Chapter 21 Coronary Artery Computed Tomography Angiography
COMPUTED TOMOGRAPHY CORONARY ARTERY ANGIOGRAPHY: TECHNOLOGIC CONSIDERATIONS
The main challenge in imaging the coronary arteries with CT is the complex and continuous motion of these small targets. Therefore, both high temporal and spatial resolution are of paramount importance to obtain diagnostic quality images. The field of noninvasive coronary angiography started with the introduction of electron beam CT (EBCT; Imatron, San Francisco, CA) that operated at a temporal resolution varying between 50 and 100 milliseconds (ms) per tomographic image. This was a fourth-generation CT scanner that utilized a beam of electrons directed toward a tungsten ring; the impact of the electrons against the tungsten created a fan of x-rays moved along an arc of 210 degrees around the gantry (Fig. 21-1). The issuance of the electron beam, followed by the generation of the x-ray fan, was timed at 60% to 80% of the R-R interval on the surface electrocardiogram (ECG), and the patient was moved in small increments of 3 mm through the stationary gantry at each heartbeat while holding his breath. This step-and-shoot prospective (i.e., ECG triggered) acquisition required a 20- to 30-second breath hold to cover the entire z-axis (cranial-caudal distance) of the heart. The benefits of the EBCT scanner were the very high temporal resolution and the relatively low radiation dose provided to the patient because of the intermittent (once per heartbeat) exposure to x-rays. However, there were also important drawbacks to the technology, such as the low spatial resolution, a significant partial volume effect due to the thick tomographic slices, and the fact that the axial acquisition of the slices with a small interslice gap often created a stair-step artifact in the reconstructed images. Finally, the prospective ECG-triggered acquisition forced patients with slow heart rates to maintain long breath holds, often inducing artifacts when the patients breathed in the middle of the test. In the early 2000s, the field witnessed an important evolution with the introduction of the first 4-slice mechanical CT. A dual-slice helical CT (Elscint, Haifa, Israel) had been in use for a few years1–3 but had not received much attention in cardiovascular circles. The development of multislice CT (also known as multidetector CT [MDCT]) was made possible by the introduction of the slip-ring technology that permitted the continuous revolution of the x-ray source-detector pair in the CT gantry, allowing a perpetual spiraling motion around the patient. With this new technology, an x-ray tube generates a beam of x-rays that is split, collimated, and detected by several rows of detectors positioned on the other side of the patient’s table (Figs. 21-2 and 21-3).
As can be deduced from the preceding discussion, the introduction of scanners with more than 4 rows (16 to 64), despite better spatial resolution, did not fully improve the temporal resolution limitation of MDCT. As a result, even when using most current MDCT technology, β-blockade is still necessary to limit motion artifacts if the heart rate is greater than 70 beats/min. Nonetheless, by increasing the distance covered in the z-axis during a single rotation of the gantry, they shortened the acquisition time. This weakness of MDCT was partially overcome with the introduction of dual-source 64-row MDCT scanners (Siemens, Forcheim, Germany).4–6 These scanners utilize two x-ray tubes and two sets of detector rows set at 90 degrees from each other that operate simultaneously, reducing the effective temporal resolution to about 83 ms per tomographic slice. Furthermore, the two tubes can issue x-rays of different energy spectra (dual-energy systems), with potential advantages for the study of tissues with different x-ray absorption characteristics, as discussed later in this chapter.
The temporal resolution of the dual-source MDCT is similar to that of EBCT, and no β-blockade appears to be necessary when imaging, reportedly even in the presence of atrial fibrillation.7,8
COMPARISON OF CORONARY COMPUTED TOMOGRAPHY ANGIOGRAPHY WITH INVASIVE ANGIOGRAPHY FOR THE DETECTION OF LUMINAL STENOSIS
Native Coronary Arteries (Figs. 21-4 and 21-5)
The initial results of coronary CT angiography with EBCT were encouraging but also demonstrated the limitations of the technology. Achenbach et al9 reported a sensitivity and specificity of 92% and 94% for EBCT angiography compared to invasive coronary angiography for the detection of a luminal obstruction greater than 50% in 125 patients. However, 25% of the coronary segments were not evaluable, owing to technical limitations such as motion artifacts, calcium overlay, high image noise, and so forth. Similar results were obtained with 4-slice MDCT technology. Leber et al.10 reported a sensitivity and specificity of 82% and 96% for 4-slice MDCT with 25% to 30% nonevaluable coronary segments, and similar results were also shown by other investigators.11 A recent meta-analysis, including 22 studies of 4-slice MDCT versus invasive angiography, yielded a sensitivity of 87% and specificity of 87% on a per-vessel basis and 91% and 83% on a per-patient basis, respectively.12
The newer generations of MDCT scanners, starting with the 16-slice MDCTs, have shown improved accuracy compared to 4-slice MDCT. Each advance in technology with increased spatial and temporal resolution has been accompanied by improved results. Better spatial resolution leads to improved ability to assess coronary stents and calcified coronary artery segments (decreased partial volume effect) and improved ability to assess smaller arteries and side branches. As discussed, 16-slice MDCT typically has a spatial resolution of 0.7 mm and a temporal resolution of about 200 ms,13 whereas 64-slice MDCTs typically have a spatial resolution of 0.5 mm and a temporal resolution of 165 ms,14 except for the dual-source 64-slice MDCT that has a temporal resolution of 83 ms.15 These technical characteristics need to be compared to those of invasive angiography with a spatial resolution of 0.2 mm and temporal resolution of 5 to 20 ms.13
A number of relatively small studies compared 16-slice16–29 and 64-slice MDCT30–47 to invasive coronary angiography as the gold standard for detection of obstructive coronary artery disease (CAD) defined as either =50% or =70% luminal artery stenosis. These studies had limitations: they tended to be small (most enrolled less than 100 patients) single-center studies and involved mostly men. To date, there have been three multicenter trials comparing 64-slice MDCT to invasive coronary angiography.48–50 The published estimates of accuracy are somewhat variable because of the use of different scanning protocols and different hardware. Many of the earlier studies excluded unassessable coronary artery segments from statistical analysis, which resulted in artificially elevated measurements of accuracy.12 Most studies included only coronary segments over 1.5 to 2 mm in diameter, although a few evaluated all segments, including small side branches, leading to lower estimates of accuracy. Finally, some of the investigators reported results only on a coronary artery segment or vessel basis, rather than on a patient basis. A segment-by-segment analysis obviously provides more statistical power for the investigation, but a patient-based analysis tends to be more relevant to clinical practice and to the current use of CTA, which revolves around the decision as to whether a patient should be sent to the invasive catheterization laboratory or not.51
Three meta-analyses summarized several studies comparing the diagnostic accuracy of coronary MDCT angiography versus invasive angiography as the reference standard.12,51,52 Vanhoenacker et al.12 included 22 4-slice MDCT studies, 26 16-slice studies, and 6 64-slice studies. Each study in this meta-analysis assessed the ability of MDCT angiography to detect =50% coronary stenosis on invasive angiography. Analyses were performed on three levels: per patient (1474 total patients), in which the presence of any stenosis in any coronary artery was considered a positive result; per vessel (2692 vessels); and per coronary artery segment, based on the American Heart Association approved 15-segment model (30,775 segments). On a per-patient basis, the 4-slice MDCTs, 16-slice MDCTs, and 64-slice MDCTs demonstrated the following sensitivity and specificity: 91% and 83%, 97% and 81%, and 99% and 93%, respectively. Thus, with an increasing number of detectors, the diagnostic performance of MDCT for detection of obstructive CAD seems to have increased. Additionally, there were fewer unassessable segments with 16- and 64-slice MDCT in comparison to 4-slice MDCT.
A second meta-analysis51 comparing 4-, 8-, 16-, and 64-slice MDCT to invasive angiography reached similar conclusions. In this analysis, the authors used 41 single-center studies published between 1997 and 2006 and analyzed data per patient (2515) and per segment (21,821). Diagnostic accuracy improved with each newer generation of scanner. On a per-patient basis, 4- and 8-slice MDCT (combined analysis) showed a sensitivity and specificity of 97% and 81%, respectively, 16-slice MDCT had a sensitivity and specificity of 99% and 83%, and 64-slice MDCT had a sensitivity and specificity of 98% and 92%.
In the third meta-analysis,52 the authors compared 64-slice MDCT alone to invasive angiography. Twenty-seven studies including 1740 patients were reviewed, including four studies with patients who had undergone coronary artery bypass grafting (CABG) and five studies of patients with coronary stents. Of the 18,920 coronary segments, only 4% were unassessable and thus excluded from analysis. A positive study by MDCT or invasive angiography was defined as =50% coronary stenosis. For stenoses in the native coronary artery on a per-patient basis, 64-slice MDCT showed a sensitivity of 97.5%, specificity of 91%, positive predictive value (PPV) of 93%, and negative predictive value (NPV) of 96.5%, consistent with findings in the other two meta-analyses. For post-CABG patients, sensitivity was 98.5%, specificity 96%, PPV 92%, and NPV 99%. Accuracy was considerably less for detection of in-stent restenosis, with a sensitivity of 87%, specificity 96%, PPV 83.5%, and NPV 97%.52
The more recent prospective multicenter trials conducted utilizing 64-slice CT, however, did not completely confirm the very optimistic results reported in the earlier meta-analyses. Budoff et al. compared coronary CTA using 64-slice MDCT to invasive coronary angiography in 230 patients.48 As reported in the meta-analyses, this study showed a high NPV (99%) of coronary 64-slice MDCT for the detection of =50% or =70% coronary luminal stenoses, but a lower specificity and PPV (83% and 99%, respectively, for both per-patient and per-vessel analyses). The authors further noted a substantial decrease in specificity of MDCT in the presence of a coronary artery calcium (CAC) score over 400. The study by Meijboom et al. again illustrated the high NPV of 64-slice MDCT (97% and 99% for patient and segmental analyses) but also the lower than expected specificity and PPV of 64-slice MDCT (64% and 86% for patient and 90% and 47% for segmental analyses).49 In this study of 360 patients with stable or unstable angina, the authors reported a high false-positive rate for MDCT: 41 patients with only mild CAD on invasive angiography were classified by MDCT as having obstructive CAD. However, the investigators did not exclude any coronary segment from evaluation, and heavily calcified segments were considered to harbor obstructive stenoses. Finally, Miller et al.50 reported the results of a nine-center study conducted with Toshiba 64-slice scanners involving 291 patients. Patients with a CAC score over 600 and vessels with a diameter less than 1.5 mm were excluded. The overall area under the curve for detection of more than 50% stenosis by MDCT was 0.93 for patient and 0.91 for vessel analyses. Although the specificities were high (90% and 93%), the NPVs were lower than previously reported (83% and 89%) for both patient and vessel analyses.
Dual-source 64-slice MDCT (Siemens, Forcheim, Germany) has improved temporal resolution, with fewer motion artifacts,53 and the initial clinical experience has shown excellent diagnostic performance of this scanner.4–6
Finally, the new 320-slice MDCT scanner (Toshiba, Tochigi-ken, Japan) has undergone a very initial evaluation.54 The attractiveness of this technology is the potential to limit radiation exposure by allowing whole-heart coverage with a single rotation, although this cannot be obtained for high heart rates, as explained earlier in this chapter. In a study of 40 patients, only one of 1166 coronary artery segments was unassessable, and 89% of the segments showed excellent image quality. Four of the 40 patients underwent invasive angiography. There were seven coronary stenoses =50% noted on 320-row MDCT, and all were confirmed by invasive angiography.54 The average radiation dose was 6.8 ± 1.4 mSv for the single-rotation protocol, although several patients required more rotations, with a substantial increase in radiation dose.
In aggregate, the various studies conducted so far, with the exception of one outlying study showing an NPV of only 83%,50 have consistently shown a high NPV of CTA (in every other case >97% and often close to 100%). It is therefore appropriate to assume that at this stage of development, CTA is especially useful to exclude the presence of obstructive CAD, rather than conclusively demonstrating its presence. In fact, as illustrated by the most recent randomized studies,48,49,50 MDCT does appear to carry a significant false-positive risk for prediction of obstructive luminal disease on invasive angiography.
Coronary CTA for Evaluation of Bypass Grafts (Fig. 21-6)
Coronary CTA is useful for the evaluation of bypass grafts. Saphenous vein grafts are larger than coronary arteries and less mobile, making them easier to image. Arterial grafts are smaller in caliber than vein grafts but can be evaluated accurately by coronary CTA. Coronary CTA has shown excellent diagnostic performance for the evaluation of coronary grafts compared to invasive angiography, with 64-slice MDCT being more accurate than 16-slice MDCT.36,55–62 Several studies have quoted a sensitivity and specificity of 100% for evaluation of patency of the body of the grafts. Difficulty can arise, however, in evaluation of the anastomotic site and of the native coronary arteries distal to the anastomosis. A clear visibility of the anastomotic site to the native coronary artery is often hampered by the presence of surgical clips that cause significant image artifact. The distal segments of the native coronary arteries after the anastomosis are often small, diseased, and at times heavily calcified. These factors limit the ability of CTA to accurately detect for full spectrum of disease in patients submitted to CABG. Another potentially useful application of coronary CTA is planning for repeat bypass surgery, as shown in a publication by Gasparovic et al.63 The clear three-dimensional (3D) reconstruction of the chest cavity and visualization of the course of previous grafts, mammary arteries, location of an aortic aneurysm with respect to other vital organs, and so forth, provide an accurate in-space orientation and may be of great aid to the surgeon planning an often difficult and at times dangerous reoperation.
Coronary CTA for Evaluation of Coronary Stent Patency (Fig. 21-7)
In-stent restenosis occurs at a relatively high rate, and patients with prior stent placement comprise a good proportion of patients referred for invasive coronary angiography. Therefore, the development of a noninvasive modality that can accurately detect in-stent restenosis would be very clinically useful. Coronary stent evaluation, however, continues to be limited with coronary CTA. The main problem encountered is the “blooming artifact” (see artificial overexpansion of the borders of the stent in Fig. 21-7) caused by the high-density material of the struts that makes assessment of stent lumen patency difficult. The size of the stent64 and the coexistence of calcification in the vicinity of the stent further complicate the performance of diagnostic-quality CTA imaging. Some improvement in diagnostic quality can be obtained by reconstructing angiographic images with a sharper kernel, as shown in Figure 21-7. Naturally, the evaluation of large stents, such as those in the left main coronary artery, is more accurate.65,66
In an earlier study, 64 patients with 102 stents were assessed with 64-slice MDCT, and the results were compared to those of invasive quantitative coronary angiography.67 Only 58% of the stents were assessable, and these were larger than the stents that could not be accurately viewed (average diameter 3.28 versus 3.03 mm; P = 0.0002), yielding an overall sensitivity and specificity of 50% and 57%, respectively. For the assessable stents, the sensitivity and specificity rose to 86% and 98%, respectively. The authors further reported that paclitaxel-eluting stents (Boston Scientific, Maple Grove, MN) with 0.13-mm strut thickness were more likely to be assessable than sirolimus-eluting stents (Cordis Corporation, Miami Lakes, FL) with 0.14-mm strut thickness (P < 0.001 for frequency of assessability). Two more recent publications addressed the ability of 64-slice MDCT to establish the diagnosis of in-stent restenosis.68,69 Cademartiri et al.68 evaluated 182 patients who had received 192 stents larger than 2.5 mm in diameter and reported that 7.3% of the stents could not be evaluated. For the remaining stents, 64-slice MDCT demonstrated excellent test characteristics as follows: sensitivity 95%, specificity 93%, PPV 63%, and NPV 99%. Schuijf et al.69 performed a similar analysis in 50 patients who had received 76 stents (2.25 to 4.0 mm in diameter and 8 to 33 mm in length); of these, 14% could not be evaluated, mostly due to motion artifacts (mean heart rate in patients with nonevaluable stents versus evaluable stents: 72+9 versus 55+2; P = 0.002). In the remaining 56 stents, 64-slice MDCT demonstrated both a sensitivity and specificity of 100%. The authors further reported that nonobstructive disease within the stent was clearly diagnosable in 71% of the cases. Dual-source 64-slice MDCT also been evaluated in a recent study of 35 patients with 48 stents.70 All 48 stents were assessable by dual-source MDCT that showed a diagnostic performance versus invasive angiography as follows: sensitivity of 100%, specificity of 94%, PPV 89%, and NPV 100%. However, 67% of the stents were over 3 mm in diameter.
CORONARY COMPUTED TOMOGRAPHY ANGIOGRAPHY: COMPARISON WITH NUCLEAR MYOCARDIAL PERFUSION IMAGING
In an early study, 114 patients at intermediate pretest likelihood of CAD underwent CTA (mostly by 64-slice MDCT) and MPI.71 Of the 114 patients, 58 also underwent invasive angiography, based on the results of imaging studies and clinical data, once again introducing a bias due to the presence of an abnormal test guiding the performance of the subsequent test. All patients with a normal CTA had a normal invasive angiogram, and 90% had a normal MPI, emphasizing the ability of a normal CTA to rule out obstructive CAD in an intermediate-risk population. All patients with obstructive or nonobstructive disease on CTA had CAD on invasive angiography, but only 59% had an abnormal MPI. Of the patients with obstructive CAD on CTA, 82% had obstructive disease on invasive angiography, and 50% had an abnormal MPI. Di Carli et al.,72 Hacker et al.,73 and Gaemperli et al.74 reached the same conclusion: There is often a substantial discrepancy between severity of stenosis assessed by CTA and MPI results. Lin et al.75 proposed a method for improving detection of perfusion abnormalities in patients with atherosclerotic disease on CTA. Taking advantage of the ability of CTA to detect the presence and extent of obstructive and nonobstructive atherosclerosis along the coronary arteries, the authors devised a variety of scores based not only on the severity of stenosis but also the location, extent, and composition of all visualized plaques. They utilized data from 163 patients submitted to sequential CTA and MPI, and were able to show that a score based on the sum of all stenoses, or one based on the presence of mixed plaque (calcified and noncalcified), as well as the proximity of plaques to the coronary ostia, predicted an abnormal result on MPI with high accuracy. Hence, plaque location and morphology may provide additional data that improve the physician’s ability to predict based on abnormal MPI results.
An elegant method to potentially overcome the partial limitations of both CTA and MPI and improve the diagnostic accuracy of these tools is image fusion. The existence of hybrid imaging equipment, such as PET/CT and SPECT/CT scanners, and the implementation of postprocessing software to merge data obtained from separate MPI and CTA equipment allow superimposing the coronary artery tree over perfusion maps of the left ventricle (Figs. 21-8 and 21-9).76,77 Fusion imaging appears to provide a more precise co-localization of perfusion defects in a specific area of the myocardium with coronary artery stenoses, and to improve the overall diagnostic accuracy of either tool taken separately.
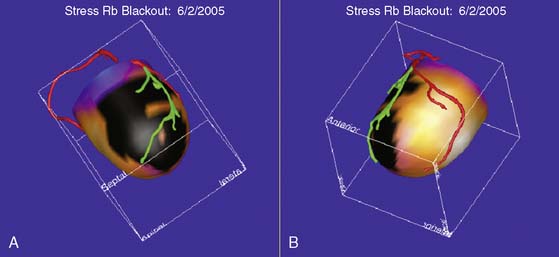
Figure 21-9 Fusion of SPECT and computed tomography angiography images shown in Fig. 21-8, in a patient with single-vessel coronary artery disease. In the fused display, the black area identifies a region of myocardial hypoperfusion during stress. The segment of the left anterior descending coronary artery rendered in green identifies the area of disease seen on CT angiography.
The preceding discussion clearly demonstrates that MPI and CTA provide complementary information, since a stenotic lesion on CTA or invasive angiography is not necessarily associated with a hemodynamic abnormality, and an abnormal MPI is not necessarily accompanied by a critical luminal stenosis on CTA. An abnormal CTA in the presence of a normal MPI, however, indicates the presence of subclinical atherosclerosis that should prompt more aggressive medical therapy even in the absence of hemodynamic alterations. The discrepancy between severity of stenosis on CTA and invasive angiography (described in the first section of this chapter), as well as the discrepancy between severity of stenosis on CTA and inducibility of myocardial ischemia on MPI, should not come as a complete surprise. Indeed, it is important to remember that with CTA, the clinician identifies more than the mere luminal stenosis of a vessel, as can be seen with invasive angiography. In fact, with CTA it is possible to identify the presence of outward and inward vessel wall remodeling, which increases the difficulty of clearly assessing the severity of luminal restriction. Furthermore, the presence of mere stenosis does not necessarily establish the presence of flow abnormalities. Neither invasive coronary angiography nor coronary CTA correlate well with the assessment of the hemodynamic significance of coronary stenoses by intracoronary fractional flow reserve measured by Doppler flow wires.78,79 Finally, as said in other sections of this chapter, the presence of dense calcification causing blooming artifact (see Fig. 21-5) and the occurrence of motion artifacts render the interpretation of severity of stenosis very difficult. All of these factors mitigate the probability of a perfect match between anatomic and functional imaging.
Nicol et al.80 addressed the question of what type of CTA results should prompt a referral to MPI. In a study of 52 symptomatic patients with low to intermediate pretest probability of disease, an abnormal MPI result was observed more often (86% versus 50%) in subjects with a CTA stenosis =70% than =50%. They concluded that CTA lesions narrowing the coronary lumen =70% should be considered “hemodynamically significant,” and patients hosting this type of lesion should be referred for invasive angiography. Since the NPV of a CTA stenosis less than 50% was 100% for an abnormal MPI, no further testing should be recommended for these patients. Further MPI testing, however, should be considered for patients with stenosis severity graded as 50% to 69%. Similarly, Sato et al.81 performed 64-slice CTA and MPI in 104 lean Japanese patients; once again the probability of an abnormal MPI was significantly greater for CTA stenosis =80% (PPV: 86%) compared to lower degrees of stenosis. It should be noted that both investigator groups (Nicol and Sato) emphasized ischemia detected by MPI as the primary target of investigation for CAD, a viewpoint that clinicians interested in the detection of preclinical coronary atherosclerosis may find arguable and oppose.
MPI and CTA have been compared in the setting of acute chest pain in the emergency room. In low-risk patients, the accuracy of CTA appears to be at least as good as MPI for the diagnosis of acute coronary syndrome (ACS).82 In one study, a low-risk population of 85 patients with chest pain, negative serial cardiac enzymes, and normal ECG were submitted to both rest/stress MPI and CTA (64-slice MDCT). Patients with a reversible defect on MPI or =50% stenosis on CTA were referred for invasive angiography. The endpoints were accurate identification of greater than 70% stenosis by invasive angiography or a major adverse cardiac event within 30 days. Seven patients met a primary endpoint (all > 70% stenosis by invasive angiography). Sensitivity and specificity of MPI for predicting an endpoint were 71% and 90%, respectively, while sensitivity and specificity of 64-slice MDCT were 86% and 92%. In a similar emergency room setting, Goldstein et al.83 randomized 99 low-risk patients to 64-slice CTA and 98 similar patients to MPI at a single medical center. The patients in whom CTA showed minimal or no disease were discharged home; if CTA showed over 70% stenosis in one or more vessels, they were sent directly to invasive angiography, and if the lesion was determined to be intermediate, they were referred for MPI. A standard protocol was followed for the patients referred to MPI first. Among the CTA patients, 68 were immediately discharged home, 8 were referred for invasive angiography, and 24 to MPI. Patients were managed equally well in the two imaging arms, and there were no untoward events at 6 months in either group. In the CTA group, however, the final diagnosis was reached in a significantly shorter time (3.4 versus 15 hours; P < 0.001), with a cost saving of about $300 per patient, and the patients required fewer recurrent evaluations for chest pain. It would appear, therefore, that in the emergency room setting, CTA may be an acceptable alternative to MPI in patients at low pretest likelihood of disease. Of interest, in a matched-cohort observational study of patients with new-onset chest pain undergoing coronary CTA or MPI, the 2-year mortality rate based on severity of CAD as assessed by coronary CTA was not significantly different from the rate based on severity of ischemia as assessed by MPI.84 This isolated outcome study suggests that the noninvasive assessment of severity of anatomic stenosis or hemodynamic impediment to blood flow may have similar prognostic implications. Obviously, such data will need to be confirmed in larger prospective studies, but they constitute interesting hypothesis-generating information. Cury et al. recently reviewed the use of coronary CTA in the emergency department setting (Table 21-1).85 This review confirmed the high NPV of CTA for the exclusion of acute coronary syndromes in patients at low to intermediate pretest probability of disease.
CORONARY COMPUTED TOMOGRAPHY ANGIOGRAPHY TO PERFORM MYOCARDIAL PERFUSION IMAGING AND ASSESS VIABILITY
The option to utilize CTA to evaluate myocardial perfusion, infarct size, and viability has been recently explored. Although MDCT can be used for these applications, there is a substantial difference between CTA and MPI; with the former, one can obtain mostly perfusion information (i.e., presence or absence of blood flow in the coronary arteries and into the myocardium), the latter provides information on cellular and molecular function, which is, of course, dependent on coronary blood flow. During the arterial phase of CT angiography, reduced contrast enhancement may be due to severe epicardial coronary stenosis or occlusion, obstruction of the microcirculation, or myocardial scar. Chronic myocardial infarction can usually be identified as an area of reduced myocardial enhancement, but wall thickness and abnormal wall motion, differentiation of severe stenosis, acute myocardial infarction, or microvascular obstruction are problematic on resting CTA angiography. One of the major limiting factors for CTA is the rapid fading of contrast from the myocardium. MPI imaging is an “equilibrium imaging” acquired over several minutes, while CTA perfusion imaging is an instantaneous form of myocardial imaging, and the fleeting appearance of iodine contrast hampers the clear distinction of hypoperfused myocardium, normally perfused myocardium, and the blood pool. Pharmacologic stress testing prior to CTA to improve detection of myocardial ischemia with this tool has been applied in both animal86 and human experiments.87 George et al.86 created coronary artery stenoses in the LAD of eight anesthetized dogs and performed rest and adenosine stress CTA. They then compared the myocardial signal intensity (measured in Hounsfield units [HU]) of ischemic and remote nonischemic regions and measured myocardial blood flow (MBF) with infusion of microphages. They found a close correlation between MBF and myocardial signal intensity, both in ischemic and distant regions. Kurata et al.87 performed rest and stress CTA in 12 patients. All 12 patients were also submitted to MPI and 9 of them to invasive coronary angiography. Adenosine triphosphate (ATP) stress CTA and MPI showed an agreement of 83% (P < 0.05 for concordance), with only a few discordant myocardial segments between the two techniques. Hence, it appears that stress CTA may perform fairly well for detection of myocardial ischemia. However, the performance of stress CTA implies not only a double exposure to a considerable amount of radiation but also a double dose of iodine contrast, rendering this technique unattractive at the current state of development. An interesting application of CTA for myocardial perfusion was recently proposed by Ruzsics et al.53 In 35 patients with chest pain, the authors performed CTA utilizing a dual-source 64-slice MDCT (Siemens, Forcheism, Germany) with each x-ray tube operated at different x-ray energy spectra. The aim of the authors was to obtain simultaneous diagnostic information on coronary artery stenosis and myocardial perfusion from the same CT acquisition without the need to repeat two separate studies. The patients were also submitted to invasive coronary angiography and MPI for comparison. CT demonstrated an accuracy of 92% to detect both coronary artery obstructive disease and myocardial perfusion abnormalities (Fig. 21-10). Since dual-energy imaging will become available in several CT systems in the near future, these findings suggest that the presence of perfusion defects may enhance the diagnostic accuracy of CTA for coronary stenoses, often hampered by the presence of severe artifacts (as discussed elsewhere in this chapter).