Chapter 15 Coronary Artery Disease Detection
Pharmacologic Stress SPECT
INTRODUCTION
Pharmacologic stress is used in approximately 40% of the stress myocardial perfusion imaging (MPI) studies performed for the detection of coronary artery disease (CAD) in the United States.1 Approximately 75% of inpatients, 40% of outpatients, 30% of patients younger than 75 years, and 50% of patients older than 75 years cannot perform maximal exercise.2 Submaximal exercise lowers the sensitivity of exercise MPI and underestimates the degree and extent of ischemia.3 Exercise is the preferred stress modality for patients who can exercise and achieve adequate exercise endpoints, whereas pharmacologic stress is reserved for patients who have left bundle branch block (LBBB) electronically paced rhythms, or are unable to exercise or achieve adequate exercise endpoints (Table 15-1).3–5 Pharmacologic stress agents include vasodilators such as adenosine, dipyridamole, adenosine triphosphate (ATP), or the newer selective adenosine A2A receptor agonists and inotropic and chronotropic agents such as dobutamine and arbutamine. Adenosine triphosphate and arbutamine are not used in the United States and thus will not be discussed in this chapter.
Table 15-1 Factors That Favor Pharmacologic Myocardial Perfusion Imaging
From Zoghbi G, Iskandrian AE: Pharmacologic stress testing. In Garcia EV, Iskandrian AE (eds): Nuclear Cardiac Imaging: Principles and Applications. New York: Oxford University Press, 2008, pp 293-315, with permission.
PHARMACOLOGY
Adenosine
Adenosine, a small heterocyclic compound made of a purine base and a sugar ribose, is produced endogenously by endothelial and vascular smooth muscle cells in small amounts during normal cellular conditions and in larger amounts under ischemic conditions.6 The intracellular adenosine production involves two different pathways. In the S-adenosyl pathway, S-adenosyl methionine is converted to adenosine and homocysteine via the intermediary of S-adenosyl homocysteine.1,3,6 In the ATP pathway that predominates during episodes of ischemia, ATP is dephosphorylated sequentially to adenosine diphosphate (ADP), adenosine monophosphate (AMP), and finally to adenosine and phosphate by the catalytic action of a 5′nucleotidase.1,3,6 A carrier-mediated transporter transports the produced adenosine into the extracellular space, where it interacts with its cell membrane receptors on endothelial and smooth muscle cells.1,3,6 It subsequently reenters the intracellular space of endothelial cells, smooth muscle cells, or red blood cells via facilitated transport, where it gets degraded to xanthine and uric acid in a pathway that involves adenosine deaminase, nucleoside phosphorylase, and xanthine oxidase or to AMP via an adenosine kinase pathway.1,3,6 Adenosine can also be produced extracellularly from the dephosphorylation of ATP and ADP that are released from mast cells, nerve endings, or platelets.1
Adenosine receptors are divided into at least four types: A1, A2A, A2B, and A3.1,3,6,7 The different adenosine receptor subtypes, their location, and action are listed in Table 15-2.1,7,8 Activation of the A2A receptor causes coronary vasodilatation, and activation of the other receptors is responsible for undesirable effects.8 Adenosine binding to the A2 receptor activates adenylate cyclase and increases intracellular cyclic AMP production via Gs protein activation.8 This causes opening of potassium channels, inhibition of voltage-gated calcium channels, and hyperpolarization of smooth muscle cells, with resultant decrease in calcium uptake and intracellular calcium release that lead to smooth muscle relaxation and arteriolar dilation.3,8 Adenosine binding to A1 receptors inhibits norepinephrine release, stimulates endothelial-derived relaxing factor production, and activates Gi proteins that inhibit adenylate cyclase, decrease intracellular cyclic AMP, and increase potassium channel conductance—all of which result in smooth muscle contraction.1,3,8 Adenosine also has direct effects on sympathetic nerve endings, resulting in the increase in heart rate (HR) and the rise in blood pressure (BP) seen occasionally.1 Xanthine-containing compounds, such as theophylline and caffeine, competitively inhibit the action of adenosine on these receptors.4
Receptor Type | Location | Action |
---|---|---|
A1 |
From Zoghbi G, Iskandrian AE: Pharmacologic stress testing. In Garcia EV, Iskandrian AE (eds): Nuclear Cardiac Imaging: Principles and Applications. New York: Oxford University Press, 2008, pp 293-315, with permission.
Adenosine has a very short half-life of less than 2 seconds and a rapid onset of action.4,9 Its peak hyperemic effect is reached within 2 minutes after beginning its infusion and returns to baseline within 2 minutes after termination of its infusion.4,9
Dipyridamole
Dipyridamole, a pyrimidine base, increases interstitial adenosine level by inhibiting adenosine-facilitated reuptake across vascular, endothelial, and red blood cell membranes that are responsible for its degradation.3,4 Thus, dipyridamole indirectly increases endogenous adenosine production at its receptor sites, mediating its various effects.3,4 Its peak vasodilatory effect occurs within 3 to 7 minutes from beginning of infusion, and its half-life lasts around 30 to 45 minutes.3,4
Dobutamine
Dobutamine is a synthetic sympathomimetic amine that has a direct weak β2 and α1 receptor agonist activity and a strong β1 agonist activity with dose-dependent inotropic and chronotropic effects.3,10 Dobutamine doses less than 10 μg/kg/min cause cardiac β1 and peripheral α1 receptor stimulation resulting in augmentation of stroke volume and cardiac output and minimal changes in HR and BP.3,10 Dobutamine doses over 10 μg/kg/min cause predominant cardiac β1 receptor stimulation resulting in significant dose-dependent increases in HR, contractility, and cardiac output, with minimal increase in systemic BP.3,10 The systemic BP occasionally decreases due to peripheral vasodilatation caused by more effects on the peripheral β2 receptor.3,10,11 Myocardial blood flow (MBF) increases, predominantly due to the increase in myocardial oxygen demand and a minimal direct vasodilatory effect on the coronary bed.3,10,11
Dobutamine’s onset of action is within 2 minutes of its infusion and reaches steady state after several minutes.3,10,11 Dobutamine has a 2-minute half-life and is metabolized in the liver through methylation and conjugation.11 The cardiovascular effects of the various pharmacologic stressors are summarized in Table 15-3.
MYOCARDIAL BLOOD FLOW
The hemodynamic significance of coronary lesions can be evaluated invasively with Doppler catheters that measure flow velocities (coronary flow reserve [CFR]), with pressure catheters that measure pressure gradients (fractional flow reserve [FFR]), or with both to measure stenosis resistance (resistance index).12,13 Coronary flow can be measured noninvasively using positron emission tomography (PET). Resting and hyperemic MBF are mainly dependent on myocardial oxygen demand expressed by the double-product of BP × HR, as well as other factors such as contractility, coronary driving pressure, left ventricular (LV) hypertrophy, blood viscosity, anemia, and microvascular disease (Table 15-4).5,14–17
Myocardial Blood Flow in Normal Patients
Wilson and associates first compared the effect of intravenous adenosine to intracoronary papaverine using a Doppler wire.18 In patients with normal CFR by papaverine, intravenous adenosine caused a dose-dependent increase in CFR up to a dose of 140 μg/kg/min.18 Most patients (92%) achieved near-maximal hyperemia comparable to papaverine. This compares to 50% of patients who achieved maximal vasodilatation with 0.56 mg/kg of dipyridamole infused over 4 minutes and to 84% of the subjects who received adenosine doses of 70 μg/kg/min.18 CFR was submaximal with adenosine doses of 35 and 70 μg/kg/min compared to papaverine and the 100 and 140 μg/kg/min adenosine doses (Fig. 15-1). The maximum CFR achieved with adenosine (3.4 ± 1.2) or with dipyridamole (3.1 ± 1.2) was lower than that achieved with papaverine (3.9 ± 1.1).19 The coronary vascular resistance by adenosine and papaverine was significantly lower than by dipyridamole.19 The peak flow velocity was achieved quicker with adenosine (within 55 ± 34 seconds) compared to dipyridamole (within 287 ± 101 seconds).19 In a PET study, the CFR was 4.0 ± 1.3 with dipyridamole and 4.3 ± 1.6 with adenosine, with considerable interindividual variation (range of 1.5 to 5.8 with dipyridamole; 2.0 to 8.4 with adenosine).20 The peak MBF was similar between a standard dipyridamole dose of 0.56 mg/kg (2.13 ± 0.28 mL/min/g) compared to a higher dipyridamole dose of 0.8 mg/kg (2.08 ± 0.20 mL/min/g).21
The effect of dobutamine on MBF was studied in the normal coronary arteries of 15 patients with CAD, using 13N-ammonia PET.22 The CFR increased 2.4-fold in the normal coronary arteries, corresponding to a 2.2-fold increase in the double-product.22 Dobutamine produced a lower peak MBF (2.16 ± 0.99 mL/min/g) than adenosine (3.10 ± 0.90 mL/min/g).23 Dobutamine-atropine infusion caused a greater increase in peak MBF (5.89 ± 1.58 mL/mg/min) than dipyridamole (4.33 ± 1.23 mL/mg/min), though with no difference in coronary vascular resistance.24 The 8.8-fold increase in peak MBF with dobutamine-atropine was out of proportion to the 4-fold increase in the double-product, possibly because of the atropine-mediated increase in HR.
Myocardial Blood Flow with Modified Pharmacologic Stress Protocols
The effect of 140 μg/kg/min intravenous adenosine was studied alone or in combination with supine bicycle exercise in 11 healthy volunteers using 13N-ammonia PET.25 Compared to adenosine stress alone, adenosine combined with exercise resulted in significantly higher systolic and mean BP, HR, and double-product. Adenosine combined with exercise resulted in significantly lower peak MBF (2.2 ± 0.4 mL/min/g) compared to adenosine alone (2.6 ± 0.4 mL/min/g).25 The CFR and coronary vascular resistance were also lower with the combined protocol.25 Similarly, the addition of isometric handgrip to dipyridamole caused a significant decrease rather than an increase in MBF.21
In summary, adenosine and dipyridamole cause a threefold to fivefold increase in MBF in normal coronary arteries, independent of myocardial oxygen demand (unlike dobutamine and exercise). Adenosine and dipyridamole cause a higher CFR than exercise and dobutamine.22 The hyperemic response is more predictable with adenosine than with dipyridamole and is much shorter lived.26 Addition of exercise to either adenosine or dipyridamole decreases the peak MBF compared to either alone.25
Myocardial Blood Flow in Patients with CAD
The landmark study of Gould showed that the resting MBF in dogs remained normal up to a diameter stenosis of 90%, while the CFR progressively decreased starting at 45% to 50% diameter stenosis (≈75% area stenosis).27 These results were later confirmed using PET in patients with single-vessel CAD and normal LV function.28 The resting MBF was not affected by stenosis severity, but the hyperemic MBF after intravenous adenosine progressively decreased with increasing percent diameter stenosis and decreasing minimal luminal diameter.28 The CFR started to decline at 40% diameter stenosis (≈60% area stenosis) and reached 1 at 80% diameter stenosis (≈90% area stenosis).28 Wilson et al. studied 50 patients with limited and discrete one- or two-vessel CAD using papaverine with Doppler and pressure wires.29 The CFR significantly correlated with percent area stenosis, minimal cross-sectional area, and translesional pressure gradient.29 Lesions with less than 70% area stenosis (<50% diameter stenosis) or more than 2.5 mm2 cross-sectional area had a CFR greater than 3.29
Dobutamine-induced CFR was significantly lower in regions supplied by vessels with more than 50% diameter stenoses compared to less than 50% (1.7 versus 2.3).30 A maximally tolerated dobutamine dose was compared to a standard dose of adenosine in 13 patients with CAD using PET.23 In normal segments, dobutamine caused a peak MBF of 2.16 ± 0.99 mL/min/g, which was 25% less than that achieved with adenosine (P < 0.001). In abnormal segments, dobutamine caused an MBF of 0.83 ± 0.43 mL/min/g compared to 0.90 ± 0.49 mL/min/g with adenosine (P = NS). The hyperemic response to dobutamine was in excess of that expected by the double-product and was attributed to the inotropic, oxygen-wasting, and β2 agonist effects of dobutamine. The effects of dobutamine and adenosine on MBF and CFR were compared in patients with 50% to 75% and greater than 75% diameter stenosis severity using PET.31 CFR was significantly greater with adenosine than with dobutamine stress in control subjects and remote territories not subtended by significant CAD.31 Flow heterogeneity was achieved across all coronary stenoses greater than 50% with adenosine but only in the presence of greater than 75% coronary stenoses with dobutamine.31
MYOCARDIAL BLOOD FLOW AND PERFUSION IMAGING
The conduit epicardial coronary arteries provide little resistance to flow under physiologic conditions.27 The MBF is primarily autoregulated by the arteriolar bed.27 In the presence of a stenosis in the epicardial artery, the translesional pressure drops, and the arteriolar bed progressively dilates proportional to the stenosis severity, maintaining normal flow to the myocardium.29 However, the arteriolar autoregulation and the vasodilatory reserve reach a maximum when the diameter stenosis approaches 85% to 90%, after which the coronary perfusion pressure drops to less than 45 mm Hg, along with a drop in the resting MBF.29 The ability of a stenosed artery to further augment the MBF during hyperemic conditions becomes limited because of the limited vasodilatory reserve of the arteriolar bed. The capillaries are important for exchange at the cellular level and contain 90% of the blood volume in the myocardium.32 A constant capillary pressure of 30 mm Hg is required to maintain hemostasis.32 During hyperemia of a stenosed vessel, the capillary resistance increases (derecruitment) to maintain a constant hydrostatic pressure in the face of dropping perfusion pressure and limited vasodilatory reserve.32 The hyperemic capillary resistance is directly proportional to the stenosis severity.32 The radiotracer uptake is dependent on its concentration, the MBF, and the capillary surface area for exchange.32 Thus the capillary surface area becomes an important limiting factor to radiotracer uptake. The more the derecruitment, the less the surface area available for radiotracer extraction, leading to a perfusion defect in the area supplied by a stenosed artery.32
Perfusion defects during stress MPI are generated from the disparity in MBF (or myocardial blood volume) and the differential radiotracer uptake between regions supplied by diseased compared to normal coronary arteries (Fig. 15-2).5 The regional tracer concentration also depends on the roll-off phenomenon in extraction at high rates of MBF.33 The first-pass extraction fraction of most radiotracers decreases when the flow rate reaches 2.5× the baseline value and leads to underestimation of the flow in relation to the myocardial tracer concentration.33 This roll-off effect is thought to be related to a disproportional increase in blood flow velocity compared to the increase in myocardial oxygen demand and may limit the ability of vasodilator MPI to detect mild to moderate coronary artery stenoses.5,33 The effect of the roll-off phenomenon is greater with technetium-99m tracers than thallium-201.33
The comparison of the results of Doppler flow wires to MPI has provided further insight into the generation of perfusion defects. Using dual Doppler flow wires to measure dipyridamole-induced relative CFR in normal (2.6) and diseased (1.1) coronary arteries, Voudris et al. showed a strong correlation (r = 0.90, P < 0.001) between CFR and the relative tracer uptake ratio on single-photon emission tomography (SPECT).34 In another study, a CFR less than 1.8 predicted the presence of a reversible perfusion defect on MPI with a 96% concordance rate.35 Other theories have been postulated as mechanisms for perfusion abnormalities during vasodilator stress such as decrease in distal perfusion pressure due to a decrease in BP, stenosis collapse, or coronary steal.36
PERFUSION IMAGING PROTOCOLS
Patients should be instructed to fast for 4 to 6 hours before the test to minimize nausea and vomiting.3,4 The methylxanthines competitively inhibit the adenosine receptors and may cause false-negative results.4 Current imaging guidelines recommend holding caffeinated products for 12 hours and dipyridamole, dipyridamole-containing medications (Aggrenox), or aminophylline for 24 hours prior to the test.3,4 Pentoxifylline can be continued prior to adenosine, and oral dipyridamole can be continued prior to intravenous dipyridamole.3,4 Some recommend holding antianginal medications for 24 to 48 hours prior to dobutamine and even vasodilator stress due to the ameliorating effect of these medications on perfusion defects.3,37 An intravenous line with a dual-port Y-connector for injecting the radiopharmaceutical and an infusion pump are needed for adenosine and dobutamine, whereas dipyridamole infusion does not require an infusion pump.3,4 Continuous electrocardiographic (ECG) monitoring and BP recordings at 1-minute intervals are recommended.4 The contraindications and endpoints of pharmacologic MPI are listed in Tables 15-5 and 15-6.
Table 15-5 Contraindications to Pharmacologic Stress Testing
Contraindications to Dipyridamole or Adenosine |
AV, atrioventricular; MI, myocardial infarction.
Reprinted from Zoghbi G, Iskandrian AE: Coronary artery disease detection: Pharmacologic stress. Zaret BL, Beller GA, eds. Clinical Nuclear Cardiology: State of the Art and Future Directions, 3rd ed. Philiadelphia: Elsevier Mosby, 2005, pp 233–253 with permission from Elsevier.
Table 15-6 Endpoints in Pharmacologic Stress Testing
With Dipyridamole or Adenosine |
With Dobutamine |
Reprinted from Zoghbi G, Iskandrian AE: Coronary artery disease detection: Pharmacologic stress. Zaret BL, Beller GA, eds. Clinical Nuclear Cardiology: State of the Art and Future Directions, 3rd ed. Philiadelphia: Elsevier Mosby, 2005, pp 233–253 with permission from Elsevier.
The acquisition protocols with the different stressors and radiotracers used (thallium, sestamibi, and tetrofosmin) are shown in Figure 15-3. The protocols used with technetium-labeled agents can be same-day stress/rest or rest/stress, 2-day stress/rest, or dual isotope.3,4,38,39 The thallium protocol is stress/4-hour redistribution/reinjection. The images are acquired 10 minutes after thallium injection and 40 to 60 minutes after sestamibi or tetrofosmin injection.3,4,38
Adenosine is marketed as Adenoscan and is infused intravenously at a rate of 140 μg/kg/min over 5 to 6 minutes.4,37,38 A 3- or 4-minute infusion is used in some laboratories.4,37,38 The radiotracer is injected during the third minute of the infusion.4 A modified adenosine protocol can be used in higher-risk patients such as those with history of hyperactive airway disease, liver cirrhosis with massive ascites, recent ischemic events, or low systolic BP.3,4,37,38 The modified protocol starts at a rate of 50 μg/kg/min for 1 minute followed by 75, 100, and 140 μg/kg/min at 1-minute intervals if tolerated.3,4,37,38 Low-level upright treadmill exercise at 1.7 mph and 0% grade during adenosine infusion can be used in patients without LBBB or a permanent pacemaker.3,4,37,38
Dipyridamole is injected intravenously at a dose of 0.56 mg/kg over a 4-minute period.3,4,37,38 The radioactive tracer is injected 3 to 5 minutes after termination of the dipyridamole infusion.3,4,37,38 Low-level upright treadmill exercise at 1.7 mph and 0% grade for 4 to 6 minutes shortly after completion of the dipyridamole infusion can be used in patients without a LBBB or permanent pacemaker.3,4,37,38 The radiotracer is injected during the low-level exercise, which should be continued for 2 minutes after radiotracer injection.3,4,37,38 A 50% higher dose of dipyridamole is sometimes used in Europe, but it is not certain whether the higher dose produces more coronary hyperemia.21
Dobutamine is administered intravenously at an initial dose of 5 to 10 μg/kg/min and is augmented by 10 μg/kg/min every 3 minutes until a maximum dose of 40 μg/kg/min is reached, target HR is achieved, or symptoms develop.4,37,38 Atropine in 0.5- to 1-mg increments (up to 2 mg total) is administered intravenously if the peak HR is not reached with a maximal dobutamine dose.3,40 Atropine is contraindicated in patients with myasthenia gravis, obstructive gastrointestinal tract, narrow-angle glaucoma, or uropathy.3,40 The radioactive tracer is administered at peak HR, and the dobutamine infusion is continued for an additional 1 minute (see Fig. 15-3).40
SAFETY AND SIDE EFFECTS
Minor side effects occur more commonly with adenosine than with dipyridamole; however, they are short lived and better tolerated and rarely require reversal with theophylline.4 Around 80% of patients developed minor symptoms during adenosine infusion and 50% during dipyridamole infusion.4,6,41,42 Clinically significant side effects such as severe chest pain, hypotension, and bronchospasm occurred in 1.6% of patients receiving adenosine.4,6,41,42 The most common side effects of adenosine and dipyridamole are listed in Table 15-7. Adenosine was better tolerated in men than women (except for flushing) and in older patients than younger patients, and was preferred over dipyridamole in the patients who received both stressors on separate days.43,44 Reversal of side effects with theophylline was required in 12% of patients receiving dipyridamole but in fewer than 1% of patients receiving adenosine.4 Chest pains during vasodilator stress are due to stimulation of the adenosine A1 receptor and thus could occur in patients with normal coronary arteries.7,45 Dyspnea during vasodilator stress is rarely due to bronchospasm or pulmonary edema and more commonly due to carotid chemoreceptor stimulation that increases the depth and rate of respiration (tachypnea).46 Adenosine and dipyridamole are well tolerated in smokers and in patients with compensated nonreactive airway disease.38,41,46 To prevent bronchospasm during stress testing, prophylactic administration of inhaled β agonists before adenosine or dipyridamole infusions has been used in patients with moderate to severe obstructive lung disease.47
Atrioventricular (AV) blocks occur in 2% of patients undergoing dipyridamole stress and in 7.6% of patients undergoing adenosine stress and are usually intermittent, transient, well tolerated, may resolve even if the adenosine infusion is continued, and are not reasons to terminate the infusion.3,37 Second- and third-degree AV blocks occurred in 4% and less than 1% of patients undergoing adenosine stress.48 In patients with first-degree AV block at baseline undergoing adenosine stress, second- and third-degree AV blocks occurred transiently in 37% and 14%, respectively, did not require any treatment, and usually resolved with decreasing or discontinuing the adenosine infusion.37,48 Concomitant use of AV blocking agents with adenosine or increasing age did not influence the incidence of AV blocks.48,49 AV block during adenosine infusion usually occurs in the first 2 to 3 minutes of the infusion, follows the hyperemic response, and does not require termination of the infusion in over 95% of cases.3,37 The radiotracer can be injected when the AV block occurs while the adenosine dose is subsequently down-titrated.3 Adenosine testing has been shown to be safe in elderly patients and in patients with significant aortic stenosis.49,50
Severe side effects with vasodilator stress agents occur rarely. In two large multicenter safety trials, the respective incidences of death and nonfatal myocardial infarction (MI) were 0 and 1/10,000 with adenosine and 1/10000 and 1.8/10,000 with dipyridamole.41,51 Severe side effects, especially bronchospasm (incidence of 8/10000 with adenosine), can be promptly reversed with 50 to 100 mg of intravenous theophylline injected over 1 minute and repeated to a total dose of 250 to 300 mg.3,37 Theophylline competitively inhibits the adenosine receptor, and its injection should be delayed for 1 to 2 minutes after tracer injection, if possible, to ensure adequate tracer uptake.3 An exaggerated hypotensive response with adenosine stress may occur in patients with severe liver disease who are being evaluated for transplant surgery. The hypotension is likely due to overdosing, as the dry weight in these patients may be only 50% of their total weight because they often have massive ascites. A titration adenosine protocol is more prudent in these patients.
Side effects occur in around 75% of patients undergoing dobutamine stress.52,53 The most common side effects are chest pains, palpitations, flushing, dyspnea, and arrhythmias (see Table 15-7).54 Chest pain during dobutamine stress testing is not a predictor of ischemia as defined by reversible perfusion defects on SPECT imaging.55 Premature ventricular complexes and nonsustained ventricular tachycardia occurred in 12% and 4.2% of patients who received dobutamine and in 31% and 6.3% of patients who received dobutamine-atropine.10,53 Ventricular arrhythmias were more frequent in patients with LV dysfunction, fixed perfusion defects, resting wall-motion abnormalities, and history of prior ventricular arrhythmias.10,11,40,53,54 In a dobutamine safety study that involved 1012 patients, there were no deaths, MIs, or malignant arrhythmias.53 The side effects of dobutamine can be reversed by a short-acting β-blocker such as esmolol.4 We believe that dobutamine should not be used shortly after acute MI and possibly also in patients with large aortic abdominal aneurysm and those with atrial fibrillation or history of serious ventricular arrhythmias.
HEMODYNAMIC EFFECTS
Vasodilators
Adenosine and dipyridamole cause modest decreases in systolic, diastolic, and mean BP and a modest increase in HR, with a greater effect observed with adenosine than dipyridamole.38 Dipyridamole increased the HR by 11 ± 7 beats/min, decreased the mean BP by 10 ± 3 mm Hg, and increased the cardiac output by 34%.56 Adenosine increased the HR by 14 to 17 beats/min and decreased the systolic BP by 10 to 18 mm Hg and the diastolic BP by 8-9 mm Hg.41 In patients undergoing adenosine stress, the HR increased in 94% of patients, the systolic BP decreased in 85% of patients, and the diastolic BP decreased in 80% of patients.6 The HR response to adenosine infusion was diminished in patients with diabetes mellitus (DM) and normal perfusion on SPECT imaging, most likely due to diabetes induced cardiovascular autonomic neuropathy.57 The systolic BP decreased transiently to less than 80 mm Hg in 2.5% of patients and increased paradoxically in 13% of patients.6 The pulmonary capillary wedge pressure slightly increased in normal subjects and more so in patients with CAD.41 The decrease in BP is due to a drop in the systemic vascular resistance. The increase in the pulmonary capillary wedge pressure is due to an increase in venous return and preload, increased diastolic stiffness, and coronary turgor in normal patients, and also due to ischemia-induced diastolic and systolic LV dysfunction in patients with CAD.36 The increase in cardiac output is primarily due to an increase in HR.36 Adenosine and dipyridamole produce their coronary vasodilatory effects independent from their peripheral hemodynamic effects. Thus, CAD detection accuracy is independent of the vasodilator peripheral hemodynamic changes.58,59
Dobutamine
The hemodynamic effects of dobutamine are comparable to those observed with submaximal exercise.3,10 A 40 μg/kg/min dobutamine dose increased the systolic BP by 27 mm Hg and the HR by 45 beats/min and decreased the diastolic BP by 17 mm Hg.3,60 Incremental dobutamine doses progressively increased the HR while the BP increased and leveled at a dose of 20 μg/kg/min.3,60 Hypotension during dobutamine stress, defined as ≥ 20 mm Hg drop in BP from baseline, occurred in 14% to 20% of patients and is due to peripheral vasodilatation, not to ischemia.3,11 As such it is not predictive of the presence or extent of wall-motion abnormality or LV dysfunction and has no prognostic implications as seen with exercise-induced hypotension.3,11 The hypotensive response occurs more commonly in patients with advanced age, high baseline systolic BP, dynamic LV outflow obstruction, and small hyperdynamic LV.3,10,11 In one study, however, dobutamine-induced hypotension correlated with the number of ischemic segments on perfusion imaging in patients with previous MI.10 Dobutamine-related sinus node deceleration is defined as an initial increase followed by a decrease in HR and occurs in 7% to 19% of patients.3,10,11 It results from activation of cardioinhibitory receptors that activate the Bezold-Jarisch reflex. 10,11
ISCHEMIC RESPONSE
Vasodilators
Adenosine or dipyridamole can cause ischemia by producing coronary steal that could be collateral dependent or transmural. Collateral-dependent steal results from decrease in collateral flow distal to a stenosed artery because of differential flow decrease in the donor vessel.61,62 Transmural steal is less common and less severe and occurs from the subendocardial to subepicardial region, owing to the difference in residual vasodilatory reserve in the subendocardium and subepicardium.61–63 Systemic hypotension that occurs due to systemic vasodilatation exacerbates the steal phenomenon.62 Markers of ischemia are ST-segment depressions, typical angina pectoris, and regional wall-motion abnormalities.61 Most perfusion defects, however, are not due to ischemia but rather to disparity in regional blood flow as a result of variations in hemodynamic severity of coronary stenoses.
Ischemic ST-segment changes due to vasodilator stress are usually depression and rarely elevation. Ischemic changes occurred in 15% to 40% of patients with CAD undergoing dipyridamole stress and in less than 10% of an unselected population.64,65 ST depressions occurred in 7.6% of 959 patients who underwent adenosine stress and were more common in women (64%) compared to men (36%).66 Adenosine-induced ischemic ST-segment changes have been correlated with higher baseline and peak systolic BP, greater increase in BP and HR, occurrence of typical angina during adenosine infusion, extensive CAD, presence of collaterals on coronary angiography, extensive and severe perfusion abnormalities, and transient ischemic dilation (TID) (Fig. 15-4).66–68 ST depressions occur less commonly than perfusion defects but have a specificity of 90% for CAD detection.68 Adenosine-induced ischemic ECG changes with normal perfusion on MPI occurred in 1% to 2% of patients, of whom 80% to 88% were women.69–71 A high event rate was reported in these patients in two studies but was not supported by another study from our own group.69–71
Dobutamine
ST-segment depressions occurred in up to 50% of patients with CAD undergoing dobutamine and had a 60% sensitivity and a 90% specificity.10,11 ST-segment depressions were significantly related to the dobutamine dose and to the CAD extent.10,11 ST-segment elevations occurred in 6% of patients and as such are markers of extensive perfusion abnormalities, severe CAD, and depressed LV function.10,11
LUNG THALLIUM UPTAKE
Increased thallium uptake, defined as a lung-to-heart ratio greater than 50%, is thought to occur because of increased LV filling pressure.3 Increased lung thallium uptake was reported in 22% to 38% of patients who underwent dipyridamole-thallium imaging and in 30% to 52% of patients who underwent adenosine-thallium imaging.72 Increased thallium uptake correlates with LV cavity dilation, poor LV function, prior MI, the extent and severity of ischemia, and multivessel disease and collaterals on coronary angiography.56,65,73 Although increased lung thallium uptake and TID are both associated with severe and extensive CAD, there was no significant correlation between the two indices during dipyridamole-thallium MPI, signifying different pathophysiologic processes.74 An increased lung-to-heart ratio has also been described with technetium tracers, but the absolute values are lower than with thallium.73
TRANSIENT ISCHEMIC DILATATION
Transient ischemic dilation is defined as the quantitative or visual increase in LV cavity size on the poststress images compared to the resting images.3,75 TID results from subendocardial hypoperfusion or rarely from a true ischemia-mediated increase in LV end-diastolic volume.65,76 TID occurs in up to a third of patients undergoing adenosine stress and in up to a fourth of patients undergoing dipyridamole stress.65,76 The presence of TID has been associated with the presence of extensive and severe CAD on angiography, presence of collaterals, extensive and severe perfusion abnormalities on MPI, lower poststress LV function, and more wall-motion abnormalities.77–80 In patients without prior MI who underwent dipyridamole MPI, the sensitivity and specificity of TID to detect severe multivessel CAD were 80% and 92%, respectively.81 The accuracy of TID for detecting left main (LM) or severe three-vessel disease during adenosine MPI was 81%.82 TID can occur in the absence of CAD, as a result of subendocardial ischemia (seen in patients with hypertensive heart disease or hypertrophic cardiomyopathy) or due to technical factors.75
POSTSTRESS LEFT VENTRICULAR DYSFUNCTION
Poststress LV dysfunction is defined as a poststress LV ejection fraction (EF) drop by more than 5% compared to rest.83,84 Poststress stunning is defined as poststress worsening or new regional wall-motion or thickening abnormalities compared to rest.83,84 Poststress LV dysfunction occurred in 33% of patients with CAD who underwent adenosine MPI and in 25% of patients who underwent dipyridamole MPI.83,85,86 Poststress LV dysfunction with dipyridamole stress had a sensitivity and specificity of 35% and 93%, respectively, for the detection of severe CAD.86 Poststress LV dysfunction has been correlated with abnormal segmental wall thickening, TID, large ischemic defects, end-systolic dilation and extensive CAD on coronary angiography.83,86,87 Poststress LV dysfunction or wall motion abnormalities are thought to result from ischemia-induced stunning. A recent study suggested that poststress LV stunning resulted from artifacts of low count regions, since there was an overestimation of post-ischemic stunning on gated SPECT imaging compared to simultaneous poststress ECG.84 Alternatively, poststress stunning might be more frequent if imaging is performed earlier, as suggested by PET experience. In patients with normal stress SPECT imaging, ATP stress or exercise stress had differential effects on LV volumes that were measured more than 30 minutes after stress, implying differential effects of stress methods on LV volumes.88 In another study of patients with normal stress SPECT, adenosine stress resulted in a significant decrease in stress LVEF, while exercise stress resulted in a significant increase in stress LVEF compared to rest LVEF.89
DIAGNOSIS OF CORONARY ARTERY DISEASE
The accuracy of CAD diagnosis by stress MPI depends on several factors such as pretest and posttest referral biases, patient selection, definition of CAD and perfusion abnormalities, endpoints attained with dobutamine, use of attenuation correction and gating, radiotracer type, and the experience of the reader.3 Most of the studies compared the results of MPI to coronary angiography, considered the gold standard. However, various studies using PET and pressure-based or Doppler-based catheter techniques have shown considerable variability between coronary angiography and the physiologic significance of coronary lesions (Fig. 15-5).3,13,90 Even in patients with nonsevere lesions on coronary angiography and normal FFR, the presence of reversible perfusion defects was associated with a higher lesion plaque burden detected by intravascular ultrasound.91
Vasodilators
Most of the intravenous dipyridamole MPI studies were performed using planar thallium imaging and used qualitative analysis without gating and attenuation correction. The average sensitivity and specificity of planar thallium dipyridamole MPI were 82% and 75%, respectively, while those of dipyridamole-thallium SPECT were 89% and 78%, respectively.92 The mean sensitivities and specificities of vasodilator SPECT for detecting CAD (≥50% stenosis) without correction for referral bias were 86% and 73%, respectively (Fig. 15-6).92 In a study designed without referral bias, the sensitivity and specificity were 72% with pharmacologic SPECT compared to 78% and 85% with exercise SPECT, respectively.93 The results of dipyridamole and adenosine MPI with the different radiotracers are shown in Figure 15-6. The results of a meta-analysis of adenosine and dipyridamole SPECT for detecting CAD and multivessel CAD are shown in Figure 15-7.94 The normalcy rate of adenosine-sestamibi performed in patients with low likelihood of CAD was 90%.94 The sensitivity of adenosine-thallium SPECT for the detection of one-vessel, two-vessel and three-vessel CAD was 83%, 91%, and 97%, respectively.56 The respective sensitivities and specificities of adenosine thallium SPECT to detect over 50% stenosis were 60% and 90% for the left circumflex (LCX) artery, 75% and 96% for the right coronary artery (RCA), and 75% and 97% for the left anterior descending (LAD) artery, with an accuracy of 75% for the LCX, 86% for the RCA, and 85% for the LAD arteries.6 Thallium and sestamibi-dipyridamole SPECT performed in the same patients had similar sensitivities of 100% and specificities of 75% for CAD detection.95 Sestamibi had a higher sensitivity compared with tetrofosmin; however, the diagnostic accuracy and image quality were not different between the two tracers.96
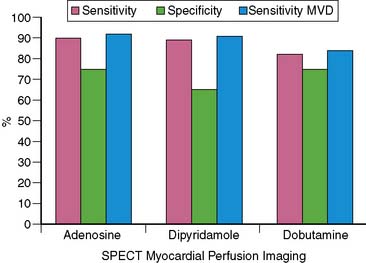
Figure 15-7 Weighted sensitivities of pharmacologic stress SPECT for detecting multivessel disease (MVD).
(From Zoghbi G, Iskandrian AE: Pharmacologic stress testing. In Garcia EV, Iskandrian AE [eds]: Nuclear Cardiac Imaging: Principles and Applications. New York: Oxford University Press, 2008, pp 293-315.)
The use of gating, attenuation correction, and imaging in the prone position have improved the specificity of SPECT imaging without overall change in sensitivity.92,97,98 Figure 15-8 shows the effects of attenuation correction and gating on the accuracy of adenosine SPECT.
Dobutamine
Most patients undergoing dobutamine stress tend to be sicker patients, resulting in an important selection bias. Dobutamine also directly affects sestamibi kinetics by affecting mitochondrial potentials, resulting in a lower tracer concentration at a given level of MBF compared to adenosine.10 In a meta-analysis of 20 studies involving 1014 patients who underwent dobutamine SPECT, the weighted sensitivity, specificity, and accuracy for CAD detection were 88%, 74%, and 84%, respectively.11 The sensitivity was 82% in studies that did not use atropine and was 90% in studies that used a maximum dobutamine dose with atropine.11 The sensitivity of dobutamine SPECT to detect one-, two-, and three-vessel disease 84%, 95%, and 100%, respectively.11 The mean respective sensitivities and specificities to detect significant CAD were 50% and 94% for the LCX, 88% and 81% for the RCA, and 68% and 90% for the LAD arteries.11
Comparison of Pharmacologic Myocardial Perfusion Imaging
In a meta-analysis of 44 pharmacologic SPECT studies, the respective sensitivities and specificities for adenosine, dipyridamole, and dobutamine were 90% and 75%, 89% and 65%, and 82% and 75%.94 In a study of 54 patients that compared adenosine and dipyridamole, adenosine produced more but shorter-lived side effects (83% versus 65%), a greater decrease in systolic BP (−12 versus −5 mm Hg), a greater increase in HR (+18 versus +8 beats/min), and more reversible defects.44 The sensitivity for CAD detection was 87% with dipyridamole and 91% with adenosine.44 Dobutamine and vasodilator SPECT were compared in the same 157 patients.11 The respective sensitivity and specificity for CAD detection were 77% and 78% with dobutamine compared to 90% and 85% with vasodilator SPECT.11
Adenosine, dobutamine, and arbutamine were evaluated in 40 patients undergoing tetrofosmin MPI.99 There was a significant agreement for semiquantitative and visual segmental analysis among the three stressors despite differences in hemodynamic and side-effect profiles.99
Comparison to Exercise
The results of vasodilator SPECT are comparable to those of maximal exercise SPECT and at least better than those of submaximal exercise SPECT. In two different multicenter trials involving 244 patients, the average sensitivity, specificity, and accuracy for CAD detection were 83%, 81%, and 82% with adenosine MPI and 82%, 77%, and 79% with exercise MPI, respectively.100,101 In another study of 175 patients who performed exercise and adenosine MPI within 1 month, the visual and quantitative agreements between exercise and adenosine results were 83% and 86%, respectively.102 The defect sizes were greater with adenosine, especially when the exercise stress was submaximal.102 Bicycle exercise SPECT and adenosine were performed in the same 22 patients and showed a 100% agreement on the presence of an abnormal study and a 90% concordance rate in identifying abnormal segments.103 Dipyridamole and exercise SPECT were performed in the same 16 patients, with myocardial bridging of the LAD by coronary angiography.104 The prevalence of perfusion defects was similar between the two stressors, though there was a trend toward higher perfusion scores with exercise.104 Dipyridamole and treadmill exercise N-13 ammonia PET MPI were performed in the same 26 patients.105 The summed difference score (SDS), summed stress score (SSS), and total perfusion defect size were significantly larger with exercise stress compared to dipyridamole stress.105 Dipyridamole, adenosine, and exercise sestamibi MPI were compared in the same 20 subjects and were found to have comparable defect sizes and severity.106 Dipyridamole, adenosine, dobutamine, and exercise tetrofosmin MPI were compared in the same 38 patients.107 Dipyridamole and dobutamine produced less defect severity, extent, and reversibility compared to exercise and adenosine.107
In summary, dipyridamole and adenosine have similar sensitivities for CAD detection as with exercise, though adenosine has a slightly higher specificity.26 Table 15-8 shows the appropriate selection of a specific stress modality.
Condition | Test of Choice |
---|---|
If patient can achieve a good exercise | ![]() |
If patient has exercise limitations | ![]() |
If patient has bronchospasm | ![]() |
If patient has LBBB/pacemaker | ![]() |
If patient is < 72 hours of acute MI | ![]() |
If patient is < 24 hours of PCI | ![]() |
If patient has a large AAA | ![]() |
AAA, abdominal aortic aneurysm; LBBB, left bundle branch block; MI, myocardial infarction, PCI, percutaneous coronary intervention; TET, treadmill exercise test.
Results in Women
Most of the stress SPECT studies were performed in men. Women usually present at an older age, attain lower exercise endpoints, and undergo pharmacologic stress rather than exercise stress.108 The diagnostic accuracy of stress SPECT in women is affected by the presence of smaller LV size and myocardial mass, and breast attenuation.109 The diagnostic accuracy of CAD detection in women has improved with the use of vasodilator stress, higher- energy tracers, attenuation correction, and gating.110
Adenosine SPECT in women had a sensitivity, specificity, and positive predictive value of 93%, 78%, and 88%, respectively, and a normalcy rate of 93% for detecting ≥ 50% diameter stenosis.111 Adenosine SPECT had a higher sensitivity for CAD detection in men compared to women (94% versus 84%).112 Adenosine and exercise had similar sensitivity for multivessel disease detection in men and women, but the sensitivity of adenosine was higher than that of exercise in women with one-vessel disease.112 Women who underwent dual-isotope adenosine SPECT had significantly smaller stress, rest, and reversible defects than had men.97 In women who underwent stress SPECT with thallium and sestamibi, the respective sensitivity and specificity for detecting greater than 70% stenosis were 80% and 84% with sestamibi and 84% and 67% with thallium.113 The specificity of sestamibi imaging improved to 94% with the addition of gating, which was not used in the thallium images.113 Exercise or dobutamine tetrofosmin SPECT had a sensitivity, specificity, and accuracy of 83%, 80%, and 82%, respectively, to detect greater than 50% CAD in women.109 The sensitivity was 72% for single-vessel CAD and 93% for multivessel CAD.109
Detection of Left Main or Three-Vessel Disease
Left main (LM) or three-vessel disease has no specific pathognomonic perfusion abnormality pattern. Abnormal perfusion in more than one vascular territory is seen in 30% to 70% of patients with LM or three-vessel disease, and rarely no perfusion abnormalities are detected.112,114 The use of tracers with better extraction fraction and newer quantitative techniques that assess perfusion reserve ratio may improve the ability to more reliably detect individual diseased vessels and eliminate false-negative results. Stepwise discriminant analysis identified multivessel thallium abnormality, increased lung thallium uptake, and ST depression as predictors of LM or three-vessel disease in patients who underwent adenosine-thallium SPECT.114 A model consisting of magnitude of ST depression, summed reversibility score, and increased lung uptake had incremental power over clinical variables to detect severe LM or three-vessel disease.115 Severe LM or three-vessel disease was present in the 57% of the predicted high-risk patients and in 9% of the predicted low-risk patients.115 The SSS and the prescan likelihood of CAD were the independent multivariate predictors of severe LM or multivessel CAD in women who underwent adenosine SPECT.116 A SSS of greater than 8 had a sensitivity of 91% and a specificity of 70% for identifying women with severe CAD.116 In a study of 101 patients with greater than 50% LM CAD who underwent gated exercise or adenosine stress sestamibi SPECT MPI, moderate to severe defects (>10% myocardium at stress) were identified in only 56% of patients visually and 59% quantitatively.117 Non-significant perfusion defects (<5% myocardium) were seen in 13% of patients visually and 15% quantitatively.117 Combining perfusion and nonperfusion abnormalities, especially TID, identified 83% of patients as high risk.
HYBRID PROTOCOLS
Abbreviated Adenosine Protocols
Abbreviated adenosine infusion times have been used in some laboratories to reduce adenosine side effects and costs. Studies that compared a 3-minute to a 6-minute adenosine protocol showed less AV block, hypotension, tachycardia, and other side effects in the 3-minute group.118,119 Dysrhythmias occurred within 2 minutes from initiation of the 3-minute and 6-minute infusions.118 The sensitivity for CAD detection was similar between the two groups, although perfusion defect size was slightly smaller in the 3-minute group in one study, and the redistribution was higher in the 6-minute group in another study.118,119
In a study that compared a 4-minute to a 6-minute adenosine infusion protocol, side effects occurred in a similar frequency in both groups but were of shorter duration in the 4-minute group.120 Premature discontinuation of the infusion was more common in the 6-minute group.120 Ischemic ST changes and chest discomfort occurred less frequently in the 4-minute group, although the accuracies for CAD detection were similar between the two groups.120 In a study that compared a 4-minute adenosine infusion in conjunction with low-level treadmill exercise to a 6-minute adenosine infusion protocol, the 4-minute protocol with exercise had better image quality and fewer side effects.121
Vasodilators and Exercise
Adenosine or dipyridamole stress has been combined with handgrip, submaximal, or maximal exercise during or shortly after the vasodilator infusion but before tracer injection.4 The reported benefits of the combination protocols include an increase in the double-product and the incidence of ischemic ECG changes, improved image quality, improved target-to-background ratio (decreased hepatic activity), reduced hypotension and other side effects, and a trend to produce more ischemic defects.122–128 As discussed earlier, the addition of exercise did not increase MBF above that achieved with dipyridamole or adenosine alone.21
Modified Dobutamine Protocols
Dobutamine was used in combination with dipyridamole as an additional 10 and 20 μg/kg/min each for 3 minutes at the end of the 4-minute 0.56 mg/kg dipyridamole infusion.129 The systolic BP, double-product, summed stress and reversibility scores, ECG changes, angina, and abnormal perfusion images were greater in the combination protocol compared to the dipyridamole-only protocol.129 Side effects were similar between the two groups.129
Patients who fail to attain their target HR by the end of the 40 μg/kg/min dobutamine infusion are routinely given 0.5 to 1.0 mg of atropine.4 The atropine-dobutamine regimen caused 94% of patients to achieve target HR or an ischemic endpoint.54 The sensitivity of CAD detection improved from 82% with dobutamine alone to 90% with atropine-dobutamine.11
Low-level exercise supplementation for the last 2 minutes of a dobutamine infusion caused significantly higher heart-to-liver and heart-to-diaphragm thallium uptake ratios, with resultant improved image quality and no differences in the occurrence of side effects or ECG changes.130 A leg-raising protocol was used in patients who did not reach their target HR by the end of the 30 μg/kg/min, and involved alternating leg raising to 45 degrees up to 3 minutes as tolerated while dobutamine was increased and maintained at 40 μg/kg/min.131 Compared to the conventional dobutamine with atropine as-needed protocol, the leg-raising protocol caused significantly higher peak HR and double-product, lower time to reach peak HR, fewer side effects including arrhythmias, and 100% attainment in target HR.131 Combination of handgrip exercise with dobutamine compared to dobutamine alone reduced the total dose of dobutamine required to achieve target HR and decreased the side effects associated with dobutamine.132 Target HR was achieved in 97% of the patients in the combination protocol and in 82% of the dobutamine-alone protocol.132 One study compared a standard progressive dobutamine protocol with increments of 10 μg/kg/min and atropine at the end of the 40 μg/kg/min infusion if target HR is not reached, to an accelerated protocol where atropine was administered at the end of the first stage of a progressive dobutamine protocol aiming at the same HR of the standard protocol.133 The incidence of adverse effects was reduced in the accelerated (34.5%) compared to the standard protocol (54.8%; P < 0.05), as well as the dobutamine infusion duration (508 ± 130 versus 715 ± 142 sec; P < 0.001).133 The maximal HR, percent of achieved maximal HR, rate-pressure product, ST changes, and perfusion scores were similar in the two groups.133
SPECIAL PATIENTS
Patients With Left Bundle Branch Block
Patients with LBBB can develop false anteroseptal ischemic perfusion defects on dobutamine or exercise SPECT in the absence of disease in the LAD territory.134 Doppler flow wire studies in patients with LBBB and normal coronary arteries demonstrated a reduction in diastolic MBF in the LAD due to early diastolic compressive resistance resulting from the asynchronous and delayed systolic septal contraction.134 The decreased diastolic MBF is further exacerbated during exercise by the tachycardia-induced reduction in the diastolic filling time.134 False-positive perfusion abnormalities can occur in up to 50% of patients undergoing exercise SPECT and in 84% to 100% of patients undergoing dobutamine SPECT.10,135
In a study that compared exercise and adenosine thallium MPI in patients with LBBB, the specificity for detecting greater than 50% diameter stenosis in the LAD was 82% with adenosine and 42% with exercise.135 Adenosine-tetrofosmin SPECT and coronary angiography were performed in patients with chest pain and LBBB.136 A significant angiographic LAD disease was found in 25% of patients. The sensitivity and the specificity of adenosine SPECT were 75% and 89%, respectively, and the positive and negative predictive values were 70% and 91%, respectively.136 The use of iterative reconstruction and attenuation correction in patients with spontaneous or pacemaker-induced LBBB resulted in more pronounced and less reversible apical-septal defect patterns compared with filtered projection.137
Vasodilators are the stressors of choice for patients with LBBB, and neither should be combined with exercise or dobutamine.4 Perfusion defects in the RCA or LCX territory are predictive of disease in these vessels regardless of the stress modality.3 Additionally, a normal perfusion study is meaningful regardless of stress modality.3 Finally, anteroseptal fixed defects are predictors of prior MI and are not affected by stress modality.3 Gated SPECT imaging in patients with LBBB may show septal wall motion abnormality despite normal perfusion, but the thickening is often normal.3
Patients with Permanent Ventricular Pacing
Permanent ventricular pacing, like LBBB, may produce false-positive reversible perfusion defects in the septum and also in the inferior wall and apex in the absence of coronary disease.138,139 These perfusion defects are thought to arise from the asynchronous ventricular activation of pacing that alters regional perfusion and function.139 Patients with right ventricular pacing who underwent exercise thallium MPI and coronary angiography developed false-positive perfusion defects in the inferoposterior (71%), apical (50%), and inferoseptal walls (28%).140 In another study of patients with pacemakers who underwent exercise SPECT, false-positive perfusion defects occurred in 58% of patients and were associated with longer durations of pacing, apical wall motion abnormalities, and lower LVEF.138 As with LBBB, it is recommended to use vasodilator stress in patients with pacemakers.4
Patients with Left Ventricular Hypertrophy or Cardiomyopathy
LV septal hypertrophy observed in some patients with end-stage renal disease, hypertension, or hypertrophic cardiomyopathy may downscale the lateral wall, mimicking a lateral wall perfusion abnormality due to lower lateral-to-septal count density ratio.141 A racial difference in CFR and perfusion defects on dipyridamole-thallium MPI was demonstrated between whites and blacks who had normal coronary arteries and LV hypertrophy on echocardiography.142 In blacks, the CFR decreased significantly as the severity of LV hypertrophy increased, whereas in whites the CFR and the frequency of perfusion defects did not significantly differ in the presence or absence of LV hypertrophy. The frequency of abnormal scans in blacks was 59% in those with LV hypertrophy and 31% in those without hypertrophy.142 Exercise SPECT in patients with LV hypertrophy on echocardiography had a sensitivity and a specificity of 84% and 82%, respectively.143 The sensitivity, specificity, and accuracy for CAD detection were similar between hypertensive patients with and without LV hypertrophy and between hypertensive and normotensive patients who underwent exercise SPECT.144 LV hypertrophy should not affect the results of perfusion imaging, as long as the “hot spot” artifact is recognized as a normal variant.
Patients with hypertrophic, ischemic, or nonischemic cardiomyopathies may develop reversible or fixed perfusion in the septum or elsewhere, even in the absence of CAD.145 These defects are likely due to supply-demand imbalances, metabolic abnormalities, MBF regional differences, and microscarring.145 The relationship between the extent of adenosine-tetrofosmin SPECT defect and the presence of significant CAD as cause of cardiomyopathy was statistically significant in patients with LVEF between 35% and 50% but not significant in those with LVEF ≤ 35%.146
EFFECT OF ANTI-ISCHEMIA MEDICATIONS
The effect of anti-ischemia medications on stress SPECT has been studied in patients on therapy compared to patients without therapy (parallel studies) or in the same patients before and after treatment (serial studies).147 The lipid-lowering and anti-angina medications such as calcium channel blockers, β-blockers, and nitrates have been shown to improve perfusion abnormalities in some studies.147 β-blockers and some of the calcium channel blockers attenuate the double-product of dobutamine or exercise stress.3 Calcium channel blockers and nitrates dilate the epicardial, resistive, and collateral circulation and decrease the epicardial-to-endocardial flow ratio and the heterogeneity of MBF.3 These effects are thought to attenuate the effects of vasodilators. Additionally, nitrates decrease preload, afterload, and oxygen demand and subsequently improve subendocardial flow.3,148 β-blockers improve the vasodilatory capacity of coronary arteries by decreasing the extravascular compressive forces and improving diastolic relaxation.149
Intravenous propranolol administration before dobutamine SPECT caused lower peak HR and BP despite infusing higher doses of dobutamine.150 The perfusion defects were smaller after propranolol infusion, and myocardial ischemia was abolished in 23% of patients.150 The accuracy of dobutamine MPI was not altered when atropine was given to augment the submaximal chronotropic response.150
The effects of anti-ischemia medications on vasodilator stress MPI have been variable. Treatment with atenolol or placebo prior to dipyridamole MPI did not cause a difference in the overall perfusion results between the groups.151 However, the sizes of the perfusion defects were larger in 25% of patients after atenolol.151 Acute administration of metoprolol before dipyridamole SPECT decreased the sensitivity of CAD detection from 86% to 71% and reduced the extent and severity of ischemia by 25% to 30%.152 In a retrospective study of patients who underwent adenosine SPECT while on β-blockers, the extent, severity, and reversibility of perfusion defects were not significantly different from a group of patients with gender, age, pretest symptoms, and history of CAD who underwent adenosine SPECT while off β-blockers.153 The effects of amlodipine, nitroglycerin, and metoprolol were studied in patients with CAD (>70% stenosis) who randomly underwent dipyridamole MPI PET while on and off these medications (>5 half-lives).154 Amlodipine did not have a significant effect on resting or dipyridamole-induced hyperemic MBF. Nitroglycerin increased the resting MBF, and metoprolol decreased the resting and hyperemic MBF.154 The effects of nitrates, calcium channel blockers, or both were studied in patients who underwent dipyridamole with low-level exercise MPI with and without these medications.148 Anti-angina medication use before dipyridamole-exercise MPI decreased the defect size by 30%, decreased individual vessel sensitivity from 92% to 62% (especially LAD and LCX) with no effect on specificity and had no hemodynamic effects.148
In patients who underwent adenosine 13N-ammonia PET, pretreatment with β-blockers for 12 weeks significantly improved the hyperemic MBF in stenosis-dependent segments to a similar level in stenosis-independent segments.149 In patients with CAD who underwent adenosine and exercise SPECT on two different occasions, treatment with β-blockers caused less severe and less extensive reversible perfusion defects with exercise stress compared with adenosine stress.155
Lipid-lowering medications, particularly statins, improve perfusion defects on stress MPI by their beneficial effects on endothelial integrity and function, decreasing vascular inflammation, improving intrinsic coronary wall elasticity, improving coronary reserve and arterial wall responsiveness to vasodilators, and to a lesser extent by mild regression of fixed stenoses.147 Lipid-lowering medications have been shown to improve the perfusion abnormality of exercise and vasodilator SPECT or PET in several studies.156–163 The improvement occurred as early as 2 months in some studies and more definitely beyond 6 months of lipid-lowering therapy.147
Combined anti-ischemia medications, including calcium channel blockers, β-blockers, nitrates, and statins, decreased perfusion defect size and severity with dipyridamole and adenosine SPECT.148,164 In a study of stable patients after MI who underwent sequential adenosine SPECT studies, intensive medical therapy was comparable to coronary revascularization for suppressing ischemia, as demonstrated by before-and-after treatment.165
In summary, acute or chronic administration of nitrates has been shown to decrease the size and severity of exercise-induced myocardial perfusion defects.147 β-blockers ameliorate the perfusion pattern of exercise and dobutamine MPI, but the results are inconsistent with vasodilator MPI. The changes are likely due to alterations in MBF.147 Calcium channel blockers improve myocardial perfusion during exercise in patients with CAD.147 Their effects on vasodilator MPI have not been studied. In patients with known CAD who are undergoing exercise MPI and are on anti-anginal medications, it is important to continue these medications until the day of the exercise study to determine the protective effects of such medications. In patients with suspected but unproven CAD, it may be useful to discontinue such medications for 24 to 48 hours, especially if exercise perfusion imaging is used.5 We still believe that such medications have fewer effects on the results of vasodilator imaging and may be continued until the day of the study. Thus vasodilator imaging is not the most appropriate stress modality to study drug effect, unless these medications are given in very high doses.5
The effects of caffeine on adenosine appear to be related to the dose of caffeine and could be overcome by a higher dose of adenosine. In a study of 10 patients with CAD, FFR was not significantly different before and after the infusion of 4 mg/kg intravenous caffeine (mean caffeine level of 3.7 ± 1.8 mg/L).166 In a study of 30 patients with ischemia on adenosine SPECT perfusion imaging done while off caffeine, the ingestion of an 8-oz cup of coffee 1 hour before adenosine SPECT did not affect the results.167 The respective total quantitative perfusion defect and the SDS were 12 ± 10% and 3.8 ± 1.9 before caffeine and 12 ± 10% (P = ns) and 3.9 ± 2.3 (P = ns) after caffeine (mean level of 3.1 ± 1.6 mg/L).167 In another study of 30 patients, 2 cups of coffee (200 mg of caffeine) attenuated the results of 140 μ/kg/min infusion of adenosine with exercise (SDS of 12.0 ± 4.4 at baseline versus SDS of 4.1 ± 2.1 after caffeine [mean level of 6.2 ± 2.6 mg/L], P < 0.001) but did not have any effect on the results of a 210 μ/kg/min infusion of adenosine with exercise (SDS of 7.7 ± 4.0 at baseline versus 7.8 ± 4.2 after caffeine [mean level of 5.7 ± 2.0], P = 0.7)168 (Fig. 15-9).
NEW AGENT DEVELOPMENT
Compared to adenosine, the selective adenosine A2A receptor agonists can be administered as a single bolus and are more specific for the A2A receptor, causing selective coronary vasodilatation with lesser side effects.169 Apadenoson (BMS068645 or ATL146e), regadenoson (CVT-Lexiscan), and binodenoson (MRE-0470 and WRC-0470) are selective A2A agonists that have been clinically tested.169,170 Lexiscan was approved by the U.S. Food and Drug Administration in April 2008. The three selective agonists differ in their affinity to the A2A receptor, onset and duration of hyperemia, and mode of administration as fixed-dose or weight-based boluses.170 Regadenoson is administered as a fixed bolus, whereas the other two agonists are weight-based.170 Binodenoson has higher receptor affinity and thus has longer duration of hyperemia than regadenoson and adenosine.170 The clinical results of apadenoson have not yet been published.
Binodenoson
In a study that used a Doppler flow wire to study the coronary hemodynamic response to five different binodenoson doses, the 1.5 μg/kg intravenous bolus produced coronary hyperemia similar to intravenous adenosine, with lower side effects than the 3.0 μg/kg bolus dose.171 The 1.5 μg/kg dose produced coronary hyperemia within seconds that reached its maximal effect by 4.5 ± 3.7 minutes and was sustained for 7.4 ± 6.9 minutes. This was accompanied by modest changes in BP, HR, and double-product and produced no adverse ECG changes.171 In the phase II clinical trial, binodenoson had dose-dependent side effects (Table 15-9) and comparable ischemia detection to adenosine.172 There were no second- or third-degree AV blocks, bronchospasm, or hypotension. Chest pain, flushing, and dyspnea occurred less commonly with binodenoson compared to adenosine.172 There was very good to excellent agreement between binodenoson and adenosine with respect to the extent and severity of reversible perfusion defects.172 The results of the phase III study, a double-blind, double-dummy MPI of 1.5 μg/kg binodenoson bolus along with a 6-minute placebo infusion compared with placebo bolus along with a 6-minute 140 μg/kg/min adenosine infusion, were recently presented during the 2008 American College of Cardiology late-breaking clinical trials. The mean paired summed difference score difference of binodenoson versus adenosine images was −0.09 with 61% concordance between the two agents, and 3% complete discordance. The incidence of second- or third-degree AV blocks was 0% with binodenoson and 3% with adenosine. Compared to adenosine, binodenoson caused less flushing (50% versus 32%; P < 0.05), chest pain (61% versus 38%; P < 0.05), and dyspnea (51% versus 42%; P < 0.05) with lower patient-rated intensities. Binodenoson caused higher maximal changes in HR (25.3 versus 22.5 beats/min, P < 0.001) and similar changes in BP compared to adenosine. However, the Cardiovascular and Renal Drugs Advisory Committee that met in July 2009 did not recommend binodenoson for approval by the FDA due to design, endpoint and analysis related issues in the phase III trial.
Regadenoson
In a study that used a Doppler flow wire to study the coronary hemodynamic response, regadenoson increased peak blood flow velocity by up to 3.4-fold in a dose-dependent manner.173 Regadenoson (400 to 500 μg) caused a mean duration of CFR ≥ 2.5 for 2.3 to 2.4 minutes, increased HR by up to 21 ± 6 beats/min, decreased systolic BP by up to −24 ± 16 mm Hg, and decreased diastolic BP by up to 15 ± 14 mm Hg.173 Aminophylline at 100 mg attenuated the increase in peak blood flow velocity but not the tachycardia caused by 400 μg of regadenoson.173 The hemodynamic and side effects of regadenoson from the phase III trial are listed in Table 15-9.174 Regadenoson was better tolerated and preferred over adenosine and caused a greater and faster increase in HR, a similar drop in diastolic and systolic BP, and a slower recovery of BP and HR to baseline.174 There was good concordance for ischemia detection extent and severity between adenosine and regadenoson.174 Regadenoson was efficacious in detecting ischemia regardless of age, gender, body mass index, and presence of diabetes mellitus.175
In summary, despite the selectivity of the newer agents to the A2A receptor, subjective side effects such as flushing, dyspnea, and chest pain still occur with variable frequencies though are more transient and less severe.3,174 This might be due to the dose-dependent effects used in these clinical trials that might have rendered them less selective for the A2A receptor.3,174 Moreover, chest pain and dyspnea might be induced by A2A sympathetic receptor stimulation, as evidenced by rapid and sustained increase in HR in the face of slight drop in BP.3,174 However, the more serious side effects such as hypotension, AV blocks, and bronchospasm have not been observed so far, leading to optimism about the future role of these agents as stressors with ease of bolus administration and less serious adverse effects.3,174 In a pilot safety study of 48 patients with mild or moderate asthma who had bronchial reactivity to adenosine monophosphate, regadenoson was safe and well tolerated.176 In another pilot study of 49 outpatients with moderate or severe chronic obstructive pulmonary disease, regadenoson was overall safe when compared to placebo.177 New-onset wheezing was observed in 6% and 12% after regadenoson and placebo, respectively (P = 0.33).177 Mean maximum decline in FEV1 was similar between the two groups. No patient required acute treatment with bronchodilators or oxygen.177
Regadenoson is marketed as Lexiscan and is administered as a single 0.4-mg peripheral intravenous bolus (<10 seconds) followed immediately by a 5-mL saline solution flush. The radiotracer is injected 10 to 20 seconds after the saline flush and may be injected directly into the same catheter as Lexiscan (see Fig. 15-3).174
1. Hendel R.C., Jamil T., Glover D.K. Pharmacologic stress testing: New methods and new agents. J Nucl Cardiol. 2003;10:197-204.
2. Orlandi C. Pharmacology of coronary vasodilation: a brief review. J Nuc Cardiol. 1996;3:S27-S30.
3. Zoghbi G., Iskandrian A.E. Chapter 16: Pharmacologic Stress Testing. In: Garcia E.V., Iskandrian A.E., editors. Nuclear Cardiac Imaging: Principles and Applications. New York: Oxford University Press; 2008:293-315.
4. Henzlova M.J., Cerqueira M.D., Mahmarian J.J., Yao S.S. Stress protocols and tracers. J Nucl Cardiol. 2006;13:e80-e90.
5. Zoghbi G.J., Iskandrian A.E. Chapter 14: Coronary Artery Disease detection: Pharmacologic Stress. In: Zaret B.L., Beller G.A., editors. Clinical Nuclear Cardiology: State of the Art and Future Directions. Philadelphia: Mosby, Inc; 2005:233-253.
6. Abreu A., Mahmarian J.J., Nishimura S., Boyce T.M., Verani M.S. Tolerance and safety of pharmacologic coronary vasodilation with adenosine in association with thallium-201 scintigraphy in patients with suspected coronary artery disease. J Am Coll Cardiol. 1991;18:730-735.
7. Bertolet B.D., Belardinelli L., Franco E.A., Nichols W.W., Kerensky R.A., Hill J.A. Selective attenuation by N-0861 (N6-endonorboran-2-yl-9-methyladenine) of cardiac A1 adenosine receptor-mediated effects in humans. Circulation. 1996;93:1871-1876.
8. Sato A., Terata K., Miura H., Toyama K., Loberiza F.R.Jr, Hatoum O.A., Saito T., Sakuma I., Gutterman D.D. Mechanism of vasodilation to adenosine in coronary arterioles from patients with heart disease. Am J Physiol Heart Circ Physiol. 2005;288:H1633-H1640.
9. Hendel R.C., Wackers F.J., Berman D.S., Ficaro E., Depuey E.G., Klein L., Cerqueira M. American society of nuclear cardiology consensus statement: reporting of radionuclide myocardial perfusion imaging studies. J Nucl Cardiol. 2003;10:705-708.
10. Elhendy A., Bax J.J., Poldermans D. Dobutamine stress myocardial perfusion imaging in coronary artery disease. J Nucl Med. 2002;43:1634-1646.
11. Geleijnse M.L., Elhendy A., Fioretti P.M., Roelandt J.R. Dobutamine stress myocardial perfusion imaging. J Am Coll Cardiol. 2000;36:2017-2027.
12. Meuwissen M., Siebes M., Chamuleau S.A., van Eck-Smit B.L., Koch K.T., de Winter R.J., Tijssen J.G., Spaan J.A., Piek J.J. Hyperemic stenosis resistance index for evaluation of functional coronary lesion severity. Circulation. 2002;106:441-446.
13. Ogilby J.D. Role of adenosine in the cardiac catheterization laboratory. Am J Cardiol. 1997;79:15-19.
14. Chareonthaitawee P., Kaufmann P.A., Rimoldi O., Camici P.G. Heterogeneity of resting and hyperemic myocardial blood flow in healthy humans. Cardiovasc Res. 2001;50:151-161.
15. Czernin J., Muller P., Chan S., Brunken R.C., Porenta G., Krivokapich J., Chen K., Chan A., Phelps M.E., Schelbert H.R. Influence of age and hemodynamics on myocardial blood flow and flow reserve. Circulation. 1993;88:62-69.
16. Kaufmann P.A., Gnecchi-Ruscone T., Schafers K.P., Luscher T.F., Camici P.G. Low density lipoprotein cholesterol and coronary microvascular dysfunction in hypercholesterolemia. J Am Coll Cardiol. 2000;36:103-109.
17. Yokoyama I., Momomura S., Ohtake T., Yonekura K., Nishikawa J., Sasaki Y., Omata M. Reduced myocardial flow reserve in non-insulin-dependent diabetes mellitus. J Am Coll Cardiol. 1997;30:1472-1477.
18. Wilson R.F., Wyche K., Christensen B.V., Zimmer S., Laxson D.D. Effects of adenosine on human coronary arterial circulation. Circulation. 1990;82:1595-1606.
19. Rossen J.D., Quillen J.E., Lopez A.G., Stenberg R.G., Talman C.L., Winniford M.D. Comparison of coronary vasodilation with intravenous dipyridamole and adenosine. J Am Coll Cardiol. 1991;18:485-491.
20. Chan S.Y., Brunken R.C., Czernin J., Porenta G., Kuhle W., Krivokapich J., Phelps M.E., Schelbert H.R. Comparison of maximal myocardial blood flow during adenosine infusion with that of intravenous dipyridamole in normal men. J Am Coll Cardiol. 1992;20:979-985.
21. Czernin J., Auerbach M., Sun K.T., Phelps M., Schelbert H.R. Effects of modified pharmacologic stress approaches on hyperemic myocardial blood flow. J Nucl Med. 1995;36:575-580.
22. Krivokapich J., Huang S.C., Schelbert H.R. Assessment of the effects of dobutamine on myocardial blood flow and oxidative metabolism in normal human subjects using nitrogen-13 ammonia and carbon-11 acetate. Am J Cardiol. 1993;71:1351-1356.
23. Skopicki H.A., Abraham S.A., Picard M.H., Alpert N.M., Fischman A.J., Gewirtz H. Effects of dobutamine at maximally tolerated dose on myocardial blood flow in humans with ischemic heart disease. Circulation. 1997;96:3346-3352.
24. Tadamura E., Iida H., Matsumoto K., Mamede M., Kubo S., Toyoda H., Shiozaki T., Mukai T., Magata Y., Konishi J. Comparison of myocardial blood flow during dobutamine-atropine infusion with that after dipyridamole administration in normal men. J Am Coll Cardiol. 2001;37:130-136.
25. Muller P., Czernin J., Choi Y., Aguilar F., Nitzsche E.U., Buxton D.B., Sun K., Phelps M.E., Huang S.C., Schelbert H.R. Effect of exercise supplementation during adenosine infusion on hyperemic blood flow and flow reserve. Am Heart J. 1994;128:52-60.
26. Beller G.A., Zaret B.L. Contributions of nuclear cardiology to diagnosis and prognosis of patients with coronary artery disease. Circulation. 2000;101:1465-1478.
27. Gould K.L. Noninvasive assessment of coronary stenoses by myocardial perfusion imaging during pharmacologic coronary vasodilatation. I. Physiologic basis and experimental validation. Am J Cardiol. 1978;41:267-278.
28. Uren N.G., Melin J.A., De Bruyne B., Wijns W., Baudhuin T., Camici P.G. Relation between myocardial blood flow and the severity of coronary-artery stenosis. N Engl J Med. 1994;330:1782-1788.
29. Wilson R.F., Marcus M.L., White C.W. Prediction of the physiologic significance of coronary arterial lesions by quantitative lesion geometry in patients with limited coronary artery disease. Circulation. 1987;75:723-732.
30. Krivokapich J., Czernin J., Schelbert H.R. Dobutamine positron emission tomography: absolute quantitation of rest and dobutamine myocardial blood flow and correlation with cardiac work and percent diameter stenosis in patients with and without coronary artery disease. J Am Coll Cardiol. 1996;28:565-572.
31. Jagathesan R., Barnes E., Rosen S.D., Foale R.A., Camici P.G. Comparison of myocardial blood flow and coronary flow reserve during dobutamine and adenosine stress: Implications for pharmacologic stress testing in coronary artery disease. J Nucl Cardiol. 2006;13:324-332.
32. Kaul S. The role of capillaries in determining coronary blood flow reserve: Implications for stress-induced reversible perfusion defects. J Nucl Cardiol. 2001;8:694-700.
33. Okada R.D., Glover D.K., Nguyen K.N., Johnson G.3rd. Technetium-99m sestamibi kinetics in reperfused canine myocardium. Eur J Nucl Med. 1995;22:600-607.
34. Voudris V., Manginas A., Vassilikos V., Koutelou M., Kantzis J., Cokkinos D.V. Coronary flow velocity changes after intravenous dipyridamole infusion: measurements using intravascular Doppler guide wire. A documentation of flow inhomogeneity. J Am Coll Cardiol. 1996;27:1148-1155.
35. Deychak Y.A., Segal J., Reiner J.S., Rohrbeck S.C., Thompson M.A., Lundergan C.F., Ross A.M., Wasserman A.G. Doppler guide wire flow-velocity indexes measured distal to coronary stenoses associated with reversible thallium perfusion defects. Am Heart J. 1995;129:219-227.
36. Ogilby J.D., Iskandrian A.S., Untereker W.J., Heo J., Nguyen T.N., Mercuro J. Effect of intravenous adenosine infusion on myocardial perfusion and function, Hemodynamic/angiographic and scintigraphic study. Circulation. 1992;86:887-895.
37. Boger L.A., Volker L.L., Hertenstein G.K., Bateman T.M. Best patient preparation before and during radionuclide myocardial perfusion imaging studies. J Nucl Cardiol. 2006;13:98-110.
38. Hansen C.L., Goldstein R.A., Berman D.S., Churchwell K.B., Cooke C.D., Corbett J.R., Cullom S.J., Dahlberg S.T., Galt J.R., Garg R.K., Heller G.V., Hyun M.C., Johnson L.L., Mann A., McCallister B.D., Taillefer R., Ward R.P. Myocardial perfusion and function SPECT. J Nucl Cardiol. 2006;13:e89-114.
39. Tadehara F., Yamamoto H., Tsujiyama S., Hinoi T., Matsuo S., Matsumoto N., Sato Y., Kohno N. Feasibility of a rapid protocol of 1-day single-isotope rest/adenosine stress Tc-99m sestamibi ECG-gated myocardial perfusion imaging. J Nucl Cardiol. 2008;15:35-41.
40. Elhendy A., van Domburg R.T., Bax J.J., Nierop P.R., Valkema R., Geleijnse M.L., Kasprzak J.D., Liqui-Lung A.F., Cornel J.H., Roelandt J.R. Dobutamine-atropine stress myocardial perfusion SPECT imaging in the diagnosis of graft stenosis after coronary artery bypass grafting. J Nucl Cardiol. 1998;5:491-497.
41. Cerqueira M.D., Verani M.S., Schwaiger M., Heo J., Iskandrian A.S. Safety profile of adenosine stress perfusion imaging: results from the Adenoscan Multicenter Trial Registry. J Am Coll Cardiol. 1994;23:384-389.
42. Ranhosky A., Kempthorne-Rawson J. The safety of intravenous dipyridamole thallium myocardial perfusion imaging. Intravenous Dipyridamole Thallium Imaging Study Group. Circulation. 1990;81:1205-1209.
43. Iskandrian A.S. Adenosine myocardial perfusion imaging. J Nucl Med. 1994;35:734-736.
44. Taillefer R., Amyot R., Turpin S., Lambert R., Pilon C., Jarry M. Comparison between dipyridamole and adenosine as pharmacologic coronary vasodilators in detection of coronary artery disease with thallium 201 imaging. J Nucl Cardiol. 1996;3:204-211.
45. Crea F., Pupita G., Galassi A.R., el-Tamimi H., Kaski J.C., Davies G., Maseri A. Role of adenosine in pathogenesis of anginal pain. Circulation. 1990;81:164-172.
46. Balan K.K., Critchley M. Is the dyspnea during adenosine cardiac stress test caused by bronchospasm? Am Heart J. 2001;142:142-145.
47. Johnston D.L., Scanlon P.D., Hodge D.O., Glynn R.B., Hung J.C., Gibbons R.J. Pulmonary function monitoring during adenosine myocardial perfusion scintigraphy in patients with chronic obstructive pulmonary disease. Mayo Clin Proc. 1999;74:339-346.
48. Alkoutami G.S., Reeves W.C., Movahed A. The safety of adenosine pharmacologic stress testing in patients with first-degree atrioventricular block in the presence and absence of atrioventricular blocking medications. J Nucl Cardiol. 1999;6:495-497.
49. Alkoutami G.S., Reeves W.C., Movahed A. The frequency of atrioventricular block during adenosine stress testing in young, middle-aged, young-old, and old-old adults. Am J Geriatr Cardiol. 2001;10:159-161.
50. Samuels B., Kiat H., Friedman J.D., Berman D.S. Adenosine pharmacologic stress myocardial perfusion tomographic imaging in patients with significant aortic stenosis. Diagnostic efficacy and comparison of clinical, hemodynamic and electrocardiographic variables with 100 age-matched control subjects. J Am Coll Cardiol. 1995;25:99-106.
51. Lette J., Tatum J.L., Fraser S., Miller D.D., Waters D.D., Heller G., Stanton E.B., Bom H.S., Leppo J., Nattel S. Safety of dipyridamole testing in 73,806 patients: the Multicenter Dipyridamole Safety Study. J Nucl Cardiol. 1995;2:3-17.
52. Cerqueira M.D., Lawrence A. Nuclear cardiology update. Radiol Clin North Am. 2001;39:931-946. vii–viii
53. Dakik H.A., Vempathy H., Verani M.S. Tolerance, hemodynamic changes, and safety of dobutamine stress perfusion imaging. J Nucl Cardiol. 1996;3:410-414.
54. Elhendy A., Valkema R., van Domburg R.T., Bax J.J., Nierop P.R., Cornel J.H., Geleijnse M.L., Reijs A.E., Krenning E.P., Roelandt J.R. Safety of dobutamine-atropine stress myocardial perfusion scintigraphy. J Nucl Med. 1998;39:1662-1666.
55. Lee J.H., Abuannadi M., Jones P.G., Bateman T., Thompson R., O’Keefe J.H. Dobutamine-induced chest pain does not predict ischemic findings on myocardial perfusion imaging. J Nucl Cardiol. 2008;15:526-529.
56. Mahmarian J.J., Verani M.S. Myocardial perfusion imaging during pharmacologic stress testing. Cardiol Clin. 1994;12:223-245.
57. Bravo P.E., Hage F.G., Woodham R.M., Heo J., Iskandrian A.E. Heart rate response to adenosine in patients with diabetes mellitus and normal myocardial perfusion imaging. Am J Cardiol. 2008;102:1103-1106.
58. Aksut S.V., Pancholy S., Cassel D., Cave V., Heo J., Iskandrian A.S. Results of adenosine single photon emission computed tomography thallium-201 imaging in hemodynamic nonresponders. Am Heart J. 1995;130:67-70.
59. Amanullah A.M., Berman D.S., Kiat H., Friedman J.D. Usefulness of hemodynamic changes during adenosine infusion in predicting the diagnostic accuracy of adenosine technetium-99m sestamibi single-photon emission computed tomography (SPECT). Am J Cardiol. 1997;79:1319-1322.
60. Hays J.T., Mahmarian J.J., Cochran A.J., Verani M.S. Dobutamine thallium-201 tomography for evaluating patients with suspected coronary artery disease unable to undergo exercise or vasodilator pharmacologic stress testing. J Am Coll Cardiol. 1993;21:1583-1590.
61. Iskandrian A.S. Myocardial ischemia during pharmacological stress testing. Circulation. 1993;87:1415-1417.
62. Nishimura S., Kimball K.T., Mahmarian J.J., Verani M.S. Angiographic and hemodynamic determinants of myocardial ischemia during adenosine thallium-201 scintigraphy in coronary artery disease. Circulation. 1993;87:1211-1219.
63. Werner G.S., Fritzenwanger M., Prochnau D., Schwarz G., Ferrari M., Aarnoudse W., Pijls N.H., Figulla H.R. Determinants of coronary steal in chronic total coronary occlusions donor artery, collateral, and microvascular resistance. J Am Coll Cardiol. 2006;48:51-58.
64. Chambers C.E., Brown K.A. Dipyridamole-induced ST segment depression during thallium-201 imaging in patients with coronary artery disease: angiographic and hemodynamic determinants. J Am Coll Cardiol. 1988;12:37-41.
65. Iskandrian A.E., Heo J. Myocardial perfusion imaging during adenosine-induced coronary hyperemia. Am J Cardiol. 1997;79:20-24.
66. Gulati M., Pratap P., Kansal P., Calvin J.E.Jr, Hendel R.C. Gender differences in the value of ST-segment depression during adenosine stress testing. Am J Cardiol. 2004;94:997-1002.
67. Amanullah A.M., Aasa M. Significance of ST segment depression during adenosine-induced coronary hyperemia in angina pectoris and correlation with angiographic, scintigraphic, hemodynamic, and echocardiographic variables. Int J Cardiol. 1995;48:167-176.
68. Marshall E.S., Raichlen J.S., Kim S.M., Intenzo C.M., Sawyer D.T., Brody E.A., Tighe D.A., Park C.H. Prognostic significance of ST-segment depression during adenosine perfusion imaging. Am Heart J. 1995;130:58-66.
69. Abbott B.G., Afshar M., Berger A.K., Wackers F.J. Prognostic significance of ischemic electrocardiographic changes during adenosine infusion in patients with normal myocardial perfusion imaging. J Nucl Cardiol. 2003;10:9-16.
70. Hage F.G., Dubovsky E.V., Heo J., Iskandrian A.E. Outcome of patients with adenosine-induced ST-segment depression but with normal perfusion on tomographic imaging. Am J Cardiol. 2006;98:1009-1011.
71. Klodas E., Miller T.D., Christian T.F., Hodge D.O., Gibbons R.J. Prognostic significance of ischemic electrocardiographic changes during vasodilator stress testing in patients with normal SPECT images. J Nucl Cardiol. 2003;10:4-8.
72. Verani M.S. Pharmacologic stress myocardial perfusion imaging. Curr Probl Cardiol. 1993;18:481-525.
73. Kumar S.P., Brewington S.D., O’Brien K.F., Movahed A. Clinical correlation between increased lung to heart ratio of tecnetium-99m sestamibi and multivessel coronary artery disease. Int J Cardiol. 2005;101:219-222.
74. Hansen C.L., Cen P., Sanchez B., Robinson R. Comparison of pulmonary uptake with transient cavity dilation after dipyridamole Tl-201 perfusion imaging. J Nucl Cardiol. 2002;9:47-51.
75. McLaughlin M.G., Danias P.G. Transient ischemic dilation: a powerful diagnostic and prognostic finding of stress myocardial perfusion imaging. J Nucl Cardiol. 2002;9:663-667.
76. Mazzanti M., Germano G., Kiat H., Kavanagh P.B., Alexanderson E., Friedman J.D., Hachamovitch R., Van Train K.F., Berman D.S. Identification of severe and extensive coronary artery disease by automatic measurement of transient ischemic dilation of the left ventricle in dual-isotope myocardial perfusion SPECT. J Am Coll Cardiol. 1996;27:1612-1620.
77. Abidov A., Bax J.J., Hayes S.W., Hachamovitch R., Cohen I., Gerlach J., Kang X., Friedman J.D., Germano G., Berman D.S. Transient ischemic dilation ratio of the left ventricle is a significant predictor of future cardiac events in patients with otherwise normal myocardial perfusion SPECT. J Am Coll Cardiol. 2003;42:1818-1825.
78. Abidov A., Berman D.S. Transient ischemic dilation associated with poststress myocardial stunning of the left ventricle in vasodilator stress myocardial perfusion SPECT: true marker of severe ischemia? J Nucl Cardiol. 2005;12:258-260.
79. Emmett L., Magee M., Freedman S.B., Van der Wall H., Bush V., Trieu J., Van Gaal W., Allman K.C., Kritharides L. The role of left ventricular hypertrophy and diabetes in the presence of transient ischemic dilation of the left ventricle on myocardial perfusion SPECT images. J Nucl Med. 2005;46:1596-1601.
80. Hung G.U., Lee K.W., Chen C.P., Lin W.Y., Yang K.T. Relationship of transient ischemic dilation in dipyridamole myocardial perfusion imaging and stress-induced changes of functional parameters evaluated by Tl-201 gated SPECT. J Nucl Cardiol. 2005;12:268-275.
81. Chouraqui P., Rodrigues E.A., Berman D.S., Maddahi J. Significance of dipyridamole-induced transient dilation of the left ventricle during thallium-201 scintigraphy in suspected coronary artery disease. Am J Cardiol. 1990;66:689-694.
82. Takeishi Y., Tono-oka I., Ikeda K., Komatani A., Tsuiki K., Yasui S. Dilatation of the left ventricular cavity on dipyridamole thallium-201 imaging: a new marker of triple-vessel disease. Am Heart J. 1991;121:466-475.
83. Tanaka H., Chikamori T., Hida S., Usui Y., Harafuji K., Igarashi Y., Yamashina A. Comparison of post-exercise and post-vasodilator stress myocardial stunning as assessed by electrocardiogram-gated single-photon emission computed tomography. Circ J. 2005;69:1338-1345.
84. Ward R.P., Gundeck E.L., Lang R.M., Spencer K.T., Williams K.A. Overestimation of postischemic myocardial stunning on gated SPECT imaging: correlation with echocardiography. J Nucl Cardiol. 2006;13:514-520.
85. D’Antono B., Dupuis G., Fortin C., Arsenault A., Burelle D. Detection of exercise-induced myocardial ischemia from symptomatology experienced during testing in men and women. Can J Cardiol. 2006;22:411-417.
86. Hung G.U., Lee K.W., Chen C.P., Yang K.T., Lin W.Y. Worsening of left ventricular ejection fraction induced by dipyridamole on Tl-201 gated myocardial perfusion imaging predicts significant coronary artery disease. J Nucl Cardiol. 2006;13:225-232.
87. Druz R.S., Akinboboye O.A., Grimson R., Nichols K.J., Reichek N. Postischemic stunning after adenosine vasodilator stress. J Nucl Cardiol. 2004;11:534-541.
88. Ohtaki Y., Chikamori T., Igarashi Y., Hida S., Tanaka H., Hatano T., Usui Y., Miyagi M., Yamashina A. Differential effects comparing exercise and pharmacologic stress on left ventricular function using gated Tc-99m sestamibi SPECT. Ann Nucl Med. 2008;22:185-190.
89. Brinkman N., Dibbets-Schneider P., Scholte A.J., Stokkel M.P. Myocardial perfusion scintigraphy with adenosine: does it impair the left ventricular ejection fraction obtained with gated SPECT? Clin Nucl Med. 2008;33:89-93.
90. Pijls N.H., De Bruyne B., Peels K., Van Der Voort P.H., Bonnier H.J., Bartunek J.K.J.J., Koolen J.J. Measurement of fractional flow reserve to assess the functional severity of coronary-artery stenoses. N Engl J Med. 1996;334:1703-1708.
91. Rodes-Cabau J., Candell-Riera J., Angel J., de Leon G., Pereztol O., Castell-Conesa J., Soto A., Anivarro I., Aguade S., Vazquez M., Domingo E., Tardif J.C., Soler-Soler J. Relation of myocardial perfusion defects and nonsignificant coronary lesions by angiography with insights from intravascular ultrasound and coronary pressure measurements. Am J Cardiol. 2005;96:1621-1626.
92. Klocke F.J., Baird M.G., Lorell B.H., Bateman T.M., Messer J.V., Berman D.S., O’Gara P.T., Carabello B.A., Russell R.O.Jr, Cerqueira M.D., St John Sutton M.G., DeMaria A.N., Udelson J.E., Kennedy J.W., Verani M.S., Williams K.A., Antman E.M., Smith S.C.Jr, Alpert J.S., Gregoratos G., Anderson J.L., Hiratzka L.F., Faxon D.P., Hunt S.A., Fuster V., Jacobs A.K., Gibbons R.J., Russell R.O. ACC/AHA/ASNC guidelines for the clinical use of cardiac radionuclide imaging—executive summary: a report of the American College of Cardiology/American Heart Association Task Force on Practice Guidelines (ACC/AHA/ASNC Committee to Revise the 1995 Guidelines for the Clinical Use of Cardiac Radionuclide Imaging). J Am Coll Cardiol. 2003;42:1318-1333.
93. Johansen A., Hoilund-Carlsen P.F., Christensen H.W., Vach W., Jorgensen H.B., Veje A., Haghfelt T. Diagnostic accuracy of myocardial perfusion imaging in a study population without post-test referral bias. J Nucl Cardiol. 2005;12:530-537.
94. Kim C., Kwok Y.S., Heagerty P., Redberg R. Pharmacologic stress testing for coronary disease diagnosis: A meta-analysis. Am Heart J. 2001;142:934-944.
95. Tartagni F., Dondi M., Limonetti P., Franchi R., Maiello L., Monetti N., Magnani B. Dipyridamole technetium-99m-2-methoxy isobutyl isonitrile tomoscintigraphic imaging for identifying diseased coronary vessels: comparison with thallium-201 stress-rest study. J Nucl Med. 1991;32:369-376.
96. Soman P., Taillefer R., DePuey E.G., Udelson J.E., Lahiri A. Enhanced detection of reversible perfusion defects by Tc-99m sestamibi compared to Tc-99m tetrofosmin during vasodilator stress SPECT imaging in mild-to-moderate coronary artery disease. J Am Coll Cardiol. 2001;37:458-462.
97. Berman D.S., Kang X., Hayes S.W., Friedman J.D., Cohen I., Abidov A., Shaw L.J., Amanullah A.M., Germano G., Hachamovitch R. Adenosine myocardial perfusion single-photon emission computed tomography in women compared with men. Impact of diabetes mellitus on incremental prognostic value and effect on patient management. J Am Coll Cardiol. 2003;41:1125-1133.
98. Nishina H., Slomka P.J., Abidov A., Yoda S., Akincioglu C., Kang X., Cohen I., Hayes S.W., Friedman J.D., Germano G., Berman D.S. Combined Supine and Prone Quantitative Myocardial Perfusion SPECT: Method Development and Clinical Validation in Patients with No Known Coronary Artery Disease. J Nucl Med. 2006;47:51-58.
99. Wright D.J., Williams S.G., Lindsay H.S., Sheard K.L., Thorley P.J., Sivananthan U.M. Assessment of adenosine, arbutamine and dobutamine as pharmacological stress agents during (99m)Tc-tetrofosmin SPECT imaging: a randomized study. Nucl Med Commun. 2001;22:1305-1311.
100. Coyne E.P., Belvedere D.A., Vande Streek P.R., Weiland F.L., Evans R.B., Spaccavento L.J. Thallium-201 scintigraphy after intravenous infusion of adenosine compared with exercise thallium testing in the diagnosis of coronary artery disease. J Am Coll Cardiol. 1991;17:1289-1294.
101. Gupta N.C., Esterbrooks D.J., Hilleman D.E., Mohiuddin S.M. Comparison of adenosine and exercise thallium-201 single-photon emission computed tomography (SPECT) myocardial perfusion imaging. The GE SPECT Multicenter Adenosine Study Group. J Am Coll Cardiol. 1992;19:248-257.
102. Nishimura S., Mahmarian J.J., Boyce T.M., Verani M.S. Equivalence between adenosine and exercise thallium-201 myocardial tomography: a multicenter, prospective, crossover trial. J Am Coll Cardiol. 1992;20:265-275.
103. Cuocolo A., Soricelli A., Pace L., Nicolai E., Castelli L., Nappi A., Imbriaco M., Morisco C., Ell P.J., Salvatore M. Adenosine technetium-99m-methoxy isobutyl isonitrile myocardial tomography in patients with coronary artery disease: comparison with exercise. J Nucl Med. 1994;35:1110-1115.
104. Vallejo E., Morales M., Sanchez I., Sanchez G., Alburez J.C., Bialostozky D. Myocardial perfusion SPECT imaging in patients with myocardial bridging. J Nucl Cardiol. 2005;12:318-323.
105. Chow B.J., Beanlands R.S., Lee A., DaSilva J.N., deKemp R.A., Alkahtani A., Ruddy T.D. Treadmill exercise produces larger perfusion defects than dipyridamole stress N-13 ammonia positron emission tomography. J Am Coll Cardiol. 2006;47:411-416.
106. Santos-Ocampo C.D., Herman S.D., Travin M.I., Garber C.E., Ahlberg A.W., Messinger D.E., Heller G.V. Comparison of exercise, dipyridamole, and adenosine by use of technetium 99m sestamibi tomographic imaging. J Nucl Cardiol. 1994;1:57-64.
107. Levine M.G., Ahlberg A.W., Mann A., White M.P., McGill C.C., Mendes de Leon C., Piriz J.M., Waters D., Heller G.V. Comparison of exercise, dipyridamole, adenosine, and dobutamine stress with the use of Tc-99m tetrofosmin tomographic imaging. J Nucl Cardiol. 1999;6:389-396.
108. Mieres J.H., Shaw L.J. Stress myocardial perfusion imaging in the diagnosis and prognosis of women with suspected coronary artery disease. Cardiol Rev. 2003;11:330-336.
109. Elhendy A., Schinkel A.F., Bax J.J., van Domburg R.T., Valkema R., Biagini E., Feringa H.H., Poldermans D. Accuracy of stress Tc-99m tetrofosmin myocardial perfusion tomography for the diagnosis and localization of coronary artery disease in women. J Nucl Cardiol. 2006;13:629-634.
110. Shaw L.J., Bairey Merz C.N., Pepine C.J., Reis S.E., Bittner V., Kelsey S.F., Olson M., Johnson B.D., Mankad S., Sharaf B.L., Rogers W.J., Wessel T.R., Arant C.B., Pohost G.M., Lerman A., Quyyumi A.A., Sopko G. Insights from the NHLBI-Sponsored Women’s Ischemia Syndrome Evaluation (WISE) Study: Part I: gender differences in traditional and novel risk factors, symptom evaluation, and gender-optimized diagnostic strategies. J Am Coll Cardiol. 2006;47:S4-S20.
111. Amanullah A.M., Kiat H., Friedman J.D., Berman D.S. Adenosine technetium-99m sestamibi myocardial perfusion SPECT in women: diagnostic efficacy in detection of coronary artery disease. J Am Coll Cardiol. 1996;27:803-809.
112. Iskandrian A.E., Heo J., Nallamothu N. Detection of coronary artery disease in women with use of stress single-photon emission computed tomography myocardial perfusion imaging. J Nucl Cardiol. 1997;4:329-335.
113. Taillefer R., DePuey E.G., Udelson J.E., Beller G.A., Latour Y., Reeves F. Comparative diagnostic accuracy of Tl-201 and Tc-99m sestamibi SPECT imaging (perfusion and ECG-gated SPECT) in detecting coronary artery disease in women. J Am Coll Cardiol. 1997;29:69-77.
114. Iskandrian A.S., Heo J., Lemlek J., Ogilby J.D., Untereker W.J., Iskandrian B., Cave V. Identification of high-risk patients with left main and three-vessel coronary artery disease by adenosine-single photon emission computed tomographic thallium imaging. Am Heart J. 1993;125:1130-1135.
115. Ho K.T., Miller T.D., Christian T.F., Hodge D.O., Gibbons R.J. Prediction of severe coronary artery disease and long-term outcome in patients undergoing vasodilator SPECT. J Nucl Cardiol. 2001;8:438-444.
116. Amanullah A.M., Berman D.S., Hachamovitch R., Kiat H., Kang X., Friedman J.D. Identification of severe or extensive coronary artery disease in women by adenosine technetium-99m sestamibi SPECT. Am J Cardiol. 1997;80:132-137.
117. Berman D.S., Kang X., Slomka P.J., Gerlach J., de Yang L., Hayes S.W., Friedman J.D., Thomson L.E., Germano G. Underestimation of extent of ischemia by gated SPECT myocardial perfusion imaging in patients with left main coronary artery disease. J Nucl Cardiol. 2007;14:521-528.
118. Villegas B.J., Hendel R.C., Dahlberg S.T., McSherry B.A., Leppo J.A. Comparison of 3- versus 6-minute infusions of adenosine in thallium-201 myocardial perfusion imaging. Am Heart J. 1993;126:103-107.
119. Treuth M.G., Reyes G.A., He Z.X., Cwajg E., Mahmarian J.J., Verani M.S. Tolerance and diagnostic accuracy of an abbreviated adenosine infusion for myocardial scintigraphy: a randomized, prospective study. J Nucl Cardiol. 2001;8:548-554.
120. O’Keefe J.H.Jr, Bateman T.M., Handlin L.R., Barnhart C.S. Four- versus 6-minute infusion protocol for adenosine thallium-201 single photon emission computed tomography imaging. Am Heart J. 1995;129:482-487.
121. Elliott M.D., Holly T.A., Leonard S.M., Hendel R.C. Impact of an abbreviated adenosine protocol incorporating adjunctive treadmill exercise on adverse effects and image quality in patients undergoing stress myocardial perfusion imaging. J Nucl Cardiol. 2000;7:584-589.
122. Pennell D.J., Mavrogeni S.I., Forbat S.M., Karwatowski S.P., Underwood S.R. Adenosine combined with dynamic exercise for myocardial perfusion imaging. J Am Coll Cardiol. 1995;25:1300-1309.
123. Samady H., Wackers F.J., Joska T.M., Zaret B.L., Jain D. Pharmacologic stress perfusion imaging with adenosine: role of simultaneous low-level treadmill exercise. J Nucl Cardiol. 2002;9:188-196.
124. Thomas G.S., Prill N.V., Majmundar H., Fabrizi R.R., Thomas J.J., Hayashida C., Kothapalli S., Payne J.L., Payne M.M., Miyamoto M.I. Treadmill exercise during adenosine infusion is safe, results in fewer adverse reactions, and improves myocardial perfusion image quality. J Nucl Cardiol. 2000;7:439-446.
125. Casale P.N., Guiney T.E., Strauss H.W., Boucher C.A. Simultaneous low level treadmill exercise and intravenous dipyridamole stress thallium imaging. Am J Cardiol. 1988;62:799-802.
126. Ignaszewski A.P., McCormick L.X., Heslip P.G., McEwan A.J., Humen D.P. Safety and clinical utility of combined intravenous dipyridamole/symptom-limited exercise stress test with thallium-201 imaging in patients with known or suspected coronary artery disease. J Nucl Med. 1993;34:2053-2061.
127. Holly T.A., Satran A., Bromet D.S., Mieres J.H., Frey M.J., Elliott M.D., Heller G.V., Hendel R.C. The impact of adjunctive adenosine infusion during exercise myocardial perfusion imaging: Results of the Both Exercise and Adenosine Stress Test (BEAST) trial. J Nucl Cardiol. 2003;10:291-296.
128. Thomas G.S., Miyamoto M.I. Should simultaneous exercise become the standard for adenosine myocardial perfusion imaging? Am J Cardiol. 2004;94:3D-10D. discussion 10D-11D
129. Shehata A.R., Ahlberg A.W., White M.P., Mann A., Fleming I.A., Levine M.G., Mather J.F., Waters D., Heller G.V. Dipyridamole-dobutamine stress with Tc-99m sestamibi tomographic myocardial perfusion imaging. Am J Cardiol. 1998;82:520-523.
130. Aydin M., Caner B., Yildirir A., Sari O., Tokgozoglu L. Dobutamine combined with low-level exercise for myocardial perfusion scintigraphy. Nucl Med Commun. 2000;21:1015-1020.
131. Bokhari S., Pinsky D.J., Bergmann S.R. An improved dobutamine protocol for myocardial perfusion imaging. Am J Cardiol. 2001;88:1303-1305.
132. Nadig M.R., Patel C.D., Malhotra A. Comparison between dobutamine stress and combination of handgrip exercise with dobutamine stress in myocardial perfusion SPECT. Nucl Med Commun. 2007;28:301-304.
133. Leao Lima Rde S., De Lorenzo A., Issa A. Reduced adverse effects with an accelerated dobutamine stress protocol compared with the conventional protocol: a prospective, randomized myocardial perfusion scintigraphy study. Int J Cardiovasc Imaging. 2008;24:55-59.
134. Skalidis E.I., Kochiadakis G.E., Koukouraki S.I., Parthenakis F.I., Karkavitsas N.S., Vardas P.E. Phasic coronary flow pattern and flow reserve in patients with left bundle branch block and normal coronary arteries. J Am Coll Cardiol. 1999;33:1338-1346.
135. O’Keefe J.H.Jr, Bateman T.M., Barnhart C.S. Adenosine thallium-201 is superior to exercise thallium-201 for detecting coronary artery disease in patients with left bundle branch block. J Am Coll Cardiol. 1993;21:1332-1338.
136. Feola M., Biggi A., Vado A., Ribichini F., Ferrero V., Leonardi G., Uslenghi E. The usefulness of adenosine 99mTc tetrofosmin SPECT for the diagnosis of left anterior descending coronary artery disease in patients with chest pain and left bundle branch block. Nucl Med Commun. 2004;25:265-269.
137. Hoefflinghaus T., Husmann L., Valenta I., Moonen C., Gaemperli O., Schepis T., Namdar M., Koepfli P., Siegrist P.T., Kaufmann P.A. Role of attenuation correction to discriminate defects caused by left bundle branch block versus coronary stenosis in single photon emission computed tomography myocardial perfusion imaging. Clin Nucl Med. 2008;33:748-751.
138. Tse H.F., Lau C.P. Long-term effect of right ventricular pacing on myocardial perfusion and function. J Am Coll Cardiol. 1997;29:744-749.
139. Tse H.F., Yu C., Wong K.K., Tsang V., Leung Y.L., Ho W.Y., Lau C.P. Functional abnormalities in patients with permanent right ventricular pacing: the effect of sites of electrical stimulation. J Am Coll Cardiol. 2002;40:1451-1458.
140. Lakkis N.M., He Z.X., Verani M.S. Diagnosis of coronary artery disease by exercise thallium-201 tomography in patients with a right ventricular pacemaker. J Am Coll Cardiol. 1997;29:1221-1225.
141. DePuey E.G., Guertler-Krawczynska E., Perkins J.V., Robbins W.L., Whelchel J.D., Clements S.D. Alterations in myocardial thallium-201 distribution in patients with chronic systemic hypertension undergoing single-photon emission computed tomography. Am J Cardiol. 1988;62:234-238.
142. Houghton J.L., Prisant L.M., Carr A.A., Flowers N.C., Frank M.J. Racial differences in myocardial ischemia and coronary flow reserve in hypertension. J Am Coll Cardiol. 1994;23:1123-1129.
143. Vaduganathan P., He Z.X., Mahmarian J.J., Verani M.S. Diagnostic accuracy of stress thallium-201 tomography in patients with left ventricular hypertrophy. Am J Cardiol. 1998;81:1205-1207.
144. Elhendy A., van Domburg R.T., Sozzi F.B., Poldermans D., Bax J.J., Roelandt J.R. Impact of hypertension on the accuracy of exercise stress myocardial perfusion imaging for the diagnosis of coronary artery disease. Heart. 2001;85:655-661.
145. Yao S.S., Qureshi E., Nichols K., Diamond G.A., Depuey E.G., Rozanski A. Prospective validation of a quantitative method for differentiating ischemic versus nonischemic cardiomyopathy by technetium-99m sestamibi myocardial perfusion single-photon emission computed tomography. Clin Cardiol. 2004;27:615-620.
146. Her S.H., Yoon H.J., Lee J.M., Jin S.W., Youn H.J., Seung K.B., Kim J.H. Adenosine Tc-99m tetrofosmin SPECT in differentiation of ischemic from nonischemic cardiomyopathy in patients with LV systolic dysfunction. Clin Nucl Med. 2008;33:459-463.
147. Zoghbi G.J., Dorfman T.A., Iskandrian A.E. The effects of medications on myocardial perfusion. J Am Coll Cardiol. 2008;52:401-416.
148. Sharir T., Rabinowitz B., Livschitz S., Moalem I., Baron J., Kaplinsky E., Chouraqui P. Underestimation of extent and severity of coronary artery disease by dipyridamole stress thallium-201 single-photon emission computed tomographic myocardial perfusion imaging in patients taking antianginal drugs. J Am Coll Cardiol. 1998;31:1540-1546.
149. Koepfli P., Wyss C.A., Namdar M., Klainguti M., von Schulthess G.K., Luscher T.F., Kaufmann P.A. Beta-adrenergic blockade and myocardial perfusion in coronary artery disease: differential effects in stenotic versus remote myocardial segments. J Nucl Med. 2004;45:1626-1631.
150. Shehata A.R., Gillam L.D., Mascitelli V.A., Herman S.D., Ahlberg A.W., White M.P., Chen C., Waters D.D., Heller G.V. Impact of acute propranolol administration on dobutamine-induced myocardial ischemia as evaluated by myocardial perfusion imaging and echocardiography. Am J Cardiol. 1997;80:268-272.
151. Bridges A.B., Kennedy N., McNeill G.P., Cook B., Pringle T.H. The effect of atenolol on dipyridamole 201Tl myocardial perfusion tomography in patients with coronary artery disease. Nucl Med Commun. 1992;13:41-46.
152. Taillefer R., Ahlberg A.W., Masood Y., White C.M., Lamargese I., Mather J.F., McGill C.C., Heller G.V. Acute beta-blockade reduces the extent and severity of myocardial perfusion defects with dipyridamole Tc-99m sestamibi SPECT imaging. J Am Coll Cardiol. 2003;42:1475-1483.
153. Lakkireddy D., Aronow W.S., Bateman T., McGhee I., Nair C., Khan I.A. Does beta blocker therapy affect the diagnostic accuracy of adenosine single-photon-emission computed tomographic myocardial perfusion imaging? Am J Ther. 2008;15:19-23.
154. Bottcher M., Refsgaard J., Madsen M.M., Randsbaek F., Kaltoft A., Botker H.E., Nielsen T.T. Effect of antianginal medication on resting myocardial perfusion and pharmacologically induced hyperemia. J Nucl Cardiol. 2003;10:345-352.
155. Muller-Suur R., Eriksson S.V., Strandberg L.E., Mesko L. Comparison of adenosine and exercise stress test for quantitative perfusion imaging in patients on beta-blocker therapy. Cardiology. 2001;95:112-118.
156. Gould K.L., Martucci J.P., Goldberg D.I., Hess M.J., Edens R.P., Latifi R., Dudrick S.J. Short-term cholesterol lowering decreases size and severity of perfusion abnormalities by positron emission tomography after dipyridamole in patients with coronary artery disease. A potential noninvasive marker of healing coronary endothelium. Circulation. 1994;89:1530-1538.
157. Mostaza J.M., Gomez M.V., Gallardo F., Salazar M.L., Martin-Jadraque R., Plaza-Celemin L., Gonzalez-Maqueda I., Martin-Jadraque L. Cholesterol reduction improves myocardial perfusion abnormalities in patients with coronary artery disease and average cholesterol levels. J Am Coll Cardiol. 2000;35:76-82.
158. Treasure C.B., Klein J.L., Weintraub W.S., Talley J.D., Stillabower M.E., Kosinski A.S., Zhang J., Boccuzzi S.J., Cedarholm J.C., Alexander R.W. Beneficial effects of cholesterol-lowering therapy on the coronary endothelium in patients with coronary artery disease. N Engl J Med. 1995;332:481-487.
159. Schwartz R.G., Pearson T.A., Kalaria V.G., Mackin M.L., Williford D.J., Awasthi A., Shah A., Rains A., Guido J.J. Prospective serial evaluation of myocardial perfusion and lipids during the first six months of pravastatin therapy: coronary artery disease regression single photon emission computed tomography monitoring trial. J Am Coll Cardiol. 2003;42:600-610.
160. Guethlin M., Kasel A.M., Coppenrath K., Ziegler S., Delius W., Schwaiger M. Delayed response of myocardial flow reserve to lipid-lowering therapy with fluvastatin. Circulation. 1999;99:475-481.
161. Huggins G.S., Pasternak R.C., Alpert N.M., Fischman A.J., Gewirtz H. Effects of short-term treatment of hyperlipidemia on coronary vasodilator function and myocardial perfusion in regions having substantial impairment of baseline dilator reverse. Circulation. 1998;98:1291-1296.
162. Yokoyama I., Momomura S., Ohtake T., Yonekura K., Yang W., Kobayakawa N., Aoyagi T., Sugiura S., Yamada N., Ohtomo K., Sasaki Y., Omata M., Yazaki Y. Improvement of impaired myocardial vasodilatation due to diffuse coronary atherosclerosis in hypercholesterolemics after lipid-lowering therapy. Circulation. 1999;100:117-122.
163. Yokoyama I., Yonekura K., Inoue Y., Ohtomo K., Nagai R. Long-term effect of simvastatin on the improvement of impaired myocardial flow reserve in patients with familial hypercholesterolemia without gender variance. J Nucl Cardiol. 2001;8:445-451.
164. Dakik H.A., Kleiman N.S., Farmer J.A., He Z.X., Wendt J.A., Pratt C.M., Verani M.S., Mahmarian J.J. Intensive medical therapy versus coronary angioplasty for suppression of myocardial ischemia in survivors of acute myocardial infarction: a prospective, randomized pilot study. Circulation. 1998;98:2017-2023.
165. Mahmarian J.J., Dakik H.A., Filipchuk N.G., Shaw L.J., Iskander S.S., Ruddy T.D., Keng F., Henzlova M.J., Allam A., Moye L.A., Pratt C.M. An initial strategy of intensive medical therapy is comparable to that of coronary revascularization for suppression of scintigraphic ischemia in high-risk but stable survivors of acute myocardial infarction. J Am Coll Cardiol. 2006;48:2458-2467.
166. Aqel R.A., Zoghbi G.J., Trimm J.R., Baldwin S.A., Iskandrian A.E. Effect of caffeine administered intravenously on intracoronary-administered adenosine-induced coronary hemodynamics in patients with coronary artery disease. Am J Cardiol. 2004;93:343-346.
167. Zoghbi G.J., Htay T., Aqel R., Blackmon L., Heo J., Iskandrian A.E. Effect of caffeine on ischemia detection by adenosine single-photon emission computed tomography perfusion imaging. J Am Coll Cardiol. 2006;47:2296-2302.
168. Reyes E., Loong C.Y., Harbinson M., Donovan J., Anagnostopoulos C., Underwood S.R. High-dose adenosine overcomes the attenuation of myocardial perfusion reserve caused by caffeine. J Am Coll Cardiol. 2008;52:2008-2016.
169. Cerqueira M.D. Advances in pharmacologic agents in imaging: new A2A receptor agonists. Curr Cardiol Rep. 2006;8:119-122.
170. Iskandrian A.E. A new generation of coronary vasodilators in stress perfusion imaging. Am J Cardiol. 2007;99:1619-1620.
171. Hodgson J.M., Dib N., Kern M.J., Bach R.G., Barrett R.J. Coronary circulation responses to binodenoson, a selective adenosine A2A receptor agonist. Am J Cardiol. 2007;99:1507-1512.
172. Udelson J.E., Heller G.V., Wackers F.J., Chai A., Hinchman D., Coleman P.S., Dilsizian V., DiCarli M., Hachamovitch R., Johnson J.R., Barrett R.J., Gibbons R.J. Randomized, controlled dose-ranging study of the selective adenosine A2A receptor agonist binodenoson for pharmacological stress as an adjunct to myocardial perfusion imaging. Circulation. 2004;109:457-464.
173. Lieu H.D., Shryock J.C., von Mering G.O., Gordi T., Blackburn B., Olmsted A.W., Belardinelli L., Kerensky R.A. Regadenoson, a selective A2A adenosine receptor agonist, causes dose-dependent increases in coronary blood flow velocity in humans. J Nucl Cardiol. 2007;14:514-520.
174. Iskandrian A.E., Bateman T.M., Belardinelli L., Blackburn B., Cerqueira M.D., Hendel R.C., Lieu H., Mahmarian J.J., Olmsted A., Underwood S.R., Vitola J., Wang W. Adenosine versus regadenoson comparative evaluation in myocardial perfusion imaging: results of the ADVANCE phase 3 multicenter international trial. J Nucl Cardiol. 2007;14:645-658.
175. Cerqueira M.D., Nguyen P., Steahr P., Underwood R., Iskandrian A.E. on behalf of the ADVANCE-MPI trial investigators. Effects of age, gender, obesity, and diabetes on the efficacy and safety of the selective A2A agonist regadenoson versus adenosine in myocardial perfusion imaging: integrated ADVANCE-MPI trial results. JACC Cardiovasc Imaging. 2008;1:307-316.
176. Leaker B.R., O’Connor B., Hansel T.T., Barnes P.J., Meng L., Mathur V.S., Lieu H.D. Safety of regadenoson, an adenosine A2A receptor agonist for myocardial perfusion imaging, in mild asthma and moderate asthma patients: a randomized, double-blind, placebo-controlled trial. J Nucl Cardiol. 2008;15:329-336.
177. Thomas G.S., Tammelin B.R., Schiffman G.L., Marquez R., Rice D.L., Milikien D., Mathur V. Safety of regadenoson, a selective adenosine A2A agonist, in patients with chronic obstructive pulmonary disease: A randomized, double-blind, placebo-controlled trial (RegCOPD trial). J Nucl Cardiol. 2008;15:319-328.