5 Decision Making in Cerebral Revascularization Surgery Using Intraoperative CBF Measurements
Introduction
Surgical cerebral revascularization can be performed through various extracranial-intracranial (EC-IC) bypass approaches, using a variety of different donor and recipient vessels, interposition grafts and anastomotic techniques. The specific choice of the type of bypass is dependent on several factors, incusing the ultimate goal of the operation, as well as the availability and suitability of particular donor and recipient vessels. The indications for EC-IC bypass fall into two broad categories:1
Intraoperative blood flow measurement
Intraoperative blood flow measurements can be easily and repeatedly performed using an ultrasonic flow probe (Charbel Micro-Flowprobe; Transonics Systems Inc., Ithaca, NY) that provides flow quantification in millimeters per minute, and is available in a variety of sizes to accommodate vessels ranging from 1 to 3 mm in width. The flow probe uses the principle of ultrasonic transit time to measure flow in vessels independent of the flow velocity profile, turbulence, or hematocrit.2,3 The body of the probe consists of two ultrasonic transducers and a fixed acoustic reflector. Once the probe is placed around a vessel, a wave of ultrasound is alternately emitted from the two ultrasonic transducers, intersects the vessel, bounces off the acoustic reflector, is received by the other transducer, and converted into an electrical signal. The flow detection unit derives from these signals an accurate measure of the transit time, namely the time it has taken for the wave of ultrasound to travel from one transducer to the other. The transit time of the ultrasound is determined by the motion of blood flowing through the vessel, and the difference between the upstream and downstream transit time is used to derive the flow in millimeters per minute through the blood vessel. The accuracy of the device has been established with in vitro and in vivo studies.3
Bypass for flow augmentation
EC-IC bypass for cerebral ischemia remains controversial. Superficial temporal artery (STA)–middle cerebral artery (MCA) bypass is the mainstay of cerebral revascularization surgery in the setting of anterior circulation ischemia. The goal of the surgery is to augment flow to the ischemic hemisphere. The use of the procedure decreased markedly after the randomized EC-IC bypass study published in 1985 failed to demonstrate the efficacy of bypass over medical management for carotid occlusive disease.4 However, subsequent analysis of the EC-IC bypass trial methodology and results have identified shortcomings in the study design and implementation, and suggested that universal abandonment of cerebral revascularization for cerebral ischemia is likely not warranted.5,6 One of the primary drawbacks of the trial was the lack of hemodynamic evaluation in the selection criteria for patients enrolled in the study. No objective physiological criteria were utilized to assess cerebral blood flow, and enrolled patients may have presented with ischemic events due to embolic phenomenon or small vessel disease, which would not be expected to respond to the flow augmentation provided by bypass surgery. Subsequent studies have demonstrated that careful evaluation of patients with occlusive cerebrovascular disease can identify a subgroup with severe compromise in cerebrovascular reserve capacity, who are at higher risk for stroke,7–12 and who may be reasonable candidates for surgical revascularization. Recently, the Japanese EC-IC Bypass Trial (JET) has reported favorable results for bypass in patients with internal carotid or MCA occlusive disease with hemodynamic compromise.13 In the United States, the role of STA-MCA bypass for recently symptomatic carotid occlusion is being re-evaluated by the Carotid Occlusion Surgery Study (COSS), which selects patients as candidates only if they demonstrate hemodynamic impairment on positron emission tomography (PET).14
Bypass Technique
Standard surgical technique is applied to performing the bypass.15,16 Briefly, for STA-MCA bypass surgery, a linear incision is placed along the course of the STA branch, and the vessel is dissected for a distance of approximately 8 cm. The temporalis fascia and muscle are divided and a craniotomy is performed. A recipient cortical MCA vessel is freed of its arachnoid attachments in preparation for anastomosis. The STA vessel is cleared of its connective tissue distally, cut at an oblique angle, and fish-mouthed. An end-to-side anastomosis is performed to the recipient cortical MCA branch under temporary occlusion, utilizing interrupted or continuous 10-0 nylon sutures. The dural opening is covered with gelfoam, and the bone flap replaced, removing any portion of the flap that may lead to pressure on the STA. The muscle is loosely re-approximated and the skin closed. For OA-PICA bypass, the occipital artery is dissected from beneath the skin and muscle flap after creating a posterior hockey-shaped suboccipital incision; a lateral suboccipital craniotomy extending across midline is performed. An anastomosis is created between the OA and the tonsillomedullary loop of the PICA, running below the edge of the cerebellar tonsil. For the STA-SCA bypass, or its variant, the STA–posterior cerebral artery (PCA) bypass, the recipient vessel is exposed via a subtemporal approach, as it courses around the midbrain. Anastomosis is performed under temporary vessel occlusion along a perforator-free segment of the SCA or PCA.
Intraoperative blood flow measurement technique
Application of intraoperative flow measurements to flow augmentation bypass entails making flow measurements at two points during the surgery. The first flow measurement needed is the cut flow (Figure 5–1A). The cut flow refers to the maximal potential flow capacity of the in situ donor vessel (STA or OA) once it has been dissected and cut open. The flow in the intact STA or OA in situ is generally very low, less than 5 to 10 ml/min, because of the small caliber of the vessel and the high resistance of the distal scalp tissue bed. However, once the vessel is dissected free from its surrounding tissue, and its distal end is cut, the full carrying capacity of the vessel in the absence of downstream resistance can be determined. This constitutes the cut flow of the vessel. Prior to flow measurement, the vessel is wrapped in papaverine-soaked cottonoids following dissection to prevent any reductions in flow related to spasm induced by vessel manipulation.
The second flow measurement of importance is the “bypass flow” (Figure 5–1B). Following the anastomosis, the flow in the STA or OA graft is re-measured, which constitutes the flow in the bypass. If the resistance in the cortical recipient bed is sufficiently low, as would be expected in patients with hemodynamic compromise due to cerebrovascular occlusive disease, the bypass flow would be expected to approximate the cut flow of the donor vessel. This can be quantified as a cut flow index (CFI):
Interpretation of intraoperative blood flow measurements
The CFI serves as a useful indicator of bypass function and long-term success. In a series of 51 bypass operations performed for flow augmentation in 47 patients, CFI was found to be a significant predictor of bypass patency (p < 0.01).17 Using a CFI of 0.5 as a threshold, the bypass patency rate was 92% in cases when CFI > 0.5, compared with 50% in cases when the CFI < 0.5. Critical examination of the cases with poor CFI revealed that a logical interpretation of bypass function can be performed intraoperatively. A low CFI can be a result of varying forms of intrinsic or extrinsic errors, which can be classified as follows:
Recognition of these errors may direct intraoperative actions, such as reopening and revising an anastomosis for a Type 2B error, or considering a second bypass to a separate recipient using the anterior STA branch for a Type 2C error (as could have been performed for the case demonstrated in Figure 5–2).
Overall, intraoperative flow measurements allow immediate verification of bypass patency. Additionally, in flow augmentation bypass, the CFI provides a sensitive predictor of postoperative bypass patency. CFI > 0.5 predicts high rates of postoperative bypass patency, whereas a poor CFI can alert surgeons to potential difficulties with the donor vessel, anastomosis, or recipient vessel intraoperatively, allowing the opportunity to rectify the problem (Figure 5–3). Furthermore, a CFI closely approximating 1.0 provides physiologic confirmation of impaired cerebrovascular reserve in the recipient bed. Mere bypass patency can be judged intraoperatively with other techniques including intraoperative micro-Doppler, indocyanine green (ICG) angiography, or conventional angiography. However, given that bypasses with a low CFI are often patent at the time of surgery, the predictive value of CFI highlights the notion that mere anatomic patency may not be as useful in predicting a successful bypass procedure as the assessment of quantitative intraoperative bypass flow.
Bypass for flow replacement
Definitive management of giant or complex aneurysms may require sacrifice of the parent vessel. Permanent occlusion of the ICA has been successfully used to treat inoperable intracranial aneurysms, and similarly, complete excision of skull base tumors occasionally requires resection of a major vessel.18,19
Although carotid sacrifice may be tolerated, ischemia and subsequent stroke can occur in up to 30% of patients.18 In young patients with a risk of de novo aneurysms over time, or in patients with existing contralateral aneurysm, bypass may be routinely warranted. Otherwise, tolerance to carotid sacrifice is most frequently evaluated using endovascular balloon occlusion testing (BOT), during which the response to temporary carotid occlusion is evaluated. Tolerance to occlusion is based upon several criteria including neurologic, radiographic, electrophysiological, perfusion, and provocative testing. Neurological criteria are the primary modality of evaluation, consisting of continuous monitoring of the patient for symptoms or signs of cerebral ischemia during a period of 20 to 30 minutes. This can be supplemented with electrophysiological monitoring with the use of electroencephalography (EEG) or evoked potentials.20 Radiographic features such as the degree of cross-filling from the communicating arteries and the speed of venous filling in the affected hemisphere can also be used to assess adequacy of collaterals.21,22 Perfusion imaging with modalities such as Xenon computed tomography (CT) and single-photon emission tomography (SPECT) can be used to further assess collateral reserve during test occlusion.23–25 Provocative testing can be added for those patients who tolerate the initial segment of BOT, by providing an additional hypotensive challenge. Patients who fail BOT require revascularization prior to carotid sacrifice to avert ischemia.26 The results of BOT in addition to intraoperative assessment using flow measurements can help to determine the best strategy for revascularization.
Bypass Technique
Terminal aneurysm flow measurements
For fusiform or complex terminal artery aneurysms located on arteries beyond the anastomotic connections of the Circle of Willis such as the ICA terminus, MCA, PCA, PICA, and ICA terminus, the standard cranial approaches are utilized. Once the aneurysm and associated vessels are exposed using standard microdissection, flow measurements are obtained in all distal vessels that could be compromised by trapping of the aneurysm. For example, the M2 branches would represent the distal vessels in the case of an M1 aneurysm (Figure 5–4), or the PICA trunk would represent the distal vessel in a PICA origin aneurysm. The cumulative flow in the distal vasculature reflects the distal territory flow requiring replacement via the bypass.
Proximal ICA aneurysm flow measurements
For proximal ICA aneurysms (Figure 5–5), including cavernous segment, ophthalmic, and paraclinoid aneurysms, the operation is performed with simultaneous exposure of the cervical ICA in the neck, while performing a standard pterional craniotomy on the ipsilateral side. Following craniotomy, the sylvian fissure is routinely opened with microdissection, exposing the M1 and A1 segments. Flow in the M1 and A1 (if the ACA territory is at risk) is measured and recorded at baseline, and then repeated following temporary occlusion of the ICA. Temporary occlusion is performed on the cervical ICA when the planned surgical intervention is to be proximal occlusion or on the supraclinoid ICA distal to the aneurysm if the surgical plan is to be trapping of the lesion. The decrement in M1 flow, and A1 flow if relevant, during temporary vessel occlusion is designated as the flow deficit.
Donor vessel flow measurement
The flow capacity of in situ pedicle grafts (STA or OA) is directly measured to determine their adequacy for replacing the distal territory flow in terminal aneurysms or the flow deficit in proximal ICA aneurysms. This is performed by determining the cut flow of the donor graft,17 as described earlier, as this reflects the maximal potential flow capacity of the vessel. Once the vessel has been dissected free from surrounding tissues, it is wrapped in papaverine-soaked cottonoids for 20 to 30 minutes during the time that the craniotomy is performed, to relieve any spasm induced by vessel manipulation. The flow is then measured shortly prior to planned bypass.
Interpretation of intraoperative blood flow measurements
Once the distal territory flow for terminal aneurysms, or flow deficit in proximal ICA aneurysms, has been determined, the selection of bypass can then be tailored to the flow replacement needed (Figure 5–6). If the cut flow of the in situ STA or OA approaches or exceeds the flow required for adequate flow replacement, the in situ graft can be utilized for the bypass. Existing data regarding blood flow and perfusion assessment during vessel occlusion indicate that a 20% to 25% or greater reduction in distal flow is correlated with ischemia.27–29 Therefore, to avoid risk of ischemia, only a cut flow within at least 20% of the recipient territory flow can be accepted as adequate. It is also important to note that the cut flow is generally measured prior to cutting the graft to its final length in order to allow heparin flushing of the vessel distal to the ultimate anastomosis site (in order to prevent risk of endothelial injury from the flushing needle). For vessels of small diameter such as the STA, the reduction in length of the graft with final trimming will inevitably result in some increase in carrying capacity. If the in situ donor vessel flow is inadequate, an interposition graft is necessary to ensure adequate flow replacement. In such cases, the choice of a long interposition graft to the cervical ICA or common carotid artery (CCA) versus a short interposition graft to the stump of the STA can also be determined by measuring the flow in the STA stump once the vessel has been truncated.
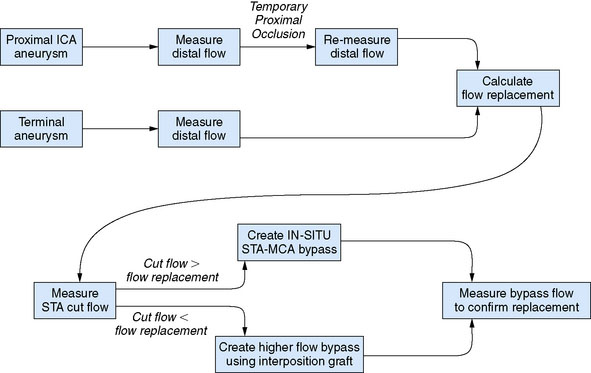
Figure 5–6 Flow measurement algorithm for flow replacement bypass.
(Adapted from Ashley WW, Amin-Hanjani S, Alaraj A, et al: Flow-assisted surgical cerebral revascularization, Neurosurg Focus 24:E20, 2008.)
Intraoperative flow measurements can also be particularly valuable in cases when the need for carotid sacrifice is unanticipated at the time of surgery or when BOT cannot be performed safely preoperatively (as in patients with ruptured aneurysms, or in poor neurological condition). The need for bypass can be tested by temporary clipping of the carotid to determine whether a flow deficit is present. A deficit of >20% would be the cut-off correlated with ischemia, and would indicate the need for bypass for flow replacement. If no significant flow deficit is encountered, suggesting adequate collaterals, a confirmatory vasodilatory challenge can be performed intraoperatively. This is accomplished by increasing the end-tidal CO2 by 10 Torr, and determining whether there is an appropriate increase in flow to confirm cerebrovascular reserve via the collaterals.30 This intraoperative version of a carotid occlusion test, if passed, would provide assurance that vessel sacrifice without the need for bypass can be undertaken.
Perioperative flow measurement
Direct vessel flow measurement prior to and after EC-IC bypass can also provide useful information for surgical planning and for postoperative follow-up. Noninvasive flow measurements can be performed with quantitative MRA (QMRA), which provides the ability to measure flow in millimeters per minute directly in vessels of interest27,31–34 using phase-contrast MR (PCMR) techniques. QMRA can be readily performed using commercially available software called NOVA (Non-invasive Optimal Vessel Analysis) system (VasSol, Inc., Chicago, IL): an axial three-dimensional (3-D) time-of-flight MRA is first performed to create a 3-D surface rendering of the vasculature, including the circle of Willis, generated using a marching cube algorithm. Vessels including bypass grafts can then be easily identified via the rotating 3-D image. A cut perpendicular to the axis of the vessel is automatically generated when picked in the 3-D image. A double-oblique cine PCMR scan is then performed based on the prescription values of the perpendicular cut from the NOVA system, and a flow value in millimeters per minute obtained.
Preoperative Planning
In patients under consideration for flow augmentation bypass, preoperative QMRA provides a measure of intracranial large vessel flows and can be used as an indicator of reduced flow to a vascular territory (Figure 5–7). These measurements can also provide an indication of the presence, quantity, and pattern of collateral flow through other vessels to the affected territory. When combined with challenge testing, cerebrovascular reserve can be evaluated by determining whether the primary arterial vessel supplying a given territory has the appropriate increase in flow in response to a vasodilatory agent such as acetozolamide. In conjunction with tissue level perfusion imaging, the large vessel flows obtained with QMRA can be utilized to assess hemodynamic compromise.35
When flow replacement bypass with parent vessel sacrifice is planned, QMRA provides measures of blood flow in the distal vessels (Figure 5–8). This serves as a baseline measure that can be used for postoperative comparison. The flows can also provide some indication of the flow replacement that will be required, although in our experience, it is expected that vessel flows measured intraoperatively will be ≥40% lower under anesthesia than when measured by QMRA in the awake state.
Postoperative Surveillance
In flow replacement bypass, postoperative QMRA measurement of flow within the graft and within the revascularized vessels can be compared to baseline to confirm the ultimate success of the bypass strategy (Figure 5–8). In flow augmentation bypass, the graft flow provides a quantitative indication of the graft’s ability to provide additional flow to the ischemic territory. Furthermore, flow measurements provide a reliable method for assessing long-term patency and function of bypass grafts. Bypass function can worsen over time, with failure occurring at an average of 2.7 years for STA-MCA grafts, and 1.4 years for vein grafts in one series of 47 patients treated for ICA occlusion.36 Serial follow-up is therefore an important aspect in judging the long-term success of the bypass.
In a series of 62 bypass cases comparing conventional angiography and QMRA for postoperative bypass evaluation and surveillance, excellent correlation was found between the modalities.37 Occluded bypasses (n = 4) were consistently not visualized on QMRA. Flow rates were found to be significantly lower in bypasses that were stenotic or diminutive in caliber over time, compared to those that remained fully patent on angiography. All angiographically poor bypasses were identifiable by flow rates of <20 ml/min or a reduction in bypass flow of >30% within 3 months, indicating that a low or rapidly declining flow is an indicator of a failing graft.
1 Charbel F.T., Guppy K.H., Ausman J.I. Cerebral revascularization: Superficial temporal middle cerebral artery anastomosis. In: Sekhar L.N., Fessler R.G., editors. Atlas of Neurosurgical Techniques. New York: Thieme; 2006:370-378.
2 Charbel F.T., Hoffman W.E., Misra M., et al. Ultrasonic perivascular flow probe: technique and application in neurosurgery. Neurol Res. 1998;20:439-442.
3 Lundell A., Bergqvist D., Mattsson E., et al. Volume blood flow measurements with a transit time flowmeter: an in vivo and in vitro variability and validation study. Clin Physiol. 1993;13:547-557.
4 The EC/IC Bypass Study Group. Failure of extracranial-intracranial arterial bypass to reduce the risk of ischemic stroke. Results of an international randomized trial. N Engl J Med. 1985;313:1191-1200.
5 Goldring S., Zervas N., Langfitt T. The Extracranial-Intracranial Bypass Study. A report of the committee appointed by the American Association of Neurological Surgeons to examine the study. N Engl J Med. 1987;316:817-820.
6 Sundt T.M.Jr. Was the international randomized trial of extracranial-intracranial arterial bypass representative of the population at risk? N Engl J Med. 1987;316:814-816.
7 Grubb R.L.Jr, Derdeyn C.P., Fritsch S.M., et al. Importance of hemodynamic factors in the prognosis of symptomatic carotid occlusion. JAMA. 1998;280:1055-1060.
8 Kleiser B., Widder B. Course of carotid artery occlusions with impaired cerebrovascular reactivity. Stroke. 1992;23:171-174.
9 Kuroda S., Houkin K., Kamiyama H., et al. Long-term prognosis of medically treated patients with internal carotid or middle cerebral artery occlusion: can acetazolamide test predict it? Stroke. 2001;32:2110-2116.
10 Ogasawara K., Ogawa A., Yoshimoto T. Cerebrovascular reactivity to acetazolamide and outcome in patients with symptomatic internal carotid or middle cerebral artery occlusion: a xenon-133 single-photon emission computed tomography study. Stroke. 2002;33:1857-1862.
11 Vernieri F., Pasqualetti P., Passarelli F., et al. Outcome of carotid artery occlusion is predicted by cerebrovascular reactivity [see comment]. Stroke. 1999;30:593-598.
12 Webster M.W., Makaroun M.S., Steed D.L., et al. Compromised cerebral blood flow reactivity is a predictor of stroke in patients with symptomatic carotid artery occlusive disease. J Vasc Surg. 1995;21:338-344. discussion 344–345
13 JET Study Group. Japanese EC-IC bypass Trial (JET Study): The second interim analysis. Surg Cereb Stroke (Jpn). 2002;30:434-437.
14 Grubb R.L.Jr, Powers W.J., Derdeyn C.P., et al. The carotid occlusion surgery study. Neurosurg Focus. 2003;14:e9.
15 Charbel F.T., Meglio G., Amin-Hanjani S. Superficial temporal artery-to-middle cerebral artery bypass. Neurosurgery. 2005;56:186-190.
16 Ausman J.I., Diaz F.G., Vacca D.F., et al. Superficial temporal and occipital artery bypass pedicles to superior, anterior inferior, and posterior inferior cerebellar arteries for vertebrobasilar insufficiency. J Neurosurg. 1990;72:554-558.
17 Amin-Hanjani S., Du X., Mlinarevich N., et al. The cut flow index: an intraoperative predictor of the success of extracranial-intracranial bypass for occlusive cerebrovascular disease. Neurosurgery. 2005;56:75-85.
18 Nishioka H. Results of the treatment of intracranial aneurysms by occlusion of the carotid artery in the neck. J Neurosurg. 1966;25:660-704.
19 Drake C.G., Peerless S.J., Ferguson G.G. Hunterian proximal arterial occlusion for giant aneurysms of the carotid circulation. J Neurosurg. 1994;81:656-665.
20 Liu A.Y., Lopez J.R., Do H.M., et al. Neurophysiological monitoring in the endovascular therapy of aneurysms. AJNR Am J Neuroradiol. 2003;24:1520-1527.
21 Abud D.G., Spelle L., Piotin M., et al. Venous phase timing during balloon test occlusion as a criterion for permanent internal carotid artery sacrifice. AJNR Am J Neuroradiol. 2005;26:2602-2609.
22 van Rooij W.J., Sluzewski M., Slob M.J., et al. Predictive value of angiographic testing for tolerance to therapeutic occlusion of the carotid artery. AJNR Am J Neuroradiol. 2005;26:175-178.
23 Lorberboym M., Pandit N., Machac J., et al. Brain perfusion imaging during preoperative temporary balloon occlusion of the internal carotid artery. J Nucl Med. 1996;37:415-419.
24 Witt J.P., Yonas H., Jungreis C. Cerebral blood flow response pattern during balloon test occlusion of the internal carotid artery. AJNR Am J Neuroradiol. 1994;15:847-856.
25 Eckard D.A., Purdy P.D., Bonte F.J. Temporary balloon occlusion of the carotid artery combined with brain blood flow imaging as a test to predict tolerance prior to permanent carotid sacrifice. AJNR Am J Neuroradiol. 1992;13:1565-1569.
26 Amin-Hanjani S., Charbel F.T. Is extracranial-intracranial bypass surgery effective in certain patients? Neurol Clin. 2006;24:729-743.
27 Charbel F.T., Zhao M., Amin-Hanjani S., et al. A patient-specific computer model to predict outcomes of the balloon occlusion test. J Neurosurg. 2004;101:977-988.
28 Eckert B., Thie A., Carvajal M., et al. Predicting hemodynamic ischemia by transcranial Doppler monitoring during therapeutic balloon occlusion of the internal carotid artery. AJNR Am J Neuroradiol. 1998;19:577-582.
29 Jawad K., Miller D., Wyper D.J., et al. Measurement of CBF and carotid artery pressure compared with cerebral angiography in assessing collateral blood supply after carotid ligation. J Neurosurg. 1977;46:185-196.
30 Ashley W.W., Amin-Hanjani S., Alaraj A., et al. Flow-assisted surgical cerebral revascularization. Neurosurg Focus. 2008;24:E20.
31 Neff K.W., Horn P., Dinter D., et al. Extracranial-intracranial arterial bypass surgery improves total brain blood supply in selected symptomatic patients with unilateral internal carotid artery occlusion and insufficient collateralization. Neuroradiology. 2004;46:730-737.
32 van Everdingen K.J., Klijn C.J., Kappelle L.J., et al. MRA flow quantification in patients with a symptomatic internal carotid artery occlusion. The Dutch EC-IC Bypass Study Group. Stroke. 1997;28:1595-1600.
33 Guppy K.H., Charbel F.T., Corsten L.A., et al. Hemodynamic evaluation of basilar and vertebral artery angioplasty. Neurosurgery. 2002;51:327-333. discussion 333–334
34 Zhao M., Charbel F.T., Alperin N., et al. Improved phase-contrast flow quantification by three-dimensional vessel localization. Magn Reson Imaging. 2000;18:697-706.
35 Thulborn K.R. MRI in the management of cerebrovascular disease to prevent stroke. Neurol Clin. 2008;26:897-921. vii–viii
36 Schick U., Zimmermann M., Stolke D. Long-term evaluation of EC-IC bypass patency. Acta Neurochir (Wien). 1996;138:938-942. discussion 942–943
37 Amin-Hanjani S., Shin J., Zhao M., et al. Evaluation of extracranial-intracranial bypass using quantitative magnetic resonance angiography. J Neurosurg. 2007;106:291-298.