletic Association (NCAA) Division I athletes using the Biodex Stability System (Biodex, Inc., Shirley, NY).75 The Biodex software generates a stability index value that is based on the amount of time the platform is off-level. Female athletes had a significantly greater stability index than their male counterparts (Fig. 17-4). In another study, single-leg balance was measured on a traditional force plate (Kistler Corporation, Worthington, OH; Model #4060-1011000).80 Testing methodology and data processing were based on that described by Goldie and coworkers24,25 and included both an eyes-closed and an eyes-open condition. Comparisons were made between male and female high school basketball players. Females demonstrated significantly better single-leg balance scores for both the eyes-closed and the eyes-opened conditions (Fig. 17-5).
Critical Points BALANCE AND POSTURAL STABILITY
It is interesting to note that many noncontact ACL injury prevention training programs include activities designed to improve balance12,29,31,42,51,62 despite the fact that females have significantly better single-leg balance and that single-leg balance deficits have not been identified as a risk factor for ACL injury. The lack of balance deficits seems to contradict the research demonstrating that females have proprioceptive deficits. The authors proposed that the measurement of static single-leg balance might not adequately challenge the underlying motor control and peripheral somatosensory system. A more dynamic test of postural control would be more appropriate and likely to reveal potential deficits related to the acquisition, processing, and integration of somatosensory information and functional joint stability. Currently, the authors are conducting a study examining single-leg balance (static) and dynamic postural control. The goals of this study are to establish the reliability of a test of dynamic postural control, determine the relationships between static balance tests and dynamic balance tests, and determine whether gender differences are present in dynamic postural control.
EMG ACTIVITY
The electromyogram measures the electrical manifestation of the contracting muscle.4 It is the electrical signal that propagates from the neuromuscular junction along the muscle fiber and is termed the muscle action potential.33 Measurement of EMG activity provides information regarding the amount of electrical activity in the contracting muscle, which provides insight into the magnitude of tension developed.86 Many variables can influence this signal and thus create difficulty in interpreting these data. These variables include velocity of muscle shortening or lengthening, rate of tension buildup, fatigue, and reflex activity.89 Muscle activation patterns, amplitude, and quantity provide important insight into the neuromuscular control of joint stability.
Dynamic knee stability is dependent on the neuromuscular control over the musculature of the knee in order to reduce strain in the ACL. For this control to be effective, the CNS must be able to anticipate destabilizing forces and act appropriately.8 Benvenuti and associates6 examined EMG activity of upper arm musculature during reaction-time arm movements and demonstrated that when destabilizing forces are anticipated, the CNS is capable of adjusting muscle activation patterns to oppose these forces, supporting the notion that anticipatory postural adjustments are planned in detail. EMH activity of the knee musculature has been investigated and compared between genders during athletic tasks in an attempt to quantify the role of the knee extensors and flexors in dynamic knee stability.7,14,49
The authors measured the EMG activity of the vastus medialis, vastus lateralis, medial hamstrings, lateral hamstrings, and gastrocnemius with surface electrodes while athletes performed a drop-land task.75 Comparisons between genders were performed for the activity onset time after initial contact and peak amplitude after initial contact. In addition, the area of activity (integrated) for the first 1 second of the contraction after landing was calculated for each muscle. No significant differences were observed for onset time for any of the muscles. Analysis of the peak amplitude and integrated electromyographic (IEMG) activity revealed a significant difference between genders for the lateral hamstrings; females had a greater peak amplitude and IEMG activity (Fig. 17-6) than males. Activation of the lateral hamstrings in response to the landing is an attempt by female athletes to prevent the anterior tibial translation that occurs as the knee flexes. It may also be an attempt to counteract potential anterolateral subluxations due to its insertion on the fibular head.
Gender differences have also been observed in EMG activation during reactive stop-jump tasks and stop-jump tasks designed to simulate tasks during which noncontact ACL injuries occur.78