Chapter 4 Cranial Meninges
The brain and spinal cord are entirely enveloped by three concentric membranes, the meninges, which provide support and protection. The outermost meningeal layer is the dura mater (pachymeninx). Beneath this lies the arachnoid mater. The innermost layer is the pia mater. The dura is an opaque, tough, fibrous coat. It incompletely divides the cranial cavity into compartments and accommodates the dural venous sinuses. It is separated from the arachnoid by a narrow subdural space. The arachnoid mater and pia mater are sometimes referred to collectively as the leptomeninges, and they share many similarities. The arachnoid is much thinner than the dura and is mostly translucent. It surrounds the brain loosely, spanning depressions and concavities. Beneath the arachnoid lies the subarachnoid space, which contains cerebrospinal fluid (CSF), secreted by the choroid plexuses of the cerebroventricular system. The pia mater is a transparent, microscopically thin membrane that follows the contours of the brain and is closely adherent to its surface. The subarachnoid space varies greatly in depth, and the larger expanses are termed subarachnoid cisterns. CSF circulates within the subarachnoid space and is reabsorbed into the venous system through arachnoid villi and granulations associated with the dural venous sinuses. Cranial and spinal meninges are continuous through the foramen magnum. Only the cranial meninges are described in this chapter.
Dura Mater
CASE 1 EPIDURAL HAEMATOMA
An EDH can readily be seen on an unenhanced head CT scan and typically has a lens-shaped appearance, as it lies in the potential space between the dura and the calvaria (Fig. 4.1). It does not cross the cranial suture lines because at those locations the dura is tightly adherent to the skull. Emergency surgery is required in most cases to relieve the pressure caused by the haematoma and, if possible, identify the source of bleeding.
Dural Partitions
Falx Cerebri
The falx cerebri is a strong, crescent-shaped sheet of dura mater lying in the sagittal plane and occupying the great longitudinal fissure between the two cerebral hemispheres (Figs. 4.2, 4.3). The crescent is narrow in front, where the falx is fixed to the crista galli, and broad behind, where it blends into the midline with the tentorium cerebelli. The anterior part of the falx is thin and may have a number of irregular perforations (see Fig. 4.2). Its convex upper margin is attached to the internal cranial surface on each side of the midline, as far back as the internal occipital protuberance. The superior sagittal sinus runs within the dura along this margin, in a cranial groove, and the falx is attached to the lips of this groove. At its lower edge, the falx is free and concave and contains the inferior sagittal sinus. The straight sinus runs along the line of attachment of the falx to the tentorium cerebelli (see Fig. 4.2).
Tentorium Cerebelli
The tentorium cerebelli (Figs. 4.2–4.4) is a sheet of dura mater with a peaked configuration reminiscent of a single-poled tent, from which its name is derived. It covers the cerebellum and passes under the occipital lobes of the cerebral hemispheres. Its concave anterior edge is free; between it and the dorsum sellae of the sphenoid bone is a large curved hiatus (the tentorial incisure or notch), which is occupied by the midbrain and the anterior part of the superior aspect of the cerebellar vermis. The tentorium divides the cranial cavity into supratentorial and infratentorial compartments that contain the forebrain and hindbrain, respectively. The convex outer limit of the tentorium is attached posteriorly to the lips of the transverse sulci of the occipital bone and the posteroinferior angles of the parietal bones, where it encloses the transverse sinuses. Laterally, the tentorium is attached to the superior borders of the petrous temporal bones, where it contains the superior petrosal sinuses (see Fig. 4.3). Near the apex of the petrous temporal bone, the lower layer of the tentorium is evaginated anterolaterally under the superior petrosal sinus to form a recess between the endosteal and meningeal layers in the middle cranial fossa. This recess is the trigeminal cave (Meckel’s cave) and contains the roots and ganglion of the trigeminal nerve. The evaginated meningeal layer fuses in front with the anterior part of the trigeminal ganglion. At the apex of the petrous temporal bone, the free border and attached periphery of the tentorium cross each other (see Fig. 4.4). The anterior ends of the free border are fixed to the anterior clinoid processes, and the attached periphery is fixed to the posterior clinoid processes. The oculomotor nerve lies in the groove between them on each side.
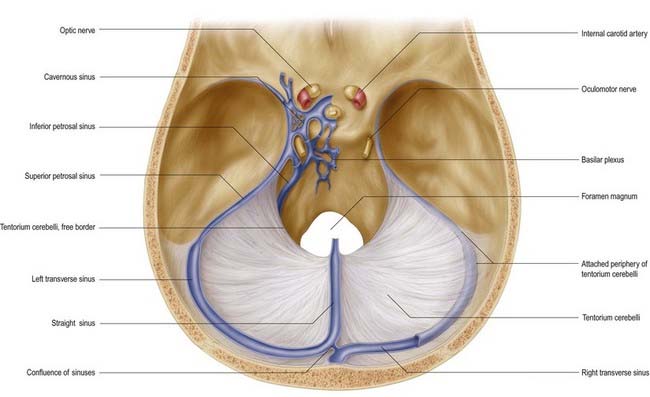
Fig. 4.4 Dura mater of the floor of the cranial cavity and the superior aspect of the tentorium cerebelli. Representations of the cavernous sinus and its venous relationships are greatly simplified and are shown on the left only. Note that the trochlear and abducens nerves are not shown (see Fig. 4.6).
Falx Cerebelli
The falx cerebelli is a small midline fold of dura mater lying below the tentorium cerebelli. It projects forward into the posterior cerebellar notch between the cerebellar hemispheres. Its base is directed upward and attached to the posterior part of the inferior surface of the tentorium cerebelli in the midline. Its posterior margin is attached to the internal occipital crest and contains the occipital sinus. The apex of the falx cerebelli frequently divides into two small folds, which disappear at the sides of the foramen magnum. Frequently the falx is double.
Diaphragma Sellae
The diaphragma sellae (see Fig. 4.2) is a small, circular, horizontal sheet of dura mater that forms a roof to the sella turcica and, in many cases, almost completely covers the pituitary gland (hypophysis). The central opening in the diaphragma allows the infundibulum and pituitary stalk to pass into the pituitary fossa. There is wide individual variation in the size of the central opening. The diaphragma sellae was an important landmark in pituitary surgery in the past—extension of a pituitary tumour above it was an indication for a subfrontal approach through a craniotomy. A transsphenoidal approach is currently preferred, irrespective of whether there is suprasellar extension.
The arrangement of the dura mater in the central part of the middle cranial fossa is complex (see Fig. 4.4). The tentorium cerebelli forms a large part of the floor of the middle cranial fossa and fills much of the gap between the ridges of the petrous temporal bones. On both sides, the rim of the tentorial incisure is attached to the apex of the petrous temporal bone and continues forward as a ridge of dura mater to attach to the anterior clinoid process. This ridge marks the junction of the roof and the lateral part of the cavernous sinus (Figs. 4.5, 4.6). The periphery of the tentorium cerebelli is attached to the superior border of the petrous temporal bone, crosses under the free border of the tentorial incisure and continues forward to the posterior clinoid processes as a rounded, indefinite ridge of the dura mater. Thus, an angular depression exists between the anterior parts of the peripheral attachment of the tentorium and the free border of the tentorial incisure (see Figs. 4.2, 4.4). This depression in the dura mater is part of the roof of the cavernous sinus and is pierced in front by the oculomotor nerve and behind by the trochlear nerve, which proceed anteroinferiorly into the lateral wall of the cavernous sinus (Fig. 4.7). In the anteromedial part of the middle cranial fossa, the dura mater ascends as the lateral wall of the cavernous sinus. It reaches the ridge produced by the anterior continuation of the free border of the tentorium and runs medially as the roof of the cavernous sinus, where it is pierced by the internal carotid artery (see Figs. 4.2, 4.4). Medially, the roof of the sinus is continuous with the upper layer of the diaphragma sellae. At or just below the opening in the diaphragma for the infundibulum and pituitary stalk, the dura, arachnoid and pia mater blend with one another and with the capsule of the pituitary gland. It is not possible to distinguish the layers of the meninges within the sella turcica, and the subarachnoid space is obliterated.
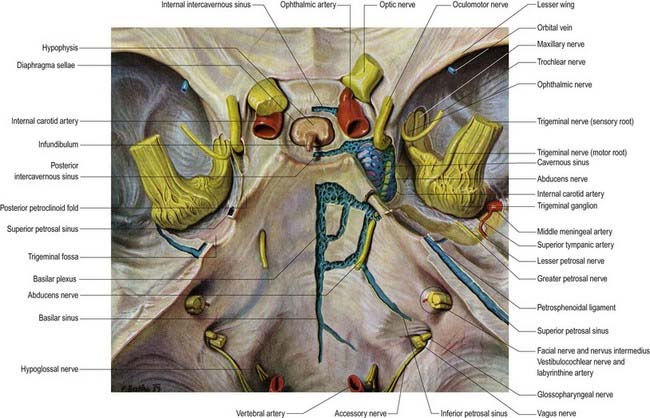
Fig. 4.6 Middle cranial fossa, viewed from above to show the cavernous and related sinuses. These have been exposed by partial removal of the dura matter. The trigeminal, trochlear and oculomotor nerves have been reflected forward on both sides.
Dural Venous Sinuses
Dural venous sinuses (see Fig. 6.16) are a complex of venous channels that lie between the two layers of dura mater, draining blood from the brain and cranial bones. They are lined by endothelium and have no valves; their walls are devoid of muscular tissue. Developmentally, the venous sinuses emerge as venous plexuses, and most sinuses preserve a plexiform arrangement to a variable degree rather than being simple vessels with a single lumen. Browder and Kaplan (1976) examined human venous sinuses in hundreds of corrosion casts and observed vascular plexuses adjoining the superior and inferior sagittal and straight sinuses and, with a lower incidence, the transverse sinuses. There was much individual variation, and departures from ‘average’ patterns were frequent in early life; for example, in infancy, the falx cerebelli may contain large plexiform channels and venous lacunae, augmenting the occipital sinus. These variations cannot be detailed in a general text. They must be established on an individual basis by angiography when the clinical necessity arises. However, it is important to emphasize the wide variation possible in the structure of cranial venous sinuses, together with their plexiform nature and wide connections with cerebral and cerebellar veins. Another kind of connection has been shown experimentally. Parts of sinuses (and even diploic veins) can be filled by forcible internal carotid injection, suggesting the existence of arteriovenous shunts (Browder and Kaplan 1976). A connection between the middle meningeal arteries and the superior sagittal sinus has been demonstrated in this way, although the sites of communication are unknown.
Superior Sagittal Sinus
The superior sagittal sinus runs in the attached, convex margin of the falx cerebri; it grooves the internal surface of the frontal bone, the adjacent margins of the two parietal bones and the squamous part of the occipital bone (Fig. 4.8; see also Figs. 4.2, 6.16). It begins near the crista galli, a few millimetres posterior to the foramen caecum, and receives primary tributaries from cortical veins of the frontal lobes, the ascending frontal veins. Narrow anteriorly, the sinus runs backward, gradually widening to approximately 1 cm. Near the internal occipital protuberance it deviates, usually to the right, and continues as a transverse sinus. Triangular in cross-section, the interior of the superior sagittal sinus possesses the openings of superior cerebral veins and projecting arachnoid granulations. It is traversed by many fibrous bands. It also communicates by small orifices with irregular venous lacunae, situated in the dura mater near the sinus. There are usually two or three of these on each side—a small frontal, a large parietal and an intermediate-sized occipital. In the elderly, the lacunae tend to become confluent, so there is one elongated lacuna on each side. Fine fibrous bands cross them, and numerous arachnoid granulations project into them. The superior sagittal sinus receives the superior cerebral veins and, near the posterior end of the sagittal suture, veins from the pericranium, which pass through the parietal foramina. The lacunae also drain the diploic veins and meningeal veins.
The dilated posterior end of the superior sagittal sinus is referred to as the confluence of the sinuses (see Fig. 4.4). This is situated to one side (usually the right) of the internal occipital protuberance, where the superior sagittal sinus turns to become a transverse sinus. It also connects with the occipital and contralateral transverse sinus. The size and degree of communication of the channels meeting at the confluence are highly variable. In more than half of subjects, all venous channels that converge toward the occiput interconnect, including the straight and occipital sinuses. In many instances, however, communication is absent or tenuous. Any sinus involved may be duplicated, narrowed or widened near the confluence.