CHAPTER 78 Development of the urogenital system
URINARY SYSTEM
Intermediate mesenchyme is disposed longitudinally in the trunk, subjacent to the somites (in the folded embryo), at the junction between the splanchnopleuric mesenchyme (adjacent to the gut medially) and the somatopleuric mesenchyme (subjacent to the ectoderm laterally) (Fig. 78.1). In lower vertebrates, intermediate mesenchyme typically develops serial, segmental epithelial diverticuli termed nephrotomes. Each nephrotome encloses a cavity, the nephrocele, which communicates with the coelom through a peritoneal funnel, the nephrostome (Fig. 78.2). The dorsal wall of a nephrotome evaginates as a nephric tubule. The dorsal tips of the cranial nephric tubules bend caudally and fuse to form a longitudinal primary excretory duct, which grows caudally and curves ventrally to open into the cloaca. The more caudally placed, and therefore chronologically later, tubules open secondarily into this duct or into tubular outgrowths from it. Glomeruli, specific arrangements of capillaries and overlying coelomic epithelium, arise from the ventral wall of the nephrocele (internal glomeruli) or the roof of the coelom adjacent to the peritoneal funnels (coelomic or external glomeruli), or in both situations (Fig. 78.2).
It has been customary to regard the renal excretory system as three organs, the pronephros, mesonephros and metanephros, succeeding each other in time and space, such that the last to develop is retained as the permanent kidney (Figs 78.1, 78.2). However, it is difficult to provide reliable criteria by which to distinguish these stages or to define their precise limits in embryos.
Pronephros
The intermediate mesenchyme becomes visible in stage 10 embryos and can be distinguished as a nephrogenic cord when 10 somites are present. A pronephros is present in human embryos only as clusters of cells in the most cranial portions of the nephrogenic cord (Figs 78.1, 78.2). More caudally, similar groups of cells appear and become vesicular. The dorsal ends of the most caudal of the vesicles join the primary excretory duct. Their central ends are connected with the coelomic epithelium by cellular strands, which probably represent rudimentary peritoneal funnels. Glomeruli do not develop in association with these cranially situated nephric tubules, which ultimately disappear. It is doubtful whether external glomeruli develop in human embryos.
Primary excretory duct
In stage 11 embryos of approximately 14 somites, the primary excretory duct can be seen as a solid rod of cells in the dorsal part of the nephrogenic cord. Its cranial end is about the level of the ninth somite and its caudal tip merges with the undifferentiated mesenchyme of the cord. It differentiates before any nephric tubules, and when the latter appear it is at first unconnected with them. In older embryos the duct has lengthened and its caudal end becomes detached from the nephrogenic cord to lie immediately beneath the ectoderm. From this level it grows caudally, independent of the nephrogenic mesenchyme, and then curves ventrally to reach the wall of the cloaca. It becomes canalized progressively from its caudal end to form a true duct, which opens into the cloaca in embryos at stage 12 (Fig. 78.1C). Clearly, up to this stage the name ‘duct’ is scarcely appropriate.
Mesonephros
From stage 12 mesonephric tubules, which develop from the intermediate mesenchyme between somite levels 8–20, begin to connect to the primary excretory duct, which is now renamed the mesonephric duct. More caudally, a continuous ridge of nephrogenic mesenchyme extends to the level of somite 24. The mesonephric tubules (nephrons) are not metameric – there may be two or more mesonephric tubules opposite each somite.
By the end of the sixth week each mesonephros is an elongated, spindle-shaped organ that projects into the coelomic cavity, one on each side of the dorsal mesentery, from the level of the septum transversum to the third lumbar segment. This whole projection is called the mesonephric ridge, mesonephros, or Wolffian body (Figs 78.1B,C, 78.3). It develops subregions, and a gonad develops on its medial surface (p. 1311). There are striking similarities in structure between the mesonephros and the permanent kidney or metanephros, but the mesonephric nephrons lack a segment that corresponds to the descending limb of the loop of Henle. The mesonephros is believed to produce urine by stage 17. A detailed comparison of the development and function of the mesonephros and metanephros in staged human embryos is not available.
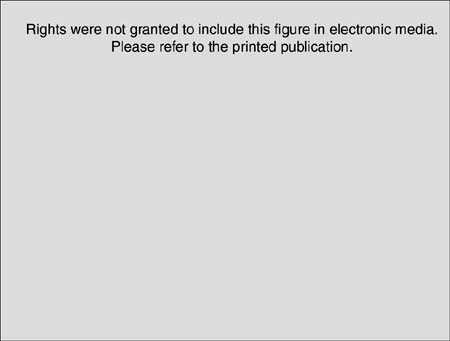
Fig. 78.3 Arrangement of mesonephric duct from mesonephros to urogenital sinus. The duct runs within the tubal fold with the paramesonephric duct. For later development, see Fig. 78.14.
(Redrawn with permission from Tuchmann-Duplessis H, Haegel P 1972 Illustrated Human Embryology, Vol 2 Organogenesis. London: Chapman and Hall. With kind permission of Springer Science+Business Media.)
In stage 18 embryos (13–17 mm) the mesonephric ridge extends cranially to about the level of rib 9. In both sexes the cranial end of the mesonephros atrophies, and in embryos 20 mm in length (stage 19) a mesonephros is found only in the first three lumbar segments, although it may still possess as many as 26 tubules. The most cranial one or two tubules persist as rostral aberrant ductules (see Fig. 78.13); the succeeding five or six tubules develop into either the efferent ductules of the testis and lobules of the head of the epididymis (male), or the tubules of the epoöphoron (female); the caudal tubules form the caudal aberrant ductules and the paradidymis (male), or the paroöphoron (female) (p. 1313).
Mesonephric duct
Once mesonephric nephrons connect to the primary excretory duct it is renamed the mesonephric duct. This runs caudally in the lateral part of the nephric ridge, and at the caudal end of the ridge it projects into the cavity of the coelom in the substance of a mesonephric fold (Fig. 78.3). As the mesonephric ducts from each side approach the urogenital sinus the two mesonephric folds fuse, between the bladder ventrally and the rectum dorsally, forming a transverse partition across the cavity of the pelvis, which is somewhat inappropriately called the genital cord (Fig. 78.3). In the male the peritoneal fossa between the bladder and the genital cord becomes obliterated, but it persists in the female as the uterovesical pouch. In the male the mesonephric duct itself becomes the canal of the epididymis, vas deferens and ejaculatory duct (p. 1317).
Urogenital sinus
The primitive hindgut ends in a cloacal region. This is connected ventrally with a blind-ending diverticulum, the allantois, which is intimately related to the development of the caudal portion of the urinary system. The enteric and allantoic portions of the hindgut are separated by the proliferation of the urorectal septum, a partition of mesenchyme and endoderm in the angle of the junction of hindgut and allantois (Fig. 78.4; see Fig. 78.7). The endodermal epithelium beneath the mesenchyme of the urorectal septum approaches but does not fuse with the cloacal membrane: it effectively divides the membrane into anal (dorsal) and urogenital (ventral) membranes, and the cloacal region into dorsal and ventral portions. The dorsal portion of the cloacal region is the putative rectum. The ventral portion can be further divided into: a cranial vesicourethral canal, continuous above with the allantoic duct; a middle, narrow channel, the pelvic portion; and a caudal, deep, phallic section, which is closed externally by the urogenital membrane. The second and third parts together constitute the urogenital sinus.
Metanephros
The metanephric kidney develops from three sources. An evagination of the mesonephric duct, the ureteric bud, and a local condensation of mesenchyme, the metanephric blastema, form the nephric structure (Fig. 78.5). Angiogenic mesenchyme migrates into the metanephric blastema slightly later to produce the glomeruli and vasa recta. It is possible that an intact nerve supply is also required for metanephric kidney induction.
An epithelial/mesenchymal interaction between the duct system and the surrounding mesenchyme occurs in both mesonephric and metanephric systems. In the mesonephric kidney, development proceeds in a craniocaudal progression, and cranial nephrons degenerate before caudal ones are produced. In the metanephric kidney a proportion of the mesenchyme remains as stem cells that continue to divide and which enter the nephrogenic pathway later when the individual collecting ducts lengthen. The temporal development of the metanephric kidney is patterned radially, such that the outer cortex is the last part to be formed. The following interactions occur in the development of the metanephric kidney (Fig. 78.5). The ureteric bud undergoes a series of bifurcations within the surrounding metanephric mesenchyme, and forms smaller ureteric ducts. At the same time the metanephric mesenchyme condenses around the dividing ducts to form S-shaped clusters, which transform into epithelia and fuse with the ureteric ducts at their distal ends. Blood vessels invade the proximal ends of the S-shaped clusters to form vascularized glomeruli.
The ureteric bud bifurcates when it comes into contact with the metanephric blastema in response to extracellular matrix molecules synthesized by the mesenchyme. Both chondroitin sulphate proteoglycan synthesis and chondroitin sulphate glycosaminoglycan processing are necessary for the dichotomous branching of the ureteric bud. In metanephric culture, incubation of fetal kidneys in β-D-xyloside, an inhibitor of chrondroitin sulphate synthesis, dramatically inhibits ureteric bud branching.
Subsequent divisions of the ureteric bud and associated mesenchyme define the gross structure of the kidney and the major and minor calyces, the distal branches of the ureteric ducts that will form the collecting ducts of the kidney. The proximal position of the ureteric bud elongates to form the developing ureter (Fig. 78.6). As the collecting ducts elongate the metanephric mesenchyme condenses around them. An adhesion molecule, syndecan, can be detected between the mesenchymal cells in the condensate. The cells switch off expression of N-CAM, fibronectin and collagen I, and start to synthesize L-CAM (also called E cadherin) and the basal lamina constituents laminin and collagen IV. The mesenchymal clusters are thus converted to small groups of epithelial cells, which undergo complex morphogenetic changes. Each epithelial group elongates, and forms first a comma-shaped, then an S-shaped, body, which continues to elongate and subsequently fuses with a branch of the ureteric duct at its distal end, while expanding as a dilated sac at its proximal end (Fig. 78.5). The latter involutes, and cells differentiate locally such that the outer cells become the parietal glomerular cells, while the inner ones become visceral epithelial podocytes. The podocytes develop in close proximity to invading capillaries derived from angiogenic mesenchyme outside the nephrogenic mesenchyme. This third source of mesenchyme produces the endothelial and mesangial cells within the glomerulus. The (metanephric-derived) podocytes and the angiogenic mesenchyme produce fibronectin and other components of the glomerular basement membrane. The isoforms of type-IV collagen within this layer follow a specific programme of maturation as the filtration of macromolecules from the plasma becomes restricted.
Platelet derived growth factor (PDGF) β-chain and the PDGF receptor β-subunit (PDGFR β) have been detected in developing human glomeruli between 54 and 109 days’ gestation. PDGF β-chain is localized in the differentiating epithelium of the glomerular vesicle during its comma and S-shaped stages, while PDGFR β is expressed in the undifferentiated metanephric blastema, vascular structures and interstitial cells. Both PDGF β-chain and PDGFR β are expressed by mesangial cells, which may promote further mesangial cell proliferation.
All stages of nephron differentiation are present concurrently in the developing metanephric kidney (Fig. 78.5). Antigens for the brush border of the renal tubule appear when the S-shaped body has formed. They appear first in the inner cortical area.
The metanephric kidney is lobulated throughout fetal life, but this condition usually disappears during the first year after birth (see Fig. 78.8). Varying degrees of lobulation occasionally persist throughout life.
Endocrine development of the kidney
The kidney functions not only as an excretory organ, but also as an endocrine organ, secreting hormones that are concerned with renal haemodynamics. Before birth homeostasis is controlled by the placenta. The fetal kidney produces amniotic fluid. The kidneys of premature babies of less than 36 weeks are immature. They contain incompletely differentiated cortical nephrons, which compromise their ability to maintain homeostasis. Problems of immaturity are further compounded by the effects of hypoxia and asphyxia, which modify renal hormones.
Urinary bladder
The urinary bladder develops from the cranial vesicourethral canal, which is continuous above with the allantoic duct (Figs 78.4, 78.6, 78.7). The mesonephric ducts open into the urogenital sinus early in development. The ureters develop as branches of the mesonephric ducts, which attain their own access to the developing bladder, and their orifices open separately into the bladder on the lateral side of the opening of the mesonephric ducts. Later the two orifices become separated still further and, although the ureter retains its point of entry into the bladder, the mesonephric duct opens into that part of the urogenital sinus that subsequently becomes the prostatic urethra (Fig. 78.6). The triangular region of absorption of the mesonephric ducts contributes to the trigone of the bladder and dorsal wall of the proximal half of the prostatic urethra, i.e. as far as the opening of the prostatic utricle and ejaculatory ducts, or its female homologue, the whole female urethral dorsal wall. The remainder of the vesicourethral canal forms the body of the bladder and urethra, and its apex is prolonged to the umbilicus as a narrow canal, the urachus.
Neonatal urinary system
At birth the two kidneys weigh approximately 23 g. They function early in development and produce the amniotic fluid that surrounds the fetus. The lobulated appearance of fetal kidneys is still present at birth (Fig. 78.8; see Fig. 14.4). Addition of new cortical nephrons continues in the first few months of postnatal life after which general growth of the glomeruli and tubules results in the disappearance of lobulation. The renal blood flow is lower in the neonate; adult values are attained by the end of the first year. The glomerular filtration rate at birth is approximately 30% of the adult value, which is attained by 3–5 months of age.
The neonatal urinary bladder is egg-shaped and the larger end is directed downwards and backwards (Figs 78.9, 78.10; see Figs 14.4, 14.5). Although described as an abdominal organ, nearly one half of the neonatal bladder lies below a line drawn from the promontory of the sacrum to the upper edge of the pubic symphysis, i.e. within the cavity of the true pelvis. From the bladder neck, the bladder extends anteriorly and slightly upwards in close contact with the pubis until it reaches the anterior abdominal wall. The apex of the contracted bladder lies at a point midway between the pubis and the umbilicus. When the bladder is filled with urine the apex may extend up to the level of the umbilicus. It is therefore possible to obtain urine by inserting a needle, connected to a syringe, into the bladder through the abdominal wall about 2 cm above the symphysis pubis and aspirating the contents into the sterile syringe. The success rate of the procedure is variable and depends upon the bladder being full: a much higher success rate has been reported by using an ultrasound scanner to locate the bladder and confirm that it contains urine prior to the insertion of the needle.
There is no true fundus in the fetal bladder as there is in the adult. Although the anterior surface is not covered with peritoneum, peritoneum extends posteriorly as low as the level of the urethral orifice. Because the apex of the bladder is relatively high, pressure on the lower abdominal wall will express urine from an infant bladder. Moreover, because the bladder remains connected to the umbilicus by the obliterated remains of the urachus (see Fig. 14.5), stimulation of the umbilicus can initiate micturition in babies. The elongated shape of the bladder in neonates means that the ureters are correspondingly reduced in length and they lack a pelvic portion. The bladder does not gain its adult, pelvic, position until about the sixth year. A distinct interureteric fold is present in the contracted neonatal bladder.
Anomalies of the urinary system
It was thought that renal cysts arose from clumps of vesicular cells, which persisted when the tips of branches from the ureteric diverticulum failed to fuse with metanephrogenic cap tissue. It is now believed that they are wide dilatations of a part of otherwise continuous nephrons. In most cases, autosomal dominant polycystic kidney disease results from mutations of PKD1 or PKD2 genes which are expressed in human embryos from 5–6 weeks of development within the mesonephros and later the metanephros (Chauvet et al 2002). In this condition the cystic dilatation may affect any part of the nephron, from Bowman’s capsule to collecting tubules. Less common is infantile cystic renal disease, inherited as a recessive trait, where the proximal and distal tubules are dilated to some degree but the collecting ducts are grossly affected.
Abnormalities of the ventral body wall caudal to the umbilicus, especially with inappropriate siting of the genital tubercle can result in exstrophy of the bladder (Fig. 78.11). In this condition the urorectal septum (internal) is associated with the genital tubercle (external), which means that the urogenital and anal membranes are widely separated. When the urogenital membrane involutes, the posterior surface of the bladder is exposed to the anterior abdominal wall. The lower part of the abdominal wall is therefore occupied by an irregularly oval area, covered with mucous membrane, on which the two ureters open. The periphery of this extroverted area, which is covered by urothelium, becomes continuous with the skin.