Chapter 8 Corneal Diseases
Introduction
Ultrasound biomicroscopy (UBM) systems are suitable for imaging of virtually all aspects of anterior segment including the cornea, anterior chamber, iris and iridocorneal angle, ciliary body and lens (Chapter 4). Ultrasound is therefore applicable to a host of corneal disorders. Although anterior segment examinations are most commonly performed with a fluid-filled scleral shell, they may also utilize a water bath or a membrane-enclosed tip applied to the eye after topical anesthetic. A gentle through-the-lid approach is most commonly used in cases of trauma, albeit at the cost of reduced sensitivity due to attenuation by the lids (Chapter 3).1,2
Pavlin et al were the first to describe UBM imaging of the cornea.2,3 Diagnostic uses in the setting of corneal pathology include congenital corneal opacification, edema,4 keratoconus,5,6 dystrophies,7 corneal scars,8,9 trauma,10 and corneal keloid.11
The earliest commercial UBM system from Zeiss-Humphrey (later Paradigm Medical Industries, Salt Lake City, UT, USA) consisted of a handheld 50 MHz probe that allowed unparalleled non-invasive imaging of anatomic areas of interest throughout the anterior segment. Later generation handheld systems from several manufacturers offer higher scan rates and more compact probes.1 An automated high frequency ultrasound system developed at Cornell University and commercialized as the Artemis-2 (ArcScan) couples a disposable water bath interface and an arcuate probe scanning pathway that approximates normality between the ultrasound beam axis and the corneal surface to facilitate corneal biometric analysis with sufficient scan width to visualize the anterior segment from sulcus to sulcus (Chapter 6). The Artemis very high frequency digital ultrasound system (bandwidth 10 to 60 MHz) has advantages over analog processing systems in its ability to consistently detect internal corneal interfaces due to the higher signal-to-noise ratio between the interface echo complex and the surrounding tissue. These sensitivity gains and a more systematic measurement approach favor excellent quantitative repeatability for small ocular structures such as corneal sublayers compared to UBM.12 Optical coherence tomography (OCT), an interferometric technique first described by Huang et al in 1991,13 has also been shown to be capable of detecting corneal sublayer interfaces such as the laser in-situ keratomileusis (LASIK) flap in the early postoperative period.14 A limitation of OCT is its inability to image the ciliary sulcus through the iris.
Cornea
Normal cornea
Previous studies have shown that the corneal imaging is best conducted with 80–100-MHz probes because the axial resolution is directly proportional to the emission frequency. Pavlin et al showed that even with high-frequency probes, the cornea appears to be divided into four layers rather than its true five layers.2,3
The first layer encountered by an acoustic signal is the epithelium, which is approximately 50 µm thick and has a slightly irregular anterior surface. Bowman’s layer is visible under the epithelium as a highly reflective continuous line because of several interfaces within it. It should be noted that these two layers are not always clearly distinguishable with a 50-MHz probe and can be better identified by post-processing of the signal.2–4
With a more regular internal lamellar structure, the corneal stroma appears as a uniform layer with medium to low reflectivity. This is in contrast to the sclera and Bowman’s layer, which are more reflective because of the more irregular arrangement of collagen fibrils. The fourth layer is represented by Descemet’s membrane (DM) and the endothelial monolayer, which cannot be visualized as two distinct layers and therefore appear as a highly reflective continuous line behind the stroma (Figure 8.1).2–4
Congenital corneal opacity
UBM is useful not only for determining the clinical diagnosis in congenital corneal opacification, but also for refining the surgical plan before keratoplasty where keratolenticular and iridocorneal adhesions and other ocular abnormalities such as aniridia and congenital aphakia are detected.15
Nischal et al studied the correlation of ultrasound biomicroscopy and histological findings in cases of congenital corneal opacification.15 In a report of 22 eyes in 13 patients who were diagnosed with Peter’s anomaly, corneal dystrophy, and sclerocornea, UBM findings changed the final diagnosis in 5 of the 13 cases. They showed that the usually contiguous reflectivity of the DM/endothelium layer seen on UBM was instead irregular, a finding confirmed on histology to be due to focal absences of Descemet’s membrane with multilayering of the endothelium in cases of posterior polymorphous dystrophy (PPMD) and absence of endothelium in cases of congenital hereditary endothelial dystrophy (CHED). Similarly, the presence of a hypoechoic layer in the anterior stroma just below the hyperechoic epithelial layer may be indicative of an absent Bowman’s layer with concomitant edema.15 The ability to delineate the zone of endothelial pathology and the presence or absence of iridocorneal adhesions is particularly important given recent advances in endothelial keratoplasty and the potential application of these approaches to appropriate cases of congenital corneal opacification (Figure 8.2).
Corneal edema
Some of the characteristic features of corneal epithelial edema – hydropic intra- and extracellular changes and intercellular vesicles – increase the quantity and intensity of echo interfaces and result in the appearance of a thicker, more reflective epithelium and Bowman’s layer. An increase in the thickness and the reflectivity of the stroma also occurs with the increased spacing of corneal lamellae and appearance of large interlamellar vesicles.2,4
With UBM it is possible to detect the cause of corneal edema in the majority of cases and to plan the correct surgical approach (Figure 8.3).4 UBM can provide particularly useful information in cases of corneal edema related to acute hydrops in patients with keratoconus, bullous keratopathy,16 and total corneal decompensation secondary to iridocorneal touch in iridoschisis (Figure 8.4).17
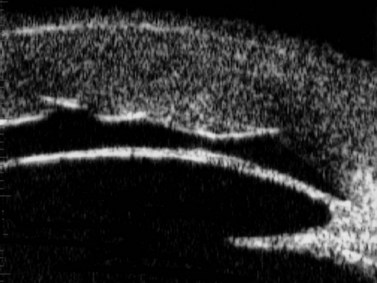
Reproduced with permission from: Heur M, Jeng BH. Anterior segment disorders. Ultrasound Clin 2008; 3(2):201–206.51
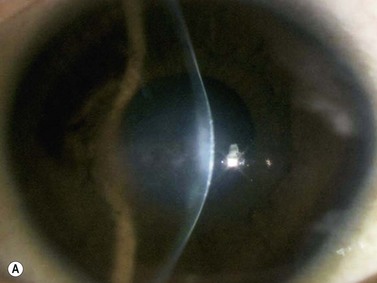
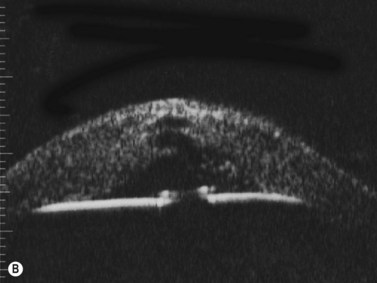
Reproduced with permission from: Nakagawa T, Maeda N, Okazaki N, et al. Ultrasound biomicroscopic examination of acute hydrops in patients with keratoconus. Am J Ophthalmol 2006; 141(6):1134–1136.16
Descemet’s membrane detachments are a common complication of intraocular surgery that can lead to corneal edema. Although spontaneous reattachment occurs in some cases, edema and visual loss can persist and diagnosis is often difficult at the slit lamp microscope in cases of diffuse edema. UBM can be a useful adjunct in visualizing the membrane for diagnosis and for confirming correct suture placement and membrane repositioning during the repair.18,19
Corneal dystrophy
Corneal dystrophies typically are diagnosed clinically based on the specific layer of the cornea affected and characteristic appearances on slit lamp biomicroscopy. UBM of granular dystrophy shows highly reflective hyaline deposits in the superficial stroma and correlates well with OCT findings (Figure 8.5).
Although UBM may be useful to identify affected regions in the setting of corneal dystrophy, Rapuano has presented data that casts doubt upon the accuracy of central stromal lesion depth measurements with a 50 MHz UBM device.7 In 34 eyes undergoing excimer laser phototherapeutic keratectomy (PTK) for a variety of primary or recurrent anterior stromal corneal dystrophies, post-hoc determinations of lesion depth from UBM images did not correlate significantly with the PTK ablation depth.
Corneal infection
UBM can be used to assess the severity of corneal infections. In opacified corneas with bacterial, fungal or amebal keratitis, the extent of corneal thinning and risk of perforation can be assessed serially even when the view at the slit lamp microscope prohibits a view of deep corneal structures. In one case of Acanthamoeba keratitis with acute hydrops, UBM was used acutely to demonstrate Descemet’s membrane rupture and anterior chamber inflammation without corneal perforation, and serially to track resolution of the Descemet’s rupture and infectious keratitis.20