CHAPTER 73 Development of the peritoneal cavity, gastrointestinal tract and its adnexae
POSTPHARYNGEAL FOREGUT
The primitive gut is divided by head and tail folding into three main compartments. The foregut extends from the buccopharyngeal membrane to its continuation into the central midgut region via the cranial intestinal portal. The midgut extends between the intestinal portals, and in the early embryo is in wide communication with the yolk sac. The hindgut extends from the caudal intestinal portal to the cloacal membrane. The cranial end of the foregut, the embryonic pharynx, is intimately associated with head and neck development (see Ch. 35). The portion of foregut that passes dorsal to the pericardial cavity gives rise to the respiratory diverticulum and oesophagus within the thorax (see Chs 35 and 59). Caudal to the developing diaphragm, the enteric gut is conventionally subdivided into three embryological portions, fore- mid- and hindgut. There are no corresponding fundamental morphological and cytological distinctions between the three parts (Fig. 73.1), and so the foregut produces a portion of the duodenum, as does the midgut, and the midgut similarly produces large intestine, as does the hindgut. The differences between the portions of the gut develop as a result of interactions between the three embryonic tissue layers which give rise to the gut, namely the endodermal inner epithelium, the thick layer of splanchnopleuric mesenchyme, and the outer layer of proliferating splanchnopleuric coelomic epithelium.
Figure 73.2 shows the gut in a stage 12 embryo in relation to the other developing viscera, especially the heart and liver. Figure 73.3 shows the overall development of the gut from stages 13–17. These diagrams should be compared. Figure 73.1 shows the fundamental relationship of the intraembryonic coelom to the developing gut.
All regions of the gut develop from epithelial/mesenchymal interactions which are dependent on the sequential expression of a range of basic and specific genes; on the regulation of the developmental clock, seen in all areas of development; on endogenous regulatory mechanisms and local environmental influences (Lebenthal 1989). Although all these factors pertain to the whole range of developing tissues, local differences in any one of these factors along the length of the developing gut promotes the differentiation of, for example, the gastric mucosa and hepatocytes; the rotation of the midgut; and the final disposition of the sessile portions of the fully formed gastrointestinal tract. The gut is functional prior to birth and able to interact with the extrauterine environment in preterm infants.
OESOPHAGUS
The oesophagus can be distinguished from the stomach at stage 13 (embryo 5 mm). It elongates during successive stages and its absolute length increases more rapidly than the embryo as a whole. Cranially it is invested by splanchnopleuric mesenchyme posterior to the developing trachea, and more caudally between the developing lungs and pericardioperitoneal canals posterior to the pericardium. (For details of tracheo-oesophageal fistulae see p. 1037). Caudal to the pericardium, the terminal, pregastric segment of the oesophagus has a short thick dorsal meso-oesophagus (from splanchnopleuric mesenchyme), while ventrally it is enclosed in the cranial stratum of the septum transversum mesenchyme. Each of the above are continuous caudally with their respective primitive dorsal and ventral mesogastria. Thus the oesophagus has only limited areas related to a primary coelomic epithelium. However, note the subsequent development of the para-oesophageal right and left pneumatoenteric recesses (see Fig. 73.7), the relation of the ventral aspect of the middle third of the oesophagus to the oblique sinus of the pericardium, and the relation of its lateral walls in the lower thorax to the mediastinal pleura. All the foregoing are secondary extensions from the primary coelom.
Oesophagus at birth
At birth the oesophagus extends 8–10 cm from the cricoid cartilage to the gastric cardiac orifice. It starts and ends one to two vertebrae higher than in the adult, extending from between the fourth to the sixth cervical vertebra to the level of the ninth thoracic vertebra (see Fig. 14.5). Its average diameter is 5 mm and it possesses the constrictions seen in the adult. The narrowest constriction is at its junction with the pharynx, where the inferior pharyngeal constrictor muscle functions to constrict the lumen: this region may be easily traumatized with instruments or catheters. In the neonate the mucosa may contain scattered areas of ciliated columnar epithelium, but these disappear soon after birth. Peristalsis along the oesophagus and at the lower oesophageal sphincter is immature at birth and results in frequent regurgitation of food in the newborn period. The pressure at the lower oesophageal sphincter approaches that of the adult at 3–6 weeks of age.
STOMACH
At the end of the fourth and beginning of the fifth week the stomach can be recognized as a fusiform dilation cranial to the wide opening of the midgut into the yolk sac (Figs 73.2 and 73.3). By the fifth week this opening has narrowed into a tubular vitelline intestinal duct, which soon loses its connection with the digestive tube. At this time the stomach is median in position and separated cranially from the pericardium by the septum transversum (see Fig. 73.5A), which extends caudally on to the cranial side of the vitelline intestinal duct and ventrally to the somatopleure. Dorsally, the stomach is related to the aorta and, reflecting the presence of the pleuroperitoneal canals on each side, is connected to the body wall by a short dorsal mesentery, the dorsal mesogastrium (see Fig. 73.6). The latter is directly continuous with the dorsal mesentery (mesenteron) of almost all of the remainder of the intestine, except its caudal short segment.
In human embryos of 10 mm (stage 15–16), the characteristic gastric curvatures are already recognizable. Growth is more active along the dorsal border of the viscus: its convexity markedly increases and the rudimentary fundus appears. Because of more rapid growth along the dorsal border, the pyloric end of the stomach turns ventrally and the concave lesser curvature becomes apparent (Fig. 73.3; see also Fig. 73.6). The stomach is now displaced to the left of the median plane and apparently becomes physically rotated, which means that its original right surface becomes dorsal and its left surface becomes ventral. Accordingly the right vagus is distributed mainly to the dorsal, and the left vagus mainly to the ventral, surfaces of the stomach. The dorsal mesogastrium increases in depth and becomes folded on itself. The ventral mesogastrium becomes more coronal than sagittal. The pancreaticoenteric recess (see Fig. 73.7B(ii)), hitherto usually described as a simple depression on the right side of the dorsal mesogastrium, becomes dorsal to the stomach and excavates downwards and to the left between the folded layers. It may now be termed the inferior recess of the bursa omentalis. Put simply, the stomach has undergone two ‘rotations’. The first is 90° clockwise, viewed from the cranial end, the second is 90° clockwise, about an anteroposterior axis. The displacement, morphological changes and apparent ‘rotation’ of the stomach have been attributed variously to its own and surrounding differential growth changes, extension of the pancreaticoenteric recess with changes in its mesenchymal walls, and pressure, particularly that exerted by the rapidly growing liver.
Muscularis
The stomach muscularis externa develops its circular layer at 8–9 weeks, when neural plexuses are developing in the body and fundus. The longitudinal muscle develops slightly later. The pyloric musculature is thicker than the rest of the stomach: in general, the thickness of the total musculature of the stomach at term is reduced compared to the adult.
Stomach at birth
The stomach exhibits fetal characteristics until just after birth when the initiation of pulmonary ventilation, the reflexes of coughing and swallowing, and crying, cause the ingestion of large amounts of air and liquid. Once postnatal swallowing has started the stomach distends to four or five times its contracted state, and shifts its position in relation to the state of expansion and contraction of the other abdominal viscera, and to the position of the body. In the neonate, the anterior surface of the stomach is generally covered by the left lobe of the liver, which extends nearly as far as the spleen (see Fig. 14.4). Only a small portion of the greater curvature of the stomach is visible anteriorly. The capacity of the stomach is 30–35 ml in the full-term neonate, rising to 75 ml in the second week and 100 ml by the fourth week (adult capacity is on average 1000 ml). The mucosa and submucosa are relatively thicker than in the adult, however, the muscularis is only moderately developed and peristalsis is not coordinated. At birth gastric acid secretion is low, which means that gastric pH is high for the first 12 postnatal hours. It falls rapidly with the onset of gastric acid secretion, usually after the first feed. Acid secretion usually remains low for the first 10 days postnatally. Gastric emptying and transit times are delayed in the neonate.
DUODENUM
The duodenum develops from the caudal part of the foregut and the cranial part of the midgut. A ventral mesoduodenum, which is continuous cranially with the ventral mesogastrium, is attached only to the foregut portion. Posteriorly the duodenum has a thick dorsal mesoduodenum which is continuous with the dorsal mesogastrium cranially and the dorsal mesentery of the midgut caudally. Anteriorly the extreme caudal edge of the ventral mesentery of the foregut extends onto the short initial segment of the duodenum. The liver arises as a diverticulum from the ventral surface of the duodenum at the foregut–midgut junction, i.e. where the midgut is continuous with the yolk sac wall (the cranial intestinal portal). The ventral pancreatic bud also arises from this diverticulum. The dorsal pancreatic bud evaginates posteriorly into the dorsal mesoduodenum slightly more cranially than the hepatic diverticulum. The rotation, differential growth, and cavitations related to the developing stomach and omenta cause corresponding movements in the duodenum, which forms a loop directed to the right, with its original right side now adjacent to the posterior abdominal wall (see Fig. 73.6). This shift is compounded by the migration of the bile duct and ventral pancreatic duct around the duodenal wall. Their origin shifts until it reaches the medial wall of the second part of the fully formed duodenum: the bile duct passes posteriorly to the duodenum and travels in the free edge of the ventral duodenum and ventral mesogastrium. Local adherence and subsequent absorption of part of the duodenal serosa and the parietal peritoneum results in almost the whole of the duodenum, other than a short initial segment, becoming retroperitoneal (sessile).
Duodenal atresia is a developmental defect found in 1 in 5000 live births (Whittle 1999). It may be associated with an annular pancreas which may compress the duodenum externally (20% of duodenal atresia), or with abnormalities of the bile duct. In 40–60% of cases the atresia is complete and pancreatic tissue fills the lumen. The condition can be diagnosed on ultrasound examination, which reveals a typical double bubble appearance caused by fluid enlarging the stomach and the proximal duodenum. Polyhydramnios is invariably present and often the indication for the scan. Duodenal atresia commonly occurs with other developmental defects, e.g. cardiac and skeletal anomalies and in Down’s syndrome.
DORSAL AND VENTRAL MESENTERIES OF THE FOREGUT
The epithelium of the stomach and duodenum does not rotate relative to its investing mesenchyme. The rotation includes the coelomic epithelial walls of the pericardioperitoneal canals, which are on each side of the stomach and duodenum and form its serosa, and the elongating dorsal mesogastrium or the much shorter dorsal mesoduodenum. A ventral mesogastrium can be seen when the distance between the stomach and liver increases. Whereas the dorsal mesogastrium takes origin from the posterior body wall in the midline, its connection to the greater curvature of the stomach, which lengthens as the stomach grows, becomes directed to the left as the stomach undergoes its first rotation. With the second rotation a portion of the dorsal mesogastrium now faces caudally (see Fig. 73.6). The ventral mesogastrium remains as a double layer of coelomic epithelium which encloses mesenchyme and forms the lesser omentum (see Fig. 73.7).
Movement of the stomach is associated with an extensive lengthening of the dorsal mesogastrium, which becomes the greater omentum, and which now, from its posterior origin, droops caudally over the small intestine, then folds back anteriorly and ascends to the greater curvature of the stomach. The greater omentum is therefore composed of a fold containing, technically, four layers of peritoneum. The dorsal mesoduodenum, or suspensory ligament of the duodenum, is a much thicker structure, and it fixes the position of the duodenum when the rest of the midgut and its dorsal mesentery elongate and pass into the umbilical cord. For a more detailed account of this process see page 1216.
SPECIAL GLANDS OF THE POSTPHARYNGEAL FOREGUT
Pancreas
The pancreas develops from two evaginations of the foregut which fuse to form a single organ. A dorsal pancreatic bud can be seen in stage 13 embryos as a thickening of the endodermal tube which proliferates into the dorsal mesogastrium (Figs 73.3 and 73.4). A ventral pancreatic bud evaginates in close proximity to the liver primordium but cannot be clearly identified until stage 14, when it appears as an evagination of the bile duct itself. At stage 16 (5 weeks) differential growth of the wall of the duodenum results in movement of the ventral pancreatic bud and the bile duct to the right side and ultimately to a dorsal position. It is not clear whether there is a corresponding shift of mesenchyme during this rotation. However, the ventral pancreatic bud and the bile duct rotate from a position within the ventral mesogastrium (ventral mesoduodenum) to one in the dorsal mesogastrium (dorsal mesoduodenum) which is destined to become fixed onto the posterior abdominal wall. By stage 17 the ventral and dorsal pancreatic buds have fused, although the origin of the ventral bud from the bile duct is still obvious. Three-dimensional reconstruction of the ventral and dorsal pancreatic buds have confirmed that the dorsal pancreatic bud forms the anterior part of the head, the body and the tail of the pancreas and the ventral pancreatic bud forms the posterior part of the head and the posterior part of the uncinate process. The ventral pancreatic bud does not form all of the uncinate process (Collins 2002).
The developing pancreatic ducts usually fuse in such a way that most of the dorsal duct drains into the proximal part of the ventral duct (Figs 73.3 and 73.4). The proximal portion of the dorsal duct usually persists as an accessory duct. The fusion of the ducts takes place late in development or in the postnatal period: 85% of infants have patent accessory ducts as compared to 40% of adults. Fusion may not occur in 10% of individuals, in which case separate drainage into the duodenum is maintained, so-called pancreatic divisum. Failure of the ventral pancreatic diverticulum to migrate will result in an annular pancreas which may constrict the duodenum locally.
The ventral pancreas does not always extend anterior to the superior mesenteric vein but remains related to its right lateral surface. Initially the body of the pancreas extends into the dorsal mesoduodenum and then cranially into the dorsal mesogastrium. As the stomach rotates, this portion of the dorsal mesogastrium is directed to the left forming the posterior wall of the lesser sac. The posterior layer of this portion of dorsal mesogastrium fuses with the parietal layer of the coelom wall (peritoneum) and the pancreas becomes mainly retroperitoneal (see Fig. 73.7C). The region of fusion of the dorsal mesogastrium does not extend so far left as to include the tail of the pancreas which passes into the lienorenal (splenorenal) ligament. The anterior border of the pancreas later provides the main line of attachment for the posterior leaves of the greater omentum.
Cellular development of the pancreas
The early specification of pancreatic endoderm involves the proximity of the notochord to the dorsal endoderm, which locally represses the expression of Shh transcription factor. Endoderm caudal to the pancreatic region does not respond to notochordal signals. The ventral pancreatic endoderm does not seem to undergo the same induction. Pancreatic mesenchyme is derived from two regions. The mesenchyme which surrounds the dorsal pancreatic bud proliferates from the splanchnopleuric coelomic epithelium of the medial walls of the pericardioperitoneal canals, whereas the ventral pancreatic bud is invested by septum transversum mesenchyme and by mesenchyme derived from the lower ventral walls of the pericardioperitoneal canals.
The process of islet differentiation is divided into two phases (Collins 2002). Phase I, characterized by proliferation of polyhormonal cells, occurs from weeks 9–15. Phase II, characterized by differentiation of monohormonal cells, is seen from week 16 onwards. The β cells, producing insulin and amylin, differentiate first, followed by α-cells which produce glucagon. The δ cells which produce somatostatin are seen after 30 weeks. The dorsal bud gives rise mostly to α cells, and the ventral bud to most of the pancreatic polypeptide producing cells. The β cells develop from the duct epithelium throughout development and into the neonatal period. Later, in weeks 10–15, some of the primitive ducts differentiate into acinar cells in which zymogen granules or acinar cell markers can be detected at 12–16 weeks.
The pancreas in the neonate has all of the normal subdivisions of the adult. The head is proportionately large in the newborn and there is a smooth continuation between the body and the tail. The inferior border of the head of the pancreas is found at the level of the second lumbar vertebra. The body and tail pass cranially and to the left, and the tail is in contact with the spleen (see Fig. 14.4).
Liver
The liver is one of the most precocious embryonic organs and is the main centre for haemopoiesis in the fetus. It develops from an endodermal evagination of the foregut and from septum transversum mesenchyme, which is derived from the proliferating coelomic epithelium in the protocardiac region. The development of the liver is intimately related to the development of the heart. The vitelline veins, succeeded by the umbilical veins passing to the sinus venosus are disrupted by the enlarging septum transversum to form a hepatic plexus, the forerunner of the hepatic sinusoids. (See Collins, 2002 for a detailed account of hepatic development.)
Early liver development
In the stage 11 embryo the location of the hepatic endoderm has been identified at the superior boundary of the rostral intestinal portal. By stage 12, the hepatic endodermal primordium is directed ventrally and begins to proliferate as a diverticulum. There are two parts: a caudal part, which will produce the cystic duct and gallbladder, and a cranial part which forms the liver biliary system (Figs 73.3, 73.5A). The cells start to express liver-specific molecular markers and glycogen storage.
During this early phase of development the liver is far more highly vascularized than the rest of the gut. The hepatic capillary plexus is connected bilaterally with the right and left vitelline veins. Dorsolaterally they empty by multiple channels into enlarged hepatocardiac channels, which lead to the right and left horns of the sinus venosus (see Fig. 73.9); usually the channel on the right side is most developed. Both left and right channels bulge into the pericardioperitoneal canals, forming sites for the exchange of fluid from the coelom into the vascular channels. The growth of the hepatic tissue in these regions is sometimes referred to as the left and right horns of the liver.
Maturation of the liver
At 3 months’ gestation, the liver almost fills the abdominal cavity and its left lobe is nearly as large as its right. When the haematopoietic activity of the liver is assumed by the spleen and bone marrow, the left lobe undergoes some degeneration and becomes smaller than the right. The liver remains relatively larger than in the adult throughout the remainder of gestation. In the neonate it constitutes 4% of the body weight, compared to 2.5–3.5% in adults. It is in contact with the greater part of the diaphragm and extends below the costal margin anteriorly, and in some cases to within 1 cm of the iliac crest posteriorly. The left lobe covers much of the anterior surface of the stomach and constitutes nearly one-third of the liver (see Fig. 14.4). Although its haemopoietic functions cease before birth its enzymatic and synthetic functions are not completely mature at birth.
Development of intrahepatic biliary ducts
The development of the intrahepatic biliary ducts follows the branching pattern of the portal vein radicles (Collins 2002). The cranial hepatic diverticulum gives rise to the liver hepatocytes, the intrahepatic large bile ducts (right and left hepatic ducts, segmental ducts, area ducts and their first branches) and the small bile ducts (septal bile ducts, interlobular ducts and bile ductules). The portal and hepatic veins arise together from the vitelline veins. Early in development the accumulation of mesenchyme around these veins is similar, whereas later mesenchyme increases around the portal veins. This is a prerequisite for bile duct development. Primitive hepatocytes surround the portal vein branches and associated mesenchyme and form a sleeve of cells termed the ductal plate. Portions of the ductal plate divide to produce lines of epithelial cells which migrate close to a portal vein branch where they differentiate into bile ducts. As the bile ducts develop, angiogenic mesenchymal cells form blood vessels which connect to the hepatic artery from 10 weeks. Thus the portal triads are patterned by the portal vein radicles which initially induce bile duct formation and then artery formation. The development of the biliary system extends from the hilum to the periphery. Abnormalities of the biliary tree are associated with abnormalities of the branching pattern of the portal vein. The developing bile ducts remain patent throughout development; the solid stage of ductal development previously promulgated has been refuted. Atresia of the extrahepatic bile ducts has been noted, often in association with extrahepatic atresia. The cause of this condition is not clear; inflammatory process may be involved, although some cases have features of ductal plate malformation (Howard 2002).
Development of extrahepatic biliary ducts
Atresia of the extrahepatic bile ducts in neonates occurs alone or in conjunction with a range of other anomalies, including situs inversus, malrotation, polysplenia and cardiac defects. In such cases the intrahepatic bile ducts have a mature tubular shape but also show features of ductal plate malformation.
MIDGUT
The midgut forms the third and fourth parts of the duodenum, jejunum, ileum and two-thirds of the way along the transverse colon: its development produces most of the small and a portion of the large intestine. In embryos of stages 10 and 11 it extends from the cranial to the caudal intestinal portals and communicates directly with the yolk sac over its entire length. Although it has a dorsal wall, at these stages the lateral walls have not yet formed. By stage 12 the connection with the yolk sac has narrowed such that the midgut has ventral walls cranially and caudally. This connection is reduced to a yolk stalk containing the vitellointestinal duct during stage 13, at which time the yolk sac appears as a sphere in front of the embryo. Posterior to the midgut the splanchnopleuric coelomic epithelia converge forming the dorsal mesentery. Ventrolaterally the intraembryonic coelom is in wide communication with the extraembryonic coelom. At stage 14 the midgut has increased in length more than the axial length of the embryonic body and, with elongation of the dorsal mesentery, it bulges ventrally, deviating from the median plane. For all these stages see Figure 73.3.
PRIMARY INTESTINAL (OR MIDGUT) LOOP
The midgut loop can first be seen at stage 15 when a bulge, the caecal bud, can be discerned on the lower limb of the loop, caudal to the yolk stalk (which arises from the apex or summit of the loop) (Fig. 73.3). Later, the original proximal limb of the loop moves to the right and the distal limb to the left (Fig. 73.3C). The longest portion of the dorsal mesentery is at the level of the yolk stalk: there is less relative lengthening near the caudal end of the duodenum or the cranial half of the colon. The midgut extends into the umbilical coelom having already rotated through an angle of 90° (anticlockwise viewed from the ventral aspect). This relative position is approximately maintained so long as the protrusion persists, during which time the proximal limb which forms the small intestine elongates greatly. It becomes coiled, and its adjacent mesentery adopts a pleated appearance. The origin of the root of the mesentery is initially both median and vertical, while at its intestinal attachment it is elongated like a ruffle and folded along a horizontal zone. The mesenteric sheet and its contained vessels has spiralled through 90°. The distal, colic, part of the loop elongates less rapidly and has no tendency to become coiled. By the time the fetus has attained a length of 40 mm (10 weeks), the peritoneal cavity has enlarged and the relative size of the liver and mesonephros is much less. The re-entry of the gut occurs rapidly and in a particular sequence during which it continues the process of rotation. The proximal loop returns first, with the jejunum mainly on the left and the ileum mainly on the right of the subhepatic abdominal cavity. As they re-enter the abdominal cavity the coils of jejunum and ileum slide inwards over the right aspect of the descending mesocolon, and so displace the descending colon to the left. The transverse colon passes superiorly to the origin of the root of the mesentery (Fig. 73.6). The caecum is the last to re-enter and at first lies on coils of ileum on the right. Later development of the colon leads to its elongation and to the establishment of the hepatic and splenic flexures. A timetable for intestinal rotation in staged human embryos is given by Kim et al (2003).
Anomalies of midgut rotation
Gastroschisis is a para-umbilical defect of the anterior abdominal wall associated with evisceration of the abdominal organs. The organs are not enclosed in membranes, thus gastroschisis can be detected by prenatal ultrasonography and differentiated from exomphalos. Gastro-schisis is thought to result from periumbilical ischaemia caused by vascular compromise of either the umbilical vein or arteries. The incidence of this condition appears to be increasing, especially in babies born to young women less than 20 years old (Whittle 1999).
UMBILICAL CORD
During the period when the midgut loop protrudes into the umbilical coelom, the edges of the ventral body wall are becoming relatively closer, forming a more discrete root for the umbilical cord. Somatic mesenchyme, which will form the ventral body wall musculature, migrates into the somatopleuric mesenchyme and passes ventrally toward the midline. The umbilical cord (see p. 181) forms all of the ventral body wall between the pericardial bulge and the developing external genitalia. It encloses a portion of the extraembryonic coelom, the umbilical coelom, into which midgut loop protrudes. When the midgut loop is abruptly returned to the abdominal cavity the more recognizable umbilical cord forms. The vitellointestinal duct and vessels involute. The cranial end of the allantois becomes thinned and its lumen partially obliterated, and it forms the urachus. The mesenchymal core of the umbilical cord is derived by coalescence from somatopleuric amniotic mesenchyme, splanchnopleuric vitellointestinal (yolk sac) mesenchyme, and splanchnopleuric allantoic (connecting stalk) mesenchyme. These various layers become fused and are gradually transformed into the viscid, mucoid connective tissue (Wharton’s jelly) which characterizes the more mature cord. The changes in the circulatory system result in a large cranially oriented left umbilical vein (the right umbilical vein regresses), and two spirally disposed umbilical arteries (see p. 181).
MATURATION OF THE SMALL INTESTINE
Mucosa
The exact timing of the cellular morphogenesis of the gut is difficult to establish, especially as it undergoes a proximodistal gradient in maturation. Developmental differences between parts of the small intestine or colon have not yet been correlated with age. The endodermal cells of the small intestine proliferate and form a layer some three to four cells thick with mitotic figures throughout. From 7 weeks, blunt projections of the endoderm have begun to form in the duodenum and proximal jejunum; these are the developing villi which increase in length until in the duodenum the lumen becomes difficult to discern. The concept of occlusion of the lumen and recanalization which is described in many accounts of development does not match the cytodifferentiation which occurs in the gut epithelia. Thus it is no longer thought that there is secondary recanalization of the gut lumen. By 9 weeks the duodenum, jejunum and proximal ileum have villi and the remaining distal portion of ileum develops villi by 11 weeks. The villi are covered by a simple epithelium. Primitive crypts, epithelial downgrowths into the mesenchyme between the villi, appear between 10 and 12 weeks similarly along a craniocaudal progression. Brunner’s glands are present in the duodenum from 15 weeks and the muscularis mucosa can be seen in the small intestine from 18 weeks.
Meconium can be detected in the lumen of the intestine by the 16th week. It is derived from swallowed amniotic fluid, which contains vernix and cellular debris, salivary, biliary, pancreatic and intestinal secretions, and sloughed enterocytes. As the mixture passes along the gut, water and solutes are removed and cellular debris and proteins concentrated. Meconium contains enzymes from the pancreas and proximal intestine in higher concentrations in preterm than full-term babies.
Serosa
The small intestine possesses only a dorsal mesentery. The movement of the root of this dorsal mesentery, and the massive lengthening of its enteric border in order to match the longitudinal growth of the gut tube, reflect the spiralizing of the midgut loop in the umbilical coelom. The specific regions of adherence of the serosa and parietal peritoneum of the small intestine in the peritoneal cavity are given on page 1216.
Small intestine at birth
In the neonate the small intestine forms an oval-shaped mass with its greater diameter transversely orientated in the abdomen, rather than vertically as in the adult. The mass of the small intestine inferior to the umbilicus is compressed by the urinary bladder, which is anterior at this point. The small intestine is 300–350 cm long at birth and its width when empty is 1–1.5 cm. The ratio between the length of the small and large intestine at birth is similar to the adult ratio. The mucosa and submucosa are fairly well developed and villi are present throughout the small intestine, however, some epithelial differentiation is incomplete. The muscularis is very thin, particularly the longitudinal layer, and there is little elastic tissue in the wall. There are few or no circular folds in the small intestine, and the jejunum and ileum have little fat in their mesentery.
PRIMITIVE HINDGUT
Just as the foregut has an extensive, ventral endodermal diverticulum which contributes to a system separate from the gut, so too the hindgut has a ventral diverticulum, the allantois, destined for a different system. However, unlike the respiratory diverticulum of the foregut, the allantois is formed very early in development, prior even to formation of the embryonic endoderm and tail folding. With the reorganization of the caudal region of the embryo at stage 10, part of the allantois is drawn into the body cavity. The early embryonic hindgut thus consists of a dorsal tubular region extending from the caudal intestinal portal to the cloacal membrane, and a ventral blind-ending allantois extending from the cloacal region into the connecting stalk. The slightly dilated cavity, lined by endoderm, that cranially receives the enteric hindgut proper and the root of the allantoenteric diverticulum is termed the endodermal cloaca. It is closed ventrally by the cloacal membrane (endoderm opposed to proctodeal ectoderm), and it also has, transiently, a small recess of endoderm in the root of the tail, the postanal gut. As elsewhere, the hindgut, allantois and endodermal cloaca are encased in splanchnopleuric mesenchyme. Proliferation of the mesenchyme and endoderm in the angle of the junction of hindgut and allantois produces a urorectal septum (see Fig. 78.4B). Continued proliferation of the urorectal septum and elongation of the endodermal structures thrusts the endodermal epithelium towards the cloacal membrane with which it fuses centrally, separating the presumptive rectum and upper anal canal (dorsally) from the presumptive urinary bladder and urogenital sinus (ventrally) (see Fig. 78.4C). The cloacal membrane is thus divided into anal (dorsal) and urogenital (ventral) membranes. The nodal centre of division is the site of the future perineal body, the functional centre of the perineum. Details of the development of the allantoic hindgut are given on page 1322.
ENTERIC HINDGUT
The development of the large intestine, whether derived from mid- or hindgut, seems to be similar. The proximal end of the colon can be first identified at stage 15 when an enlargement of a local portion of gut on the caudal limb of the midgut loop defines the developing caecum. An evagination of the distal portion of the caecum forms the vermiform appendix at stage 17 (Fig. 73.3). Apart from the embryonic studies of Streeter (1942) there is little information about the development of the large intestine in humans. The early endodermal lining of the colon appears stratified, and mitoses occur throughout the layers. A series of longitudinal folds arise initially at the rectum and caecum and later in the regions of colon between these two points. The folds segment into villi with new villi forming between. The developing mucosa invaginates into the underlying mesenchyme between the villi to form glands which increase in number by splitting longitudinally from the base upwards. The villi gradually diminish in size and are absent by the time of birth.
MATURATION OF THE LARGE INTESTINE
Colon at birth
In the neonate the colon is typically 66 cm long and averages 1 cm in width. The caecum is relatively smaller than in the adult; it tapers into the vermiform appendix. The ascending colon is shorter in the neonate, reflecting the shorter lumbar region. The transverse colon is relatively long, whereas the descending colon is short, but twice the length of the ascending colon (see Fig. 14.4), The sigmoid colon may be as long as the transverse colon; it often touches the inferior part of the anterior body wall on the left and, in about 50% of neonates, part of the sigmoid colon lies in the right iliac fossa. The muscularis, including the taeniae coli, is poorly developed in the colon as it is in the small intestine. Appendices, epiploicae and haustra are not present, which gives a smooth external appearance to the colon. Haustra appear within the first 6 months. The rectum is relatively long; its junction with the anal canal forms at nearly a right angle.
ANAL CANAL
Mesenchymal proliferation occurs around the rim of the ectodermal aspect of the anal membrane which thus comes to lie at the bottom of a depression, the proctodeum (see Figs 78.4, 78.7E). With the absorption and disappearance of the anal membrane the anorectum communicates with the exterior. The lower part of the anal canal is formed from the proctodeal ectoderm and underlying mesenchyme, but its upper part is lined by endoderm. The line of union corresponds with the edges of the anal valves in the adult. The dual origin of the anal canal is reflected in its innervation: the endodermal portion is innervated by autonomic nerves, and the ectodermal proctodeum is innervated by spinal nerves.
In the fourth and fifth weeks a small part of the hindgut, the postanal gut, projects caudally beyond the anal membrane (Fig. 73.3B); it usually disappears before the end of the fifth week.
Imperforate anus is a term used to describe many different anorectal malformations. The most common is anal agenesis, which is found in almost 50% of all cases of imperforate anus. The condition is usually associated with a fistula, which opens into the vulva (females) or into the urethra (males). It is more rare for the anal membrane to fail to perforate. The condition cannot reliably be diagnosed prenatally by ultrasound diagnosis, and it may be confused with Hirschsprung’s disease (see below) and colonic atresia. The prognosis is good for low lesions of the anal canal. The principal concern in all cases is the degree of bowel control, urinary control and in some cases sexual function, which is compromised by the condition. Anorectal malformations may be indicators of other abnormalities, for example those forming the ‘VATER’ syndrome (Vertebral, Anal, Tracheo-Oesophageal and Renal abnormalities).
ENTERIC NERVOUS SYSTEM
Enteric neurones are derived from trunk neural crest cells at somite levels 1–7 and from 28 onwards (see Fig. 24.19 and p. 374). After neurulation the crest cells begin their ventral migration and invade the gut via the dorsal mesentery (see Fig. 24.11). Glial cells associated with the gut have been identified as arising from similar levels. The local splanchnopleuric mesenchyme patterns the crest cells such that those which enter the gut layers attain an enteric fate, whereas those that remain outside the gut become committed as parasympathetic postganglionic neurones. The enteric neurones also migrate to the glands of the gut, e.g. the pancreas.
Hirschsprung’s disease
Hirschsprung’s disease is usually characterized by an aganglionic portion of gut which does not display peristalsis, and a dilated segment of colon proximal to this site. Histologically there is either an absence or a reduction in the number of ganglia and postganglionic neurones in the myenteric plexus; postganglionic innervation of the muscle layers is also often defective. It is believed that the condition is caused by a failure of neural crest cells to colonize the gut wall appropriately. An overabundance of basal laminal components, perhaps at the mesothelial/mesenchyme interface, may prevent the early migrating neural crest cells from penetrating the gut wall; their new position outside the gut does not confer on them the environmental stimuli for enteric nerve differentiation and so non-enteric development occurs in local ganglia adjacent to the gut (Gershon 1987). A variable length of large intestine may be affected: the lower and midrectum are the most common sites, but in severe cases the rectum, sigmoid, descending and even proximal colon can be aganglionic. The chronic dilatation of the colon or rectum proximal to the affected segment gives rise to the common name, megacolon. It occurs as a consequence of functional obstruction due to the failure of peristalsis within the affected segment, and the dilated colon is structurally normal. Occasionally aganglionosis affects only a very short length of rectum proximal to the anorectal junction and the degree of functional obstruction is minimal: in these cases of ‘ultra-short segment Hirschsprung’s disease’, clinical abnormalities arise later in life. Infants with Hirschsprung’s disease show delay in the passage of meconium, constipation, vomiting and abdominal distension.
GUT-ASSOCIATED LYMPHOID TISSUE
There are similarly specialized epithelial cells between the enterocytes. Intraepithelial leukocytes typically account for 15% of the epithelial cells of the gut in the adult. They have been observed at 11 weeks, with a distribution of 2–3 intraepithelial leukocytes/100 gut epithelial cells. Both T and B lymphocytes have been described in the developing gut wall. For an account of the development of the immune cells of the gut consult Butzner & Befus (1989). The neonatal gut becomes colonized by a range of bacterial flora, some of which exist in a symbiotic relationship with their host, some of which may be considered pathogenic.
PERITONEAL CAVITY
The early development of the intraembryonic coelom which gives rise to the peritoneal cavity is described on pages 189 and 199. Figure 12.2 shows a scheme of the shape of the early peritoneal cavity and indicates the mesenchymal populations derived from its epithelial walls. Initially the peritoneal cavity associated with the lower end of the foregut has separate right and left components, the pleuroperioneal canals (Fig. 73.1). At the level of the midgut, the pleuroperitoneal canals join a confluent cavity surrounding the developing gut, which transitorily is in communication with the extraembryonic coelom.
PERITONEUM
Peritoneum develops from a specific portion of the intraembryonic coelomic walls (pp. 189, 199). Initially the intraembryonic coelomic epithelium is a pseudostratified germinal layer from which cellular progeny with different fates arise in specific sites and at specific developmental times. The portion which will give rise to the peritoneum is derived from the lower portion of the pericardioperitoneal canals and the somatopleure and splanchnopleure associated with the lower foregut, midgut and upper portions of the hind gut (see Figs 12.2, 73.1).
As the gut grows, splanchnic mesenchyme accumulates around the endodermal epithelium and the whole unit generally moves ventrally. There is a concomitant enlargement of the caudal ends of the developing pericardioperitoneal (pleuroperitoneal) canals and developing peritoneal cavity. The medial walls of the intraembryonic coelom move closer and there is a relative decrease in the mesenchyme between them. The regions where the medial portions of the intraembryonic coelom come together are termed mesenteries. They are composed of two layers of peritoneum with intervening mesenchyme and contain the neurovascular structures which pass to and from the gut. At the caudal ends of the pleuroperitoneal canals the gut has both ventral and dorsal mesenteries, whereas caudal to this there is only a dorsal mesentery (Figs 73.6, 73.7).
The mesenteries attached to the gut lengthen to permit large movements or rotations of the gut tube. Later, when part or the whole of the mesentery lies against the parietal peritoneum, their apposed surfaces fuse and are absorbed, i.e. they become sessile. Only those viscera developed in direct apposition to one of the primary coelomic regions, or a secondary extension of the latter, retain a partial or almost complete visceral serous cover. Thus the original line of reflexion of mesenteries becomes altered, or in some cases the organ may become retroperitoneal. These mechanisms are significant throughout the subdiaphragmatic gut, but are predominant in the small and large intestine. All serous membranes may vary their thickness, lines of reflexion, disposition, ‘space’ enclosed and their channels of communication, by a process of areal and thickness growth on one aspect combined with cavitation (leading to expanding embryonic recess formation) on the other (Figs 73.6, 73.7).
Mesenteries of the developing gut
The cervicothoracic oesophagus develops between the pericardioperitoneal canals (Fig. 73.1). It is encased in prevertebral, retrotracheal and retrocardiac mesenchyme. As the pericardioperitoneal canals expand with the developing lung buds, and the diaphragm forms immediately below them, the oesophagus at this level has no true dorsal or ventral mesentery. At superior and intermediate thoracic levels parts of the lateral aspects of the oesophagus are closely related to the secondary, mediastinal, parietal pleura. In the lower thorax the oesophagus inclines ventrally anterior to the descending thoracic aorta. The dorsocaudally sloping midline diaphragm between oesophageal and aortic orifices may be homologized with part of a dorsal meso-oesophagus, and is used in that context in descriptions of diaphragmatic development (see p. 1013). A ventral midline diaphragmatic strip may also be considered to be a derivative of a ventral meso-oesophagus, however, this region is more usually thought of as septum transversum.
The midgut and hindgut have no ventral mesentery; thus the pleural and supra-umbilical peritoneal cavities are initially, and transiently, bilaterally symmetrical above the umbilicus. Below the umbilicus, the peritoneal cavity is freely continuous across the midline ventral to the gut (Fig. 73.5A).
Foregut mesenteries
The ventral and dorsal foregut mesenteries are relatively large compared with the slender endodermal tubes they encase; they are composed of mesenchyme sandwiched between two layers of splanchnopleuric coelomic epithelium. A complex series of recesses develop in the splanchnopleuric mesenchyme and become confluent. As a result of foregut rotation, differential growth of stomach, liver, pancreas and spleen, and completion of the diaphragm, the territories of the greater sac and lesser sac (omental bursa) are delimited, and the mesenteric complexes of these organs (omenta and ‘ligaments’) are defined (Figs 73.6, 73.7).
Consequences of rotation of the stomach
A number of processes occur concurrently which conceptually can be visualized as the movement of the right pleuroperitoneal canal to a position posterior to the stomach such that its communication with the remainder of the peritoneal cavity is reduced. These processes include the differential growth of the walls of the stomach, the formation and specific local extension of the omenta (dorsal and ventral mesogastria), the growth of the liver and particularly of the vessels and ducts which enter and leave the liver. These developments permit stomach expansion both anteriorly and posteriorly when food is ingested and free movement of peristalsis. The right pleuroperitoneal canal forms a discrete region of the peritoneal cavity, the lesser sac, and the remaining left pleuroperitoneal canal and the remainder of the peritoneal cavity form the greater sac. The entrance to the original right pleuroperitoneal canal (lesser sac) becomes reduced in size. It is called the epiploic foramen, foramen of Winslow, or the aditus of the omental bursa (bursa omentalis) (Figs 73.6, 73.7).
Early stages of lesser sac development
The lesser sac is first indicated by the appearance of multiple clefts in the para-oesophageal mesenchyme on both left and right aspects of the oesophagus. Although they may become confluent, the left clefts are transitory and soon atrophy. The right clefts merge to form the right pneumatoenteric recess that extends from the oesophageal end of the lesser curvature of the stomach as far as the caudal aspect of the right lung bud. At its gastric end it communicates with the general peritoneal cavity and lies ventrolateral to the gut; more rostrally it lies directly lateral to the oesophagus. It is not, as commonly stated, a simple progressive excavation of the right side of the dorsal mesogastrium. The right pneumatoenteric recess undergoes further extension, subdivision and modification (Fig. 73.7 A(i), B(i)). From its caudal end a second process of cleft and cavity formation occurs which produces the hepatoenteric recess. This thins and expands the splanchnopleure between the liver and the stomach and proximal duodenum, and reaches the diaphragm (Fig. 73.7A(i), C(i)). The resulting, structurally bilaminar, mesenteric sheet is the lesser omentum. It is derived, cranially to caudally, from the small meso-oesophagus; the much larger ventral mesogastrium and the most caudal free border, is from the ventral mesoduodenum. As differential growth of the duodenum occurs, the biliary duct is repositioned and most of the duodenum becomes sessile. The duodenal attachment of the free border and a continuous neighbouring strip of the lesser omentum become confined to the upper border of a short segment of its superior part. The contrasting growth and positioning of its attached viscera cause the free border to change gradually from the horizontal to the vertical. It carries the bile duct, portal vein and hepatic artery, and its hepatic end is reflected around the porta hepatis. An alternative name for this part of the lesser omentum is the hepatoduodenal ligament: it forms the anterior wall of the epiploic foramen. The floor of the foramen is the initial segment of the superior part of the duodenum, its posterior wall is the peritoneum covering the immediately subhepatic part of the inferior vena cava, and its roof the peritonealized caudate process of the liver. The major part of the lesser omentum from the lesser gastric curvature passes in an approximately coronal plane to reach the floor of the increasingly deep groove for the ductus venosus on the hepatic dorsum: this part is sometimes called the hepatogastric ligament.
Ligaments of the liver
The medial portions of the germinative coelomic epithelial walls, containing splanchnopleuric mesenchyme, septum transversum mesenchyme and developing liver, constitute the early ventral mesogastrium (Fig. 73.5A). The mesenchyme between these layers is continuous superiorly with the septum transversum mesenchyme of the diaphragm. The coelomic epithelial layers of the ventral mesogastrium almost touch anterior and posterior to the liver, and are separated by a slender lamina of mesenchyme. They form the falciform ligament and the lesser omentum respectively, and where they are in contact with the liver directly they form visceral peritoneum (Fig. 73.7D).
Where the superior layers of the coronary and left triangular ligaments meet they continue as a (bilaminar) ventral mesentery attached to the ventrosuperior aspects of the liver. Its somewhat arched umbilicohepatic free caudal border carries the left umbilical vein. As the ventral body wall develops this falciform ligament, which initially attaches to the early cranial intestinal portal, is drawn to the diminishing cranial rim of the umbilicus. It may be considered the final ventral part of the ventral mesogastrium, although its free border has a ventral mesoduodenal origin. Its passage to the ventral body wall becomes increasingly oblique, curved and falciform (sickle-shaped) as the umbilicus becomes more defined.
In the early embryo the connection between one pericardioperitoneal canal and the other was directly across the ventral surface of the cranial midgut, immediately caudal to the developing primitive ventral mesogastrium. By stage 14 the passage from one side of the falciform ligament to the other necessitates passing below the greatly enlarged liver, or the curved lower edge of the falciform ligament, or the lesser omentum. The position of the falciform ligament is of clinical interest in the neonate in diagnosing pneumoperitoneum because it is silhouetted by air on abdominal X-rays.
Caval fold
Thus the caval fold is a zone where intestinal, mesenteric, intermediate, hepatic, pericardial, pulmonary and mediastinal mesenchymes meet and blend. It provides a mesenchymal route for the upper abdominal, transdiaphragmatic and transpericardial parts of the inferior vena cava, and it is also prominent in the development of parts of the liver, lesser sac of peritoneum, and certain mesenteries. The left fold regresses whereas the right fold enlarges rapidly (Fig. 73.7A).
Later stages of lesser sac development
The lower (inferior) part of the lesser sac can be first seen in embryos of 8–9 mm CR length and the early pneumatoenteric and hepatoenteric recesses are well established. Progressive differential gastric growth produces an elliptical transverse sectional profile, with a right-sided lesser curvature, which corresponds to the original ventral border of the gastric tube. The lesser omental gastric part of the ventral mesogastrium remains attached to this border. The greater curvature of the stomach is a new, rapidly expanding, region: its convex profile projects mainly to the left, but also cranially and caudally (Fig. 73.7 A(i), B(i), C(i)). The original dorsal border of the gastric tube now traverses the dorsal aspect of the expanding rudiment, curving along a line near the lesser curvature. The primitive dorsal mesogastrium is transiently attached to it, and blends with the thick layer of compound gastric mesenchyme clothing the posterior aspect and greater curvature of the miniature stomach. Because of its thickness, the mesenchyme projects cranially, caudally, and particularly to the left, beyond the ‘new’ greater curvature of the endodermal lining of the stomach.
The processes already described in relation to the ventral mesenteries now supervene. Multiple clefts appear at various loci in the mesenchyme, and there are local mesenchyme to epithelial transitions. The groups of clefts rapidly coalesce to form transiently isolated closed spaces which soon join with each other and with the preformed upper part of the lesser sac; the newly formed epithelia join the coelomic epithelium. In sequence, the initial loci occur in the compound posterior gastric mesenchyme nearer the lesser curvature and along its zone of blending with the primitive dorsal mesogastrium; in the dorsal mesoduodenum; and independently in the caudal rim, where greater curvature mesenchyme and dorsal mesogastrium blend. As these cavities become confluent and their ‘reniform’ expansion follows, matches and then exceeds that of the gastric greater curvature, there are several major sequelae. The primitive dorsal mesogastrium increases in area by intrinsic growth, and, as cavitation proceeds, by incorporating substantial contributions from the dorsal lamella separated by cleavage of the posterior gastric mesenchyme: it is now called the secondary dorsal mesogastrium (Fig. 73.7A(ii)). The gastric attachment of the secondary dorsal mesogastrium changes progressively. It may be regarded as a set of somewhat spiral lines, longitudinally disposed, that move with time to the left, from near the lesser curvature, towards and finally reaching, the definitive greater curvature. The parietal mesogastrial and (cleaving) mesoduodenal attachment remains in the dorsal midline for a time, but it later undergoes profound changes. With the confluence of the cavities that collectively form the lower part of the lesser sac, its communication with the upper part (which corresponds to the lesser gastric curvature and right and left gastropancreatic folds) becomes better defined. Ventral to the lower part of the cavity, postcleavage splanchnopleure covers the posteroinferior surface of the stomach and a short proximal segment of the duodenum. This ventral wall is continued beyond the greater curvature and duodenum as the splanchnopleuric strip of visceral attachment of the secondary dorsal mesogastrium and mesoduodenum. The radial width of the strip is relatively short cranially (gastric fundus) and gradually increases along the descending left part of the greater curvature. It is longest throughout the remaining perimeter of the greater curvature as far as the duodenum: this prominent part shows continued marginal (caudoventral and lateral) growth with extended internal cavitation (its walls constitute the expanding greater omentum; Fig. 73.6H; see Fig. 3.6E). The margins of the cavity of the inferior part of the lesser sac are limited by the reflexed edges of the ventrally placed strata derived from the secondary dorsal mesogastrium just described. These converge to form the splanchnopleuric dorsal wall, which is initially ‘free’ throughout except at its midline dorsal root. At roughly midgastric levels, the pancreatic rudiment grows obliquely encased in this dorsal wall; its tail ultimately reaches the left limit of the lesser sac at the level of the junction between gastric fundus and body (Fig 73.7C(ii)).
Greater omentum
The greater omentum continues to grow both laterally, and particularly caudoventrally. It covers and is closely applied to the transverse mesocolon, transverse colon and inframesocolic and infracolic coils of small intestine (Fig. 73.6D–G). At this stage the quadrilaminar nature of the dependent part of the greater omentum is most easily appreciated. ‘Simple’ mesenteries are bilaminar: they possess two mesothelial surfaces derived from splanchnopleuric coelomic epithelium, which enclose a connective tissue core derived from splanchnopleuric mesenchyme. In the greater omentum, the gastric serosa covering its posteroinferior surface (single mesothelium) and the anterosuperior serosa (single mesothelium) converge to meet at the greater curvature and initial segment of the duodenum. The resulting bilaminar mesentery continues caudoventrally as the ‘descending’ stratum of the omentum. This, on reaching the omental margins, is reflexed and now passes craniodorsally to its parietal root as the ‘ascending’ posterior bilaminar stratum. The two bilaminar strata are initially in fairly close contact caudally, but separated by a fine, fluid-containing, cleft-like extension of the lower part of the lesser sac. The posterior mesothelium of the posterior stratum makes equally close contact with the anterosuperior surface of the transverse colon, starting at the taenia omentalis, and with its transverse mesocolon.
Maturation of the lesser sac
At this stage, and subsequently, it is convenient to designate the lower part of the lesser sac as consisting of three subregions: retrogastric, perigastric and greater omental (Fig. 73.7C(ii)). The names are self-explanatory but their confines are all modified by various factors. Two phenomena are particularly prominent, namely, gastric ‘descent’ relative to the liver, and fusion of peritoneal layers with altered lines of reflexion, adhesion of surfaces and loss of parts of cavities.
After the third month, hepatic growth, particularly of the left lobe, diminishes and the whole organ recedes into the upper abdomen. Meanwhile the stomach elongates and some descent occurs, despite its relatively fixed cranial and caudal ends. This produces the angular flexure of the stomach which persists postnatally. The concavity of the lesser curvature is now directed more precisely to the right, the lesser omentum is more exactly coronal and its free border vertical. Ventral to the liver the free border of the falciform ligament passes steeply craniodorsally from umbilicus to liver (see disposition in neonate in Figs 14.4 and 14.5). The mesenchymal dorsal wall of the lower part of the lesser sac, which is crossed obliquely by the growing pancreas, has hitherto remained free and retained its original dorsal midline root. Substantial areas now fuse with adjacent peritonealized surfaces of retroperitoneal viscera, the parietes, or another mesenteric sheet or fold. Where sheets fuse there is a variable loss of apposed mesothelia and some continuity of their mesenchymal cores, but they remain surgically separable and no vascular anastomosis develops across the interzone. Above the pancreas the posterior secondary dorsomesogastrial wall of the sac becomes closely applied to the peritoneum covering the posterior abdominal wall and its sessile organs, the diaphragm, much of the left suprarenal gland, the ventromedial part of the upper pole of the left kidney, the initial part of the abdominal aorta, the coeliac trunk and its branches, and other vessels, nerves, and lymphatics (see Fig. 14.4). Their peritoneal surfaces fuse. However, albeit with some tissue loss, a single mesothelium remains covering these structures, intercalated as a new secondary dorsal wall for this part of the lesser sac.
The pancreas grows from the duodenal loop, penetrating the substance of the dorsal mesoduodenum and secondary dorsal mesogastrium; their mesenchymes and mesothelia initially clothe its whole surface, except where peritoneal lines of reflexion exist. Its posterior peritoneum becomes closely applied to that covering all the posterior abdominal wall structures it crosses (the inferior vena cava, abdominal aorta, splenic vein, superior mesenteric vessels, inferior mesenteric vein, portal vein, left renal vessels, the caudal pole of the left suprarenal, a broad ventral band on the left kidney and various muscles (see Figs 14.4, 14.5). The intervening peritoneal mesothelia fuse and atrophy, and the mesenchymal cores form fascial sheaths and septa. The pancreas is now sessile. The peritoneum covering the upper left part of its head, neck and the anterosuperior part of its body forms the central part of the dorsal wall of the lesser sac. The pancreatic tail remains peritonealized by a persisting part of the secondary dorsal mesogastrium as it curves from the ventral aspect of the left kidney towards the hilum of the spleen. The infracolic parts of the pancreas are covered with greater sac peritoneum. In the greater omental subregion of the lower part of the lesser sac two contrasting forms of mesenteric adhesion occur. The posterior ‘returning’ bilaminar stratum of the omentum undergoes partial fusion with the peritoneum of the transverse colon at the taenia omentalis and with its mesocolon. The layers remain surgically separable; no anastomosis occurs between omental and colic vessels.
The upper (oesophagophrenic) part of the lesser omentum arches across the diaphragm. As this bilaminar mesentery approaches the oesophageal hiatus its laminae diverge, skirting the margins of the hiatus. They then descend for a limited distance and with variable inclination, to enclose reciprocally shaped areas on the dorsum of the gastric fundus and diaphragm. The area may be roughly triangular to quadrangular; it contains areolar tissue and constitutes the bare area of the stomach or, when large, the left extraperitoneal space. Its right lower angle is the base of the left gastropancreatic fold, and its left lower angle reconstitutes the bilaminar mesentery. The root of the latter arches downwards and to the left across the diaphragm and suprarenal gland and gives the gastrophrenic ligament to the gastric fundus. It continues to arch across the ventral surface of the upper part of the left kidney, and its layers part to receive the pancreatic tail: they initially extend to the hilum of the spleen as the lienorenal (splenorenal) ligament (see Figs 73.6C, 73.7D),. The left half of this bilaminar ‘ligament’ provides an almost complete peritoneal tunic for the spleen. It then reunites with its fellow at the opposite rim of the splenic hilum, and continues to the next part of the gastric greater curvature as the gastrosplenic ligament. The remaining part (perhaps two-thirds) of the gastric greater curvature and its short duodenal extension provide attachment for the anterior, ‘descending’, bilaminar stratum of the greater omentum. Its returning, posterior, bilaminar stratum continues to its parietal root (which extends from the inferior limit assigned to the splenorenal ligament), and curves caudally and to the right along the anterior border of the body of the pancreas, immediately cranial to the line of attachment of the transverse mesocolon. Crossing the neck of the pancreas, the same curve is followed for a few centimetres on to its head; the omental root then sharply recurves cranially and to the left, to reach the inferior border of the duodenum. Thus it reaches that part of the lesser sac provided by cleavage of the dorsal mesoduodenum from the greater sac. It enters the epiploic foramen, traverses the epiploic canal between the caudate hepatic process and proximal duodenum, then crosses the right gastropancreatic fold, and descends behind the proximal duodenum to enter the right marginal strip enclosed by the greater omentum. The definitive origins of the peritoneum from the posterior abdominal wall are shown in the adult in Figures 64.1, 69.4 and 64.5B.
Small intestine
Most of the duodenal loop encircles the head of the pancreas and is retroperitoneal. The peritoneum principally covers its ventral and convex aspects. Areas not covered are a short initial segment of the superior (first) part, which is more completely peritonealized because it gives attachment to the right margins of the greater and lesser omenta; the sites where the transverse colon is closely apposed to the descending (second) part, or where the latter is crossed by the root of the transverse mesocolon; and the sites where the mesentery crosses the transverse (third) part, and descends across the ascending (fourth) part from its upper extremity at the duodenojejunal flexure. These regions are illustrated in the adult in Figure 64.1. In addition, one or more of up to six different duodenal recesses may develop. Their variations in shape and size, their intestinal, mesenteric and vascular relations, and, when adequately recorded, the frequencies and disposition of their orifices, are given on page 1108–1109.
From a mesenteric standpoint, the succeeding small intestine, from the duodenojejunal flexure to the ileocaecal junction, undergoes less modification of its embryonic form than other gut regions. Its early dorsal mesentery is a continuous, single (but structurally bilaminar) sheet, with a midline parietal attachment (line of reflexion, or ‘root’). The attachment of the root becomes an oblique narrow band from the left aspect of the second lumbar vertebra to the cranial aspect of the right sacroiliac joint (Fig. 73.6H; see Fig. 64.1).
Transverse colon
The right extremity of the transverse colon is sessile, and is separated by fibroareolar tissue from the anterior aspect of the descending (second) part of the duodenum and the corresponding aspect of most of the head of the pancreas. The remainder of the transverse colon, up to and including the left (splenic) colic flexure, is almost completely peritonealized by the diverging layers of the transverse mesocolon. The root of the latter reaches the neck and whole extent of the anterior border of the body of the pancreas. The long axis of the definitive pancreas lies obliquely. The splenic colonic flexure is considerably more rostral than the hepatic flexure and consequently the root of the mesocolon curves obliquely upwards as it crosses the upper abdomen from right to left. As it expands, the posteroinferior wall of the greater omental part of the lesser sac gradually covers, and becomes closely applied to, the transverse mesocolon and its contained colon, finally projecting beyond the latter. Craniocaudal adherence now occurs between the omental wall and the pericolonic and mesocolonic layers.
Rectum
The rectum continues from the ventral aspect of the third sacral vertebra to its anorectal (perineal) flexure anteroinferior to the tip of the coccyx: the distance changes with age. All aspects of the rectum are encased by mesenchyme, and the early dorsally placed mass is named, by some authorities, the dorsal mesorectum. However, the latter does not form a true mesentery: with progressive skeletal development it is reduced to a woven fibroareolar sheet which displays patterned variations in thickness and fibre orientation. The sheet is closely applied to the ventral concavity of the sacrum and coccyx, and encloses numerous fibromuscular and neurovascular elements. The rectum therefore becomes sessile, and visceral peritoneum is restricted to its lateral and ventral surfaces (see Fig. 78.4).
With the disappearance of the postanal gut by the end of the fifth week, the ventrolateral peritoneum reaches the superior surface of the pelvic floor musculature: this condition persists until late in the fourth month. In the male the ventral rectal peritoneum is reflected over the posterior surface of the prostate, bladder trigone and associated structures. In the female the ventral peritoneum initially receives a reflection which covers almost the whole posterior aspect of the vagina, and is continued over the uterus. Subsequently, the closely apposed walls of these deep peritoneal pouches fuse over much of their caudal extent, their mesothelia are lost, and the viscera are separated by an intervening, bilaminar (surgically separable), fibrous stratum. In the male this becomes the rectovesical fascia and posterior wall of the prostatic sheath (see Fig. 78.10). In the female it becomes the rectovaginal septum between the lower part of the vagina and the rectum (see Fig. 78.9). The proximal third of the rectum is covered by peritoneum ventrolaterally: the lateral extensions of this tunic are triangular and deep proximally, but taper to an acute angle by the middle third of the rectum. The middle third of the rectum is covered by peritoneum only on its ventral surface, where it forms the posterior wall of the shallower rectovesical or rectovagino-uterine pouch. The remaining rectum and anal canal are extraperitoneal.
NEONATAL PERITONEAL CAVITY
The fully formed peritoneal cavity, although complex topographically, remains a single cavity with numerous intercommunicating regions, pouches and recesses (see Fig. 14.5). The only small peritoneal sacs to separate completely from the main cavity are the infracardiac bursa (Fig. 73.7(B(i)) and the tunica vaginalis testis (see Fig. 78.17).
In the neonate the peritoneal cavity is ovoid (see Fig. 14.4). It is fairly shallow from anterior to posterior because the bilateral posterior extensions on each side of the vertebral column, which are prominent in the adult, are not present. Two factors lead to the protuberance of the anterior abdominal wall in the neonate and infant. The diaphragm is flatter in the newborn, which produces a caudal displacement of the viscera. The pelvic cavity is very small in the neonate, which means that organs which are normally pelvic in the adult, i.e. urinary bladder, ovaries and uterus, all extend superiorly into the abdomen (see Figs 14.5, 78.9, 78.10). The pelvic cavity is joined to the abdominal cavity at less of an acute angle in the neonate because there is no lumbar vertebral curve and only a slight sacral curve.
The peritoneal attachments are similar to the adult. However, the greater omentum is relatively small: its constituent layers of peritoneum may not be completely fused, and it does not extend much below the level of the umbilicus (see Fig. 14.5). Generally the length of the mesentery of the small intestine and of the transverse and sigmoid mesocolons are longer than in the adult, whereas the area of attachment of the ascending and descending colons is relatively smaller. The peritoneal mesenteries and omenta contain little fat.
SPLEEN
The spleen appears about the sixth week as a localized thickening of the coelomic epithelium of the dorsal mesogastrium near its cranial end (Figs 73.6, 73.7). The proliferating cells invade the underlying angiogenetic mesenchyme, which becomes condensed and vascularized. The process occurs simultaneously in several adjoining areas which soon fuse to form a lobulated spleen of dual origin (from coelomic epithelium and from mesenchyme of the dorsal mesogastrium). The enlarging spleen projects to the left, so that its surfaces are covered by the peritoneum of the mesogastrium on its left aspect, which forms a boundary of the general extrabursal (greater) sac. When fusion occurs between the dorsal wall of the lesser sac and the dorsal parietal peritoneum, it does not extend to the left as far as the spleen, which remains connected to the dorsal abdominal wall by a short lienorenal ligament. Its original connection with the stomach persists as the gastrosplenic ligament. The earlier lobulated character of the spleen disappears, but is indicated by the presence of notches on its upper border in the adult.
The spleen displays various developmental anomalies, including complete agenesis, multiple spleens or polysplenia, isolated small additional spleniculi and persistent lobulation. Asplenia and polysplenia are associated with other anomalies, especially those involving the cardiac and pulmonary systems. Accessory spleens are very common in neonates, located in the greater omentum. At birth the spleen weighs, on average, 13 g (see Fig. 14.4). It doubles its weight in the first postnatal year and triples it by the end of the third year.
SUPRARENAL GLANDS
The suprarenal (adrenal) cortex is formed during the second month by a proliferation of the coelomic epithelium. Cells pass into the underlying mesenchyme between the root of the dorsal mesogastrium and the mesonephros (see Fig. 24.11). The proliferating tissue, which extends from the level of the sixth to the 12th thoracic segments, is soon dis-organized dorsomedially by invasion of neural crest cells from somite levels 18–24, which form the medulla, and also by the development of venous sinusoids. The latter are joined by capillaries, which arise from adjacent mesonephric arteries and penetrate the cortex in a radial manner. When proliferation of the coelomic epithelium stops, the cortex is enveloped ventrally, and later dorsally, by a mesenchymal capsule which is derived from the mesonephros. The subcapsular nests of cortical cells are the rudiment of the zona glomerulosa: they proliferate cords of cells which pass deeply between the capillaries and sinusoids. The cells in these cords degenerate in an erratic fashion as they pass towards the medulla, becoming granular, eosinophilic and ultimately autolysed. These cords of cells constitute the fetal cortex, which undergoes rapid involution during the first two years after birth. The fascicular and reticular zones of the adult cortex are proliferated from the glomerular zone after birth.
The most common abnormality of suprarenal gland development is congenital hyperplasia, which occurs in 1 : 5000–1 : 15,000 births. This condition is caused by a group of autosomal recessive disorders in which there are deficiencies in enzymes required for the synthesis of cortisol. In 90% of cases the cause is deficiency of the enzyme 21-hydroxylase, producing an accumulation of 17-hydroxyprogesterone, which is converted to androgens. The levels of androgens increase by several hundred times, causing female embryos and fetuses to undergo external genital masculinization ranging from clitoral hypertrophy to formation of a phallus and scrotum: masculinization of the brain has also been suggested. In male embryos the levels do not cause any changes in external genitalia. Signs of androgen excess may appear in childhood with precocious masculinization and accelerated growth (Lewis, Yaron & Evans 1999).
SUPRARENAL GLANDS IN THE NEONATE
The suprarenal glands are relatively very large at birth (see Figs 14.4 and 78.8) and constitute 0.2% of the entire body weight, compared with 0.01% in the adult. The left gland is heavier and larger than the right, as it is in the adult. At term each gland usually weighs 4 g; the average weight of the two glands is 9 g (average in the adult is 7–12 g). The glands involute rapidly in the neonatal period when each gland loses 25% of its mass; the average weight of both glands is 5 g by the end of the second week, and 4 g by 3 months. Birth weight is not regained until puberty. The cortex of the suprarenal gland is thicker than in the adult and the medulla of the gland is small. Early studies on fetal suprarenal glands described extensive degeneration and necrosis of fetal zone cells; however, it is believed that these studies showed disease processes rather than the normal involution of the gland. With normal involution the fetal zone cells of the postnatal gland become smaller and they assume the appearance and organization typical of zona fasciculata.
INFERIOR VENA CAVA, PORTAL CIRCULATION AND UMBILICAL VESSELS
INFERIOR VENA CAVA
The inferior vena cava of the adult is a composite vessel which develops on the posterior abdominal wall dorsal to the developing peritoneal cavity. It forms as a consequence of the temporal remodelling of successive venous complexes (see Fig. 13.4). The precise mode of development of its postrenal segment (caudal to the renal vein) is still somewhat uncertain. Its function is initially carried out by the right and left postcardinal veins (Fig. 73.8; see also Fig. 13.4), which receive the venous drainage of the lower limb buds and pelvis and run in the dorsal part of the mesonephric ridges, receiving tributaries from the body wall (intersegmental veins) and from the derivatives of the mesonephroi.
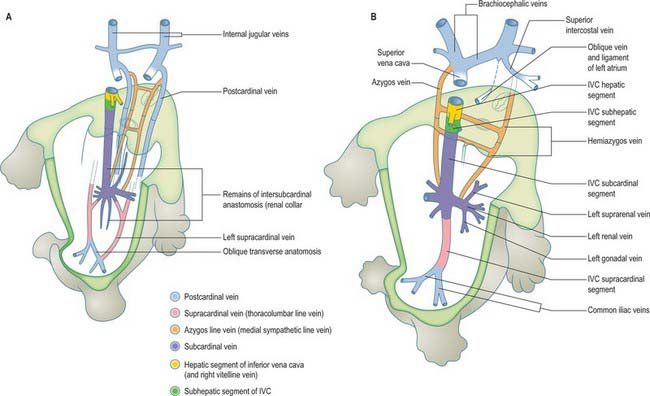
Fig. 73.8 The inferior vena cava develops within the posterior abdominal wall. It is a composite vessel formed by temporal remodelling of successive somatic venous anastomoses. (For early venous development see Fig. 13.4.)
The supracardinal veins receive the larger venous drainage of the growing body wall. The right supracardinal vein persists and forms the greater part of the postrenal segment of the inferior vena cava. The continuity of the vessel is maintained by the persistence of the anastomosis between the right supracardinal and the right subcardinal in the renal collar. The left supracardinal disappears, but some of the renal collar formed by the left supracardinal–subcardinal anastomosis persists in the left renal vein. Both supracardinal veins drain cranially into the subcardinal veins. On the right side, the subcardinal vein comes into intimate relationship with the liver. An extension of the vessel takes place in a cranial direction and meets and establishes continuity with a corresponding new formation that is growing caudally from the right vitelline hepatocardiac (common hepatic) vein. In this way, on the right side a more direct route is established to the heart and the prerenal (cranial) segment of the inferior vena. In summary, the inferior vena cava is formed from below upwards by the confluence of: the common iliac veins, a short segment of the right postcardinal vein, the postcardinal–supracardinal anastomosis, part of the right supracardinal vein, the right supracardinal–subcardinal anastomosis, right subcardinal vein, a new anastomotic channel of double origin (the hepatic segment of the inferior vena cava), and the cardiac termination of the right vitelline hepatocardiac vein (common hepatic vein).
PORTAL CIRCULATION
The development of the gut occurs contemporaneously with changes to the early symmetrical embryonic circulation (see Ch. 13). A symmetrical, segmental system of somatic arteries remains and supplies the body wall of the trunk. The underlying arrangement of these vessels is only slightly modified by subsequent development. The main changes to the embryonic arrangement occur with the growth of the foregut, liver and paired viscera, i.e. kidneys and suprarenal glands.
The early development of the ventral splanchnic arteries is outlined in Chapter 13. Three main arterial trunks, the coeliac trunk, superior mesenteric and inferior mesenteric arteries, supply the fore- mid- and hind gut respectively. From the gastric terminal segment of the future oesophagus to the upper rectum, the developing endodermal epithelium is surrounded by splanchnopleuric mesenchyme permeated by a capillary plexus which drains into an anastomosing network of veins. This plexus is particularly dense ventrally associated with the central midgut region where, for a while, it receives a network of small veins from the definitive yolk sac. Later, in normal development, these vessels atrophy as the yolk sac diminishes. More rostrally the splanchnopleuric capillaries associated with the foregut and upper portions of the yolk sac form longitudinal channels anterolateral to the gut and these become increasingly well defined as the embryonic abdominal vitelline veins. Entering the septum transversum, the right and left vitelline veins incline slightly, becoming parallel to the lateral aspects of the gut. They establish connections with capillary plexuses in the septal mesenchyme, then continue, finally curving to enter the medial part of the cardiac sinual horn of their corresponding side. The parts of the gut closely related to the presinual segments of the vitelline veins are the future subdiaphragmatic end of the oesophagus, primitive stomach, the superior (first) and descending (second) regions of the duodenum, and the remainder of the duodenal tube.
The principal ascending vitelline veins flanking the sides of the abdominal part of the foregut receive venules from its splanchnopleuric capillaries, and those of the septal mesenchyme. Within these venular nets, enlarged (but still plexiform) anastomoses connect the two vitelline veins (Fig. 73.9; see Fig. 13.1C). A subdiaphragmatic intervitelline anastomosis develops in the rostral septal mesenchyme, lying a little caudal to the cardiac sinuatrial chamber, connecting the veins near their sinual terminations. (The channel is sometimes termed suprahepatic because of the position of the hepatic primordium; with expansion of the latter it becomes partly intrahepatic.) The presumptive duodenum is crossed by three transverse duodenal intervitelline anastomoses. Their relation to the gut tube alternates so that the most cranial, the subhepatic, is ventral, the intermediate is dorsal and the caudal is ventral (Fig. 73.9B). It has become customary to describe the paraduodenal vitelline veins and their associated anastomoses as forming a figure 8. At this early stage when left and right embryonic veins are still symmetric, the cranial duodenal anastomosis becomes connected with the subdiaphragmatic anastomosis by a median longitudinal channel, the primitive median ductus venosus, which is dorsal to the expanding hepatic primordium, but ventral to the gut. The further development of the vitelline veins and anastomoses is, as indicated, closely interlocked, with rapid hepatic expansion and gut changes, and umbilical vein disposition and modification is closely involved.
CHANGES IN THE VITELLO-UMBILICAL VEINS
Progressive changes in the vitello-umbilical veins are rapid, profound and closely linked with regional modifications in shape and position of the gut, expansion and invasion of venous channels by hepatic tissue, asymmetry of the heart and cardiac venous return. The principal events are summarized in Figs 73.9, 73.10 and 73.11.
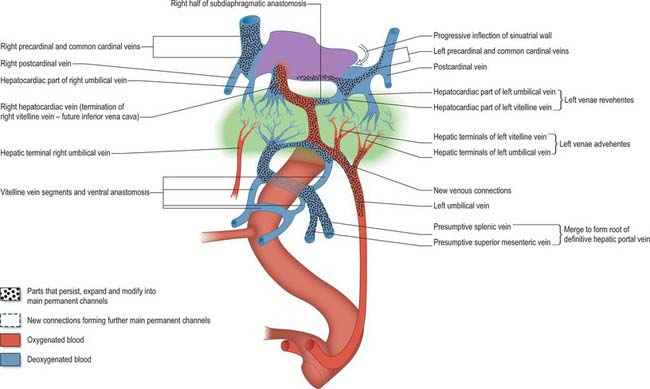
Fig. 73.10 Development of the vitelline, umbilical and terminal cardinal vein complexes: a mid-stage of asymmetry has been reached between the early symmetric condition (see Fig. 73.9) and the definitive late prenatal state. The extent of the liver within the septum transversum mesenchyme is shown in green.
From the cranial portion of the early hepatic evagination of the foregut, interconnected sheets and ‘cords’ of endodermal cells, the presumptive hepatocytes, penetrate the septum transversum mesenchyme. Hepatic mesenchyme is composed of a mixed population of cells with endothelial/angiogenic and connective tissue lineages. Capillary plexuses develop within this mesenchyme and, possibly under the influence of the endodermal hepatic sheets, the plexuses become more profuse by further addition of angioblastic septal mesenchyme, which also forms masses of perivascular intrahepatic haemopoietic tissue. These processes extend along the plexiform connections of the vitelline, and later the umbilical, veins until their intrahepatic (trans-septal) zones themselves become largely plexiform. Initially capillary in nature, they transform into a mass of rather wider, irregular, sinusoidal vessels with a discontinuous endothelium containing many phagocytic cells. The lengths of vitelline veins involved in these processes are the intermediate parts of the segments extending from the subhepatic (cranioventral duodenal) to the suprahepatic (subdiaphragmatic) transverse intervitelline anastomoses, and the corresponding lengths of the umbilical veins. Thus at this early stage the liver sinusoids are perfused by mixed blood reaching them through a series of branching vessels collectively called the venae advehentes, or afferent hepatic veins: they are, deoxygenated from the gut splanchnopleure via vitelline vein hepatic terminals and oxygenated from the placenta via hepatic terminals of the umbilical veins (Fig. 73.10). Blood leaves the liver through four venae revehentes (efferent hepatic veins); two on each side reach and open into their respective cardiac sinual horns. This full complement of four hepatocardiac veins is only transient, and becomes reduced to one dominant, rapidly enlarging channel. The originally bilaterally symmetric cardinal vein complexes, both rostral and caudal, develop transverse or oblique anastomoses so that the cardiac venous return is ultimately restricted to the definitive right atrium.
These cardiac and concomitant hepatoenteric changes are accompanied by events in supra-, intra- and subhepatic parts of the vitello-umbilical veins. Some vessels enlarge, persisting as definitive vessels to maturity and, in places, they are joined later by other channels that become defined in already established capillary plexuses. Other vessels retrogress, either disappearing completely or remaining as vestigial tags and, occasionally, vessels of fine calibre. Some vessels of crucial importance in the circulatory patterns of embryonic and fetal life become obliterated postnatally and transformed to substantial fibrous cords. Both right and left umbilical hepatocardiac and the left vitelline hepatocardiac veins continue, for a time, to discharge blood into their sinual horns; however, they begin to retrogress (Figs 73.9 and 73.10). The right umbilical channel atrophies completely. The left channels also disappear, but their cardiac terminals may, on occasion, be found as conical fibrous tags attached to the inferior wall of the coronary sinus. The right vitelline hepatocardiac vein continues enlarging and ultimately forms the terminal segment of the inferior vena cava. The latter receives the right efferent hepatic veins and new channels draining the territories of the left efferent hepatic veins. These collectively form the upper and lower groups of right and (secondary) left hepatic veins. The terminal caval segment also shows the orifice of the right half of the intervitelline subdiaphragmatic anastomosis, and a large new connection with the right subcardinal vein.
The hepatic terminals of the right and left duodenal parts of the vitelline veins are destined to form the corresponding branches of the hepatic portal vein, the left branch incorporating the cranial ventral intervitelline anastomosis. With rotation of the gut and formation of the duodenal loop, segments of the original vitelline veins and the caudal transverse anastomosis atrophy (indicated in Fig. 73.10 and 73.10), while new splanchnopleuric venous channels, the superior mesenteric and splenic veins, converge and join the left end of the dorsal intermediate anastomosis. The numerous other radicles of the portal vein and its principal branches, including the inferior mesenteric vein, are later formations.
For a period, placental blood returns from the umbilicus via right and left umbilical veins, both discharging through afferent hepatic veins into the hepatic sinusoids, where admixture with vitelline blood occurs. At approximately 7 mm crown–rump length, the right umbilical vein retrogresses completely. The left umbilical vein retains some vessels discharging directly into the sinusoids, but new enlarging connections with the left half of the subhepatic intervitelline anastomosis emerge. The latter is the start of a bypass channel for the majority of the placental blood, which continues through the median ductus venosus and finally the right half of the subdiaphragmatic anastomosis, to reach the termination of the inferior vena cava (Fig. 73.11).
FETAL/NEONATAL UMBILICAL VESSELS
Umbilical arteries
Umbilical arterial catheterization
Insertion of an umbilical catheter is undertaken to provide direct access to the arterial circulation. Arterial blood can be withdrawn repeatedly for measurement of oxygen and carbon dioxide partial pressures, pH, base excess and many other parameters of blood biochemistry and haematology. The indwelling catheter also enables the continuous measurement of arterial blood pressure.
The catheter is inserted directly into either the cut end or the side of one of the two umbilical arteries in the umbilical cord stump that remains attached to the baby after transection of the umbilical cord at the time of delivery. The catheter tip is then advanced along the length of the umbilical artery, through the internal iliac artery, into the common iliac artery and from there into the aorta. In order to keep the catheter patent, a small volume of fluid is continuously infused through it. It is important that the tip of the catheter should be located well away from arteries branching from the aorta, to avoid potentially harmful perfusion of these arteries with the catheter fluid. Thus umbilical arterial catheter tips are placed in the descending aorta either in a ‘high’ position, above the coeliac artery but well below the ductus arteriosus, or in a ‘low’ position, below the renal and inferior mesenteric arteries but above the point where the aorta bifurcates into the two common iliac arteries. The length of catheter to be inserted can be estimated from charts relating the required catheter length to external body measurements, or from birth weight. Positioning of the catheter is assessed by means of radiographs of the abdomen or chest: a ‘high’ catheter tip should be located in the descending aorta somewhere between the levels of the sixth and ninth thoracic vertebrae (T6–9), while a ‘low’ catheter tip should be at a level between the third and fourth lumbar vertebrae (L3–4). Relevant anatomical reference points are given in Table 73.1.
Table 73.1 Key anatomical reference points for umbilical arterial catheterization
Structure | Vertebral level |
---|---|
Ductus arteriosus | T4–5 |
Coeliac artery | T12 |
Superior mesenteric artery | T12–L1 |
Renal artery | L1 |
Inferior mesenteric artery | L3 |
Aortic bifurcation | L4–5 |
Butzner JD, Befus AD. Interactions among intraepithelial leucocytes and other epithelial cells in intestinal development and function. In: Lebenthal E, editor. Human Gastrointestinal Development, Chapter 37. New York: Raven Press, 1989.
Collins P. Embryology of the pancreas. In: Howard ER, Stringer MD, Colombani PM. Surgery of the Liver, Bile Ducts and Pancreas in Children, Part 8. London: Arnold; 2002:479-492.
Collins P. Embryology of the liver and bile ducts. In: Howard ER, Stringer MD, Colombani PM. Surgery of the Liver, Bile Ducts and Pancreas in Children, Part 3. London: Arnold; 2002:91-102.
Gershon MD. Phenotypic expression by neural crest-derived precursors of enteric neurons and glia. In: Madreson PFA, editor. Developmental and Evolutionary Aspects of the Neural Crest. New York: John Wiley, 1987.
Describes a mouse model of Hirschsprung’s disease..
Howard ER. Biliary atresia: aetiology, management and complications. In: Howard ER, Stringer MD, Colombani PM. Surgery of the Liver, Bile Ducts and Pancreas in Children, Part 3. London: Arnold; 2002:103-132.
Lebenthal E. Concepts in gastrointestinal development. In: Lebenthal E, editor. Human Gastrointestinal Development, Chapter 1. New York: Raven Press, 1989.
Lewis P, Yaron Y, Evans MI. Fetal endocrine disorders. In: Rodeck CH, Whittle MJ. Fetal Medicine: Basic Sciences and Clinical Practice, Chapter 62. Edinburgh: Churchill Livingstone; 1999:829-834.
Streeter GL. Developmental horizons in human embryos. Descriptions of age group XI, 13 to 20 somites and age group XII, 21 to 29 somites. Contrib Embryol Carnegie Inst Washington. 1942;30:211-245.
Whittle MJ. Gastrointestinal abnormalities. In: Rodeck CH, Whittle MJ. Fetal Medicine: Basic Sciences and Clinical Practice, Chapter 54. Edinburgh: Churchill Livingstone; 1999:703-714.
Reviews the diagnosis and treatment of abnormalities of the gastrointestinal tract..