CHAPTER 58 Diaphragm
The diaphragm is a curved musculofibrous sheet that separates the thoracic from the abdominal cavity (Figs 58.1, 58.2). Its mainly convex upper surface faces the thorax, and its concave inferior surface is directed towards the abdomen. The positions of the domes or cupolae of the diaphragm are extremely variable because they depend on body build and the phase of ventilation. Thus the diaphragm will be higher in short, fat people than in tall, thin people, and overinflation of the lung, as occurs for example in emphysema, causes marked depression of the diaphragm. Usually, after forced expiration the right cupola is level anteriorly with the fourth costal cartilage and therefore the right nipple, whereas the left cupola lies approximately one rib lower. With maximal inspiration, the cupola will descend as much as 10 cm, and on a plain chest radiograph the right dome coincides with the tip of the sixth rib. In the supine position, the diaphragm will be higher than in the erect position, and when the body is lying on one side, the dependent half of the diaphragm will be considerably higher than the uppermost one.
ATTACHMENTS AND COMPONENTS
The muscle fibres of the diaphragm arise from the highly oblique circumference of the thoracic outlet: the attachments are low posteriorly and laterally, but high anteriorly. Although it is a continuous sheet, the muscle can be considered in three parts, sternal, costal and lumbar, which are based on the regions of peripheral attachment. The sternal part arises by two fleshy slips from the back of the xiphoid process, and is not always present. The costal part arises from the internal surfaces of the lower six costal cartilages and their adjoining ribs on each side, and interdigitates with transversus abdominis (see Fig. 54.14). The lumbar part arises from two aponeurotic arches, the medial and lateral arcuate ligaments (sometimes termed lumbocostal arches) and from the lumbar vertebrae by two pillars or crura.
RELATIONS
The upper surface of the diaphragm is related to three serous membranes. On each side, the pleura separates it from the base of the corresponding lung, and the pericardium is interposed between the middle folium of the central tendon and the heart. This latter area, which is almost flat, is referred to as the cardiac plateau and extends more to the left than the right. In anteroposterior view, the superior profile of the diaphragm rises on either side of the cardiac plateau to a smooth convex dome or cupola, the cupola on the right being higher and slightly broader than that on the left. Most of the inferior surface is covered by peritoneum. The right side is accurately moulded over the convex surface of the right lobe of the liver, the right kidney and right suprarenal gland. The left side conforms to the left lobe of the liver, the fundus of the stomach, the spleen, the left kidney and the left suprarenal (adrenal) gland. In view of these differences in the profile and anatomical relationships of the right and left sides of the diaphragm, the side should always be specified in clinical descriptions. At full inspiration, the right hemidiaphragm is found at the anterior sixth rib on a posteroanterior chest radiograph; the left hemidiaphragm is 1.5–2.5 cm lower than the right (see Fig. 55.16). Unilateral paralysis may be seen as a raised hemidiaphragm on a chest radiograph, but this sign should not be relied upon uncritically in clinical practice.
APERTURES
A number of structures pass between the thorax and abdomen via apertures in the diaphragm. There are three large openings, for the aorta, oesophagus and inferior vena cava, and a number of smaller ones (Fig. 58.2).
The oesophageal aperture is located at the level of the tenth thoracic vertebra, above, in front and a little to the left of, the aortic opening. It transmits the oesophagus, gastric nerves, oesophageal branches of the left gastric vessels and some lymphatic vessels. The elliptical opening has a slightly oblique long axis, and is bounded by muscle fibres that originate in the medial part of the right crus and cross the midline, forming a ‘chimney’ approximately 2.5 cm long, which accommodates the terminal portions of the oesophagus. The outermost fibres run in a craniocaudal direction, and the innermost fibres are arranged circumferentially. There is no direct continuity between the oesophageal wall and the muscle around the oesophageal opening. The fascia on the inferior surface of the diaphragm is continuous with the transversalis fascia and is rich in elastic fibres. It extends upwards into the opening as a flattened cone to blend with the wall of the oesophagus 2–3 cm above the oesophago-gastric (squamocolumnar) junction. Some of its elastic fibres penetrate to the submucosa of the oesophagus. This peri-oesophageal areolar tissue is referred to as the phreno-oesophageal ligament. It connects the oesophagus flexibly to the diaphragm, permitting some freedom of movement during swallowing and ventilation while at the same time limiting upward displacement of the oesophagus.
On each side of the diaphragm there are small areas where the muscle fibres are replaced by areolar tissue. One, between the sternal and costal parts, contains the superior epigastric branch of the internal thoracic artery and some lymph vessels from the abdominal wall and convex surface of the liver. The other, between the costal part and the fibres that spring from the lateral arcuate ligament, is less constant; when it is present, the posterosuperior surface of the kidney is separated from the pleura only by areolar tissue.
Reflux of gastric contents into the oesophagus, with risk of inhalation into the lungs, is normally prevented by a physiological antireflux barrier located at the gastro-oesophageal junction. The major components of this barrier are the specialized smooth muscle of the wall of the lower oesophagus and the encircling fibres of the crural diaphragm. See also Chapter 65.
The diaphragm lends additional power to all expulsive efforts: sneezing, coughing, laughing, crying, urinating, defecating, and expelling the fetus from the uterus are all preceded by a deep inspiration. Similarly, a deep inspiration followed by closure of the glottis is a common preliminary to powerful recruitment of the trunk muscles, e.g. in lifting heavy weights, because the increased intra-abdominal pressure provides pneumatic bracing of the vertebral column. Repeated stress may eventually compromise the integrity of the hiatus, so that the muscular hiatal tunnel widens. Concomitant laxity of the phreno-oesophageal membrane allows the gastro-oesophageal junction to slide into the thorax; this is usually termed a sliding, or type I, hiatus hernia (Kahrilas 2001). Sliding hernias are typically acquired. They commonly occur in the fifth decade of life and are found in more than 50% of patients with gastro-oesophageal reflux, a condition that induces tonic contraction of the longitudinal oesophageal muscle, which further exacerbates the hiatus hernia. When the stomach herniates into the thorax alongside the oesophagus, it is termed a para-oesophageal, or type II, hiatus hernia.
Closed and penetrating thoracoabdominal injuries may result in rupture or laceration of the diaphragm. With closed injuries and diaphragmatic rupture, there may be subsequent herniation of the abdominal contents into the thorax. Spiral CT with planar reformation should be the primary investigation (Shanmuganathan et al 2000): MRI is usually performed when other imaging modalities have produced equivocal findings. Early operative repair is recommended in diaphragmatic trauma, because untreated cases are at risk of developing gastrointestinal obstruction or perforation. Patients with penetrating injuries may require additional assessment by thoracoscopy (Lowdermilk & Naunheim 2000).
VASCULAR SUPPLY
ARTERIES
The lower five intercostal and subcostal arteries supply the costal margins of the diaphragm while the phrenic arteries supply the main central portion of the diaphragm. The anastomotic arrangement (see p. 1010) ensures profuse blood supply.
Phrenic arteries
Two small phrenic vessels help to supply the diaphragm via the abdominal surface. They may arise separately from the aorta, just above its coeliac trunk, or by a common aortic stem, or from the coeliac trunk. Occasionally, one is from the aorta, the other from a renal artery. Each artery ascends laterally anterior to a crus of the diaphragm, near the medial border of the suprarenal gland. The left passes behind the oesophagus and then runs forwards on the left side of its diaphragmatic opening. The right phrenic artery passes posterior to the inferior vena cava, and then along the right side of its opening. Each phrenic artery divides into medial and lateral branches near the posterior border of the central tendon. The medial branch curves forwards to anastomose with its contralateral fellow in front of the central tendon and with the musculophrenic and pericardiacophrenic arteries. The lateral branch approaches the thoracic wall, and anastomoses with the lower posterior intercostal and musculophrenic arteries. The lateral branch of the right artery supplies the inferior vena cava, whereas the left sends ascending branches to the oesophagus. Invariably there is a communicating artery between the left gastric and inferior phrenic arteries.
INNERVATION
The diaphragm receives its motor supply via the phrenic nerves (Fig. 58.3). Sensory fibres are distributed to the peripheral part of the muscle by the lower six or seven intercostal nerves. The right crus of the diaphragm, the fibres of which divide to the right and left of the oesophageal opening, is innervated by both right and left phrenic nerves. There is some evidence that the crural fibres contract slightly before the costal part, and this may be functionally significant for non-respiratory tasks (Sharshar et al 2005).
Phrenic nerve
The phrenic nerve is a mixed nerve that provides the sole motor supply to the diaphragm. It is derived mostly from the fourth cervical ramus, but also receives contributions from the third and fifth cervical rami (see Fig. 43.6). The course of the cervical part of the phrenic nerve is described on page 456. Within the thorax, the phrenic nerve descends anterior to the pulmonary hilum, between the fibrous pericardium and mediastinal pleura, to the diaphragm, accompanied by the pericardiophrenic vessels. In its thoracic course, each phrenic nerve supplies sensory branches to the mediastinal pleura, fibrous pericardium and parietal serous pericardium. The right and left phrenic nerves differ in their intrathoracic relationships (Rajanna 1947).
Right phrenic nerve
The right phrenic nerve is shorter and more vertical than the left, and is separated at the root of the neck from the second part of the right subclavian artery by scalenus anterior. It subsequently descends lateral to the right brachiocephalic vein, the superior vena cava and the fibrous pericardium that covers the right surface of the right atrium and inferior vena cava, and divides just above or at the level of the diaphragm (Fig. 58.2).
Left phrenic nerve
At the root of the neck, the left phrenic nerve is commonly stated to leave the medial edge of scalenus anterior and to pass anterior to the first part of the left subclavian artery and behind the thoracic duct. However, sometimes the right and left nerves are symmetric in their cervical course, and so the left phrenic nerve may cross anterior to the second part of the left subclavian artery, separated from it by scalenus anterior at the level of the thoracic inlet. Thereafter, the left phrenic nerve crosses anterior to the left internal thoracic artery, descending across the medial aspect of the apex of the left lung and its pleura to the first part of the subclavian artery, which it crosses obliquely to reach a groove between the left common carotid and subclavian arteries. It passes anteromedially, superficial to the left vagus nerve just above the aortic arch and behind the left brachiocephalic vein, and then passes superficial to the aortic arch and the left superior intercostal vein, anterior to the left pulmonary hilum, to lie between the fibrous pericardium covering the surface of the left ventricle and the mediastinal pleura (see Fig. 56.1).
Diaphragmatic relationships
The right phrenic nerve passes through the central tendon of the diaphragm, either by the caval aperture or just lateral to it. The left phrenic nerve passes through the muscular part of the diaphragm anterior to the central tendon, just lateral to the left cardiac surface and more anterior than the right phrenic nerve (Fig. 58.2). At the diaphragm or slightly above it, each phrenic nerve supplies fine branches to the parietal pleura above, and the parietal peritoneum below, the central diaphragm. Typically, the trunk of each nerve then divides into three branches as it passes through the diaphragm. These are commonly arranged as follows (with some variation): an anterior (sternal) branch, which runs anteromedially towards the sternum and connects with its fellow; an anterolateral branch, which runs laterally anterior to the lateral leaf of the central tendon; a short posterior branch, which divides into a posterolateral ramus coursing behind the lateral leaf and a posterior (crural) ramus which supplies the crural fibres. Posterolateral and crural branches may arise separately from the phrenic nerve. These main branches may be submerged in diaphragmatic muscle or lie below it. They supply motor fibres to the muscle and sensory fibres to the peritoneum and pleura related to the central part of the diaphragm. They also relay proprioceptive fibres from the musculature. The right phrenic nerve supplies the part of the right crus to the right of the oesophagus, and the left phrenic nerve supplies the left crus and the part of the right crus that lies on the left of the oesophagus. Phrenic rami connect with branches of the coeliac plexuses on the inferior surface of the diaphragm, and there is a small phrenic ganglion on the right, at the junction of the plexuses. Rami from the plexuses supply the suprarenal glands and, on the right, the hepatic falciform and coronary ligaments, the inferior vena cava and, possibly (via connections with coeliac and hepatic plexuses), the gallbladder.
Lesions of the phrenic nerve
Division of the phrenic nerve in the neck completely paralyses the corresponding half of the diaphragm, which atrophies. If an accessory phrenic nerve exists, section or crushing of the main nerve as it lies on scalenus anterior will not produce complete paralysis. The phrenic nerve may be involved with traumatic lesions of the upper brachial plexus. Historically, it was deliberately injured in order to collapse, and hence rest, the lung in patients with pulmonary tuberculosis. Cardiac surgery is one of the most common medical causes of phrenic nerve injury, especially as a result of the instillation of saline slush for myocardial preservation. Other causes include thoracic surgery, tumours of the lung or mediastinum, and infections such as polio. Respiratory muscle weakness of rapid onset is a feature of Guillain–Barré syndrome.
ANATOMY OF BREATHING
Breathing is a highly coordinated abdominal and thoracic process. The diaphragm is the major muscle of inspiration, responsible for approximately two-thirds of quiet breathing in healthy humans. The external intercostal muscles are most active in inspiration, and the internal intercostals, which are not as strong, are most active in expiration. Increasing the vertical, transverse and anteroposterior dimensions of the chest increases the volume of the pleural space, and the resulting decrease in intrapleural pressure draws air into the lungs. During expiration, the diaphragm relaxes and moves superiorly. Air is expelled from the lungs and the elastic recoil of the lung creates a subatmospheric pressure that returns the lateral and anteroposterior dimensions of the thorax to normal (De Troyer & Estenne 1988, Celli 1998).
During inspiration, the lowest ribs are fixed, and contraction of the diaphragm draws the central tendon downwards. In this movement, the curvature of the diaphragm is scarcely altered. The cupolae move downwards and a little forwards almost parallel to their original positions. The associated downward displacement of the abdominal viscera is permitted by the extensibility of the abdominal wall, but the limit of this extensibility is soon reached. The central tendon, its motion arrested by the abdominal viscera, then becomes a fixed point from which the fibres of the diaphragm continue to contract. This causes the second to tenth ribs to be elevated and the inferior portions of the ribs are turned outwards as a result of direct transmission of pressure through the zone of apposition (Fig. 58.4). The medial aspect of the rib is elevated and this increases the transverse dimension of the chest in the same manner as a bucket handle swinging outwards (Fig. 58.5A). This effect is most evident in the lower ribs (seventh to tenth ribs). Movements at the costovertebral joints cause elevation of the anterior ends of the ribs that push the body of the sternum and the upper ribs forwards. This ‘pump handle’ movement is most evident in the superior ribs (second to sixth ribs) and increases the anteroposterior dimension of the thorax (Fig. 58.5B). The right cupola of the diaphragm, which lies on the liver, has a greater resistance to overcome than the left, which lies over the stomach, and so the right crus and the fibres of the right side are more substantial than those of the left. The balance between descent of the diaphragm, protrusion of the abdominal wall (‘abdominal’ breathing), and elevation of the ribs (‘thoracic’ breathing) varies in different individuals and with the depth of ventilation. The thoracic element is usually more marked in females, but increases in both sexes during deep inspiration.
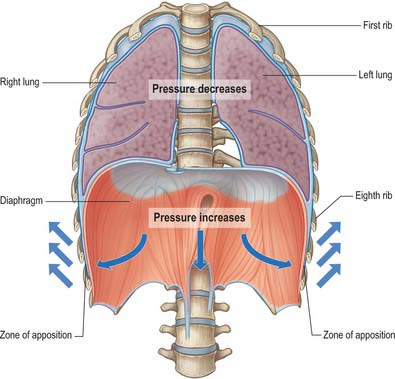
Fig. 58.4 Inspiratory movements: pressure changes during inspiration.
(Adapted from Drake, Vogl and Mitchell 2005.)
Bohn D. Congenital diaphragmatic hernia. Am J Respir Crit Care Med. 2002;166:911-915.
Reviews the epidemiology, diagnosis and treatment of congenital diaphragmatic hernia..
Celli B. The diaphragm and respiratory muscles. Chest Surg Clin N Am. 1998;8:207-224.
De Troyer A, Estenne M. Functional anatomy of the respiratory muscles. Clin Chest Med. 1988;9:175-193.
Kahrilas PJ. Supraesophageal complications of reflux disease and hiatal hernia. Am J Med. 2001;111(suppl 8A):51-55.
Reviews gastro-oesophageal reflux and type I sliding hiatus hernia..
Lowdermilk GA, Naunheim KS. Thoracoscopic evaluation and treatment of thoracic trauma. Surg Clin North Am. 2000;80:1535-1542.
Discusses the additional benefit and role of video-assisted thoracoscopy in thoracic trauma..
Paterson WG. The normal antireflux mechanism. Chest Surg Clin N Am. 2001;11:473-483.
Rajanna MJ. Anatomical and surgical considerations of the phrenic and accessory phrenic nerves. J Int Coll Surg. 1947;60:42-52.
Describes the anatomical course of the phrenic nerve in 203 cadaveric dissections..
Shanmuganathan K, Killeen K, Mirvis SE, White CS. Imaging of diaphragmatic injuries. J Thorac Imaging. 2000;15:104-111.
Sharshar T, Hopkinson NS, Ross ET, et al. Motor control of the costal and crural diaphragm – insights from transcranial magnetic stimulation in man. Respir Physiol Neurobiol. 2005;146:5-19.