Fig. 7.1
Complement activation and regulation system. The complement system consists of more than 30 molecules and forms three major pathways: the classical pathway (CP), alternative pathway (AP), and lectin pathway (LP). Italic characters mean complement regulatory proteins. C1-INH C1 inhibitor, MBL mannose-binding lectin, MASP MBL-associated serine protease, fB factor B, fD factor D, fI factor I, fH factor H, FHL-1 fH-like protein-1, CFHR proteins complement FH-related proteins
7.2.2 Regulation Mechanism
The complement system is controlled by soluble and cell-bound regulators. The C1 inhibitor (C1-INH), which belongs to the serpin family, is only one strong inhibitor in circulation for early components such as C1r, C1s, and MASP (Fig. 7.2) [7, 8]. AP is controlled by two types of inhibition mechanisms, termed decay-accelerating activity and cofactor-mediated cleavage. Decay-accelerating activity dissociates C3 and C5 convertases. Cofactor activity cleaves C3b into iC3b (inactivated form) and C3f with factor I. Plasma factor H (fH) and its truncated form factor H-like protein-1 (FHL-1), plasma C4-binding protein (C4bp), cell-bound complement receptor 1 (CR1, CD35), decay-accelerating factor (DAF, CD55), and membrane cofactor protein (MCP, CD46) belong to regulators of the complement activation family [9]. Cell-bound regulators, CD59, soluble clusterin, and vitronectin, prevent MAC formation. Carboxypeptidase N (CPN) is a soluble protease that digests anaphylatoxin, C3a, and C5a and inactivates them into C3a desArg and C5a desArg, respectively [4, 8].
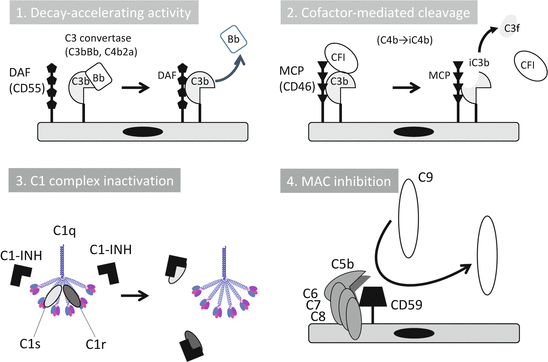
Fig. 7.2
Complement regulation mechanisms. Complement activation cascades are regulated by humoral and cell membrane-bound molecules. DAF decay-accelerating factor, Bb activated split form of factor B, MCP membrane cofactor protein, CFI complement factor I, C1-INH C1 inhibitor (The figure was modified from Kavanagh et al. [38])
7.2.3 Complement Production and Consumption
A detailed analysis of the role of the complement system in the pathogenesis of glomerulonephritis would discriminate a subpopulation of patients from others. It is emphasized that we need to have concern about the production, consumption, and deficiencies of complement components. Complement deficiencies are common genetic disorders. In most cases, heterozygotes produce one-half of the normal plasma level of a specific complement protein. The frequency of deficiency of C1-INH, MBL, C4, and C9 is approximately 1:50,000, 1:10, 1:3 (including partial deletion), and 1:1000 (in Japanese healthy blood donors), respectively [10, 11]. In an analysis of a patient with low CH50, we were first concerned with the possibility of complement deficiencies. Next, we should take notice of overproduction of complement components. The liver is the main source of these components. Because the complement components belong to the acute reactive proteins, like C-reactive protein, infections and chronic inflammation enhance the production of complement components by hepatocytes [12]. Intriguingly, the target organ can also produce the components in situ [13, 14]. For example, C3 is synthesized by kidney resident cells and a single donor kidney is able to produce 5 % of circulating C3 [13, 15]. Actually, we can see the fluctuations of complement levels in the context of many clinical situations. The breakdown product of C3, C3a desArg or, in another term, “acylation-stimulating protein (ASP),” is recognized as one type of adipocytokine. Adipocytes also produce C3, fB, and fD, which are spontaneously activated by the nearby adipocytes themselves. CPN splits C3a into C3a desArg and arginine. C3a desArg has been recognized as an inactivated form of C3a, but energetic studies identified it as a potent stimulator of glucose transport and triglyceride synthesis in adipocytes [16, 17]. Then, the level of C3 increases in an overnutrition state, such as metabolic syndrome, diabetes, or obesity (Fig. 7.3a, b). Body mass index (BMI) and serum complement parameters such as C3, C4, and CH50 have a positive correlation. Especially, the serum level of C3 has a strong correlation with BMI and can fluctuate with insulin resistance. Cryoglobulin-containing serum easily activates CP in test tubes (the so-called cold activation), with the results of the serum level of components and the titer of CH50 being overestimated [18]. Generally, in autoimmune diseases, such as lupus nephritis (LN) and IgG4-related tubulointerstitial nephritis, IC activates CP. In these diseases, IC leads to the consumption of early components of CP in the fluid phase, and laboratory data presents a low serum level of C4 and a low titer of CH50 [19]. Simultaneously, renal depositions of early components of CP, C1q, and C4 were observed. On the other hand, in the cases of post-streptococcal acute glomerulonephritis (PSAGN), most patients presented a low serum level of C3, a low titer of CH50, and glomerular depositions of C3. Thus, it is recognized that AP is the main route of complement activation in PSAGN. From the viewpoint of excess of AP activation, a newly defined clinical entity “C3 glomerulopathy,” which comprises of glomerular lesions with predominant C3 staining, was proposed. Although this category contains cases of membranoproliferative glomerulonephritis (MPGN) and dense deposit disease, many pathogenic abnormalities, such as fH mutations, C3 gene variants, nephritic factors, anti-complement regulatory proteins, were reported [20]. The histological damage of these cases was deduced due to uncontrolled AP activation.
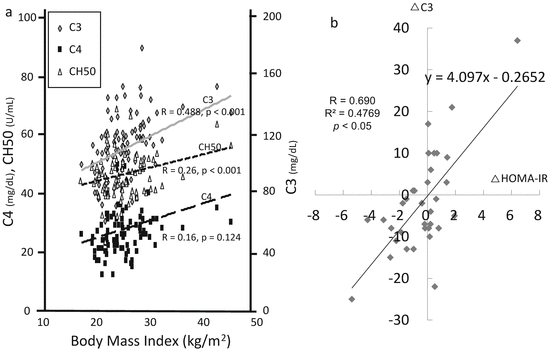
Fig. 7.3
Metabolic impact on the serum levels of C3 in hypertensive and/or chronic kidney disease patients. (a) Correlations between serum levels of complement parameters and body mass index. The serum levels of C3 presented the most significant positive correlation with body mass index. (b) Fluctuations of serum levels of C3 and insulin resistance. Scatter plots of follow-up differences in 35 subjects who were free of dietary restrictions at two time points in the following 6 months. The plots of 24 subjects (69.9 %) were located in the first quadrant or third quadrant and showed a significant positive relationship. Insulin resistance was assessed using the homeostasis model assessment (HOMA-IR) (The figure was modified from Ohsawa et al. [17])
7.3 The Role of Complements in IgA Nephropathy: Fluid Phase
Because serum levels of C3 and C4 fluctuate within the normal range, complement activation in the fluid phase has not been focused on in clinical practice. However, the ratio of the serum level of IgA and C3 (IgA/C3 ratio, more than 3.01) has become a candidate biomarker for the diagnosis of IgAN [21, 22]. In this clinical parameter, a low serum level of C3 contributes to the increase of the IgA/C3 ratio. A cross-sectional study has also indicated that serum C3 levels do not increase despite the elevated levels of other complement components in IgAN at the time of biopsy (Table 7.1). These evidences suggest the possibility that the consumption of serum C3 and MBL via AP and LP might be increased compared with hepatic production of C3 and MBL [23]. Aggregated IgA, polymeric IgA, and chemically deglycosylated IgA1 can activate directly with AP; however, the molecular basis for this difference is not fully understood [24, 25]. To the best of our knowledge, galactose-deficient IgA1 itself is able to activate C3 directly in fluid phase [26].
Table 7.1
Serum levels of complement components in patients with IgA nephropathy
N
|
CH50
|
C1q
|
C4
|
C3
|
C5
|
B
|
P
|
MBL
|
|
---|---|---|---|---|---|---|---|---|---|
(U/ml)
|
(mg/dl)
|
(mg/dl)
|
(mg/dl)
|
(%)
|
(%)
|
(µg/ml)
|
(mg/ml)
|
||
IgA nephropathy
|
50
|
44.0 ± 8.1a
|
13.4 ± 2.8
|
28 ± 11a
|
101 ± 26
|
122 ± 28
|
114 ± 32a
|
32.6 ± 27.0a
|
1.8 ± 1.8
|
Healthy controls
|
50
|
33.5 ± 5.4
|
12.6 ± 1.7
|
21 ± 5
|
106 ± 17
|
112 ± 17
|
95 ± 18
|
21.0 ± 24.0
|
2.1 ± 1.8
|
There is accumulating evidence to support a hypothesis of the occurrence of LP activation in the pathogenesis of IgAN. Under-O-glycosylated IgA leads to the polymerization of IgA [27], and purified polymeric IgA from patients with IgAN can activate LP [28]. On the other hand, prominent ligands for MBL are mannose and N-acetylglucosamine (GlcNAc), and ligands for ficolins are GlcNAc. Although such an under-O-glycosylated IgA1 (N-acetylgalactosamine, GalNAc) from patients with IgAN could potentially interact with plant lectins [29], GalNAc residues cannot be recognized by MBL and ficolins. So far, certain ligands of MBL and ficolin are yet to be defined. Secretory IgA (SIgA) is the first line of defense in protecting the respiratory and intestinal tract and is a credible candidate. Since the N-glycans on the heavy chains of both IgA1 and SIgA2 present terminal GlcNAc and mannose residues, they could be recognized by MBL and ficolins [30].
7.3.1 Fluctuation of Serum Level of C3 and MBL Deficiency
Because IgAN is a chronic glomerular disease and progresses gradually over the long term, additional factors may have a lot of influence on the prognosis. We reported on the fluctuations of the serum level of C3 over a long-term observation (mean observation period, 6.7 ± 2.1 years) in 122 patients with IgAN. In the patients whose renal symptoms, including hematuria, proteinuria, and estimated glomerular filtration rate (eGFR), were improved, the serum level of C3 was significantly elevated (Fig. 7.4) [31]. A subsequent analysis showed that body mass index has a positive correlation with the serum levels of C3 and C4 (Table 7.2) [32]. Thus, the levels and fluctuations of serum C3 and/or C4 might reflect the disease activity and simultaneously, metabolic alteration in patients with IgAN, leading us to believe that we have to pay attention when evaluating the serum levels of complement components.
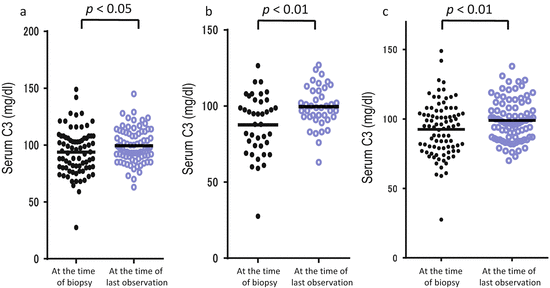
Fig. 7.4
Fluctuations of serum levels of C3 in IgA nephropathy. Serum C3 levels of the 122 patients at the time of biopsy and last observation were collected. The patients whose hematuria (a) and proteinuria (b) had disappeared presented significantly increased serum levels of C3. Also the patients whose estimated glomerular filtration rate (eGFR) had been maintained presented significantly increased serum levels of C3 (c) (The figure was modified from Suzuki et al. [31])
Table 7.2
Relationship of body mass index and clinical data by linear regression models in patients with IgA nephropathy
R
|
p
|
||
---|---|---|---|
Uric acid
|
(mg/dL)
|
0.4427
|
<0.0001
|
Triglyceride
|
(mg/dL)
|
0.4231
|
<0.0001
|
C4
|
(mg/dL)
|
0.4179
|
<0.0001
|
Hemoglobin
|
(mg/dL)
|
0.3540
|
<0.0001
|
C3
|
(mg/dL)
|
0.3575
|
<0.0001
|
HDL cholesterol
|
(mg/dL)
|
−0.3480
|
<0.0001
|
Creatinine
|
(mg/dL)
|
0.3416
|
<0.0001
|
Systolic blood pressure
|
(mmHg)
|
0.3311
|
<0.0001
|
Diastolic blood pressure
|
(mmHg)
|
0.3052
|
<0.0001
|
(n = 193)
|
Functional MBL deficiency is involved in 10–20 % of the healthy population. It is characterized by low levels of functional multimers due to a number of genetic polymorphisms within the coding (codon 54 of exon 1 is the most common in humans and it determines serum concentration and carbohydrate recognition ability) and the promoter regions of the MBL2 gene (Fig. 7.5) [33, 34]. We previously compared the clinical backgrounds between MBL-sufficient patients of IgAN and MBL-deficient patients of IgAN (Table 7.3) [35]. The mean urinary protein and eGFR levels in MBL-deficient subjects were better than in MBL-sufficient subjects. Pirulli et al. reported a similar distribution of polymorphism frequency between healthy volunteers and IgAN patients [36]. Of great interest is whether a difference can be found between MBL deficiency and MBL sufficiency at the onset and progression of IgAN.
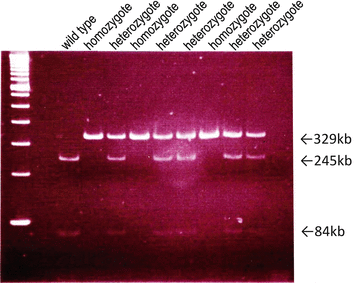
Fig. 7.5
Mbl-2 gene polymorphism of eight of mannose-binding lectin (MBL)-deficient patients. Codon 54 wild-type allele showed two bands (245 bp and 84 bp), the heterozygote allele showed three bands (329 bp, 245 bp, and 84 bp), and the homozygote allele only one band (329 bp) (The figure was modified from Ishii et al. [34])
Table 7.3
Clinical background compared with MBL-sufficient and MBL-deficient patients with IgA nephropathy
MBL sufficient
|
MBL deficient
|
|
---|---|---|
n
|
55
|
6
|
Gender (M/F)
|
22:33
|
3:3
|
Age (y)
|
30.6 ± 8.7
|
26.5 ± 6.7
|
Serum creatinine (mg/dL)
|
0.83 ± 0.30
|
0.71 ± 0.14
|
Estimated GFR (mL/min/1.73 m2)
|
85.3 ± 30.9
|
98.4 ± 13.8
|
Urinary protein (g/g·creatinine)
|
1.45 ± 1.58
|
0.69 ± 0.97
|
History of macrohematuria (%)
|
27.3
|
33.3
|
IgA (mg/dL)
|
316.9 ± 112.9
|
265.2 ± 69.6
|
C3 (mg/dL)
|
97.8 ± 15.8
|
93.2 ± 2.6
|
C4 (mg/dL)
|
21.9 ± 6.1
|
19.8 ± 6.6
|
CH50 (unit/mL)
|
40.1 ± 6.7
|
34.4 ± 3.4
|
7.3.2 Mutation of CFHR Proteins
fH, a strong regulator of C3 convertase formation via the binding of C3b, has binding sites to self-cell surface via glycosaminoglycan and thus is a biphasic regulator of AP, namely, in the fluid phase and on the cell surface [37]. Complement factor H-related (CFHR) proteins (CFHR1, CHFR2, CFHR3, CFHR4, and CFHR5) are not able to bind any complement components, but inhibit binding activity between fH and C3b [38]. The complement factor H (CFH) gene and CFHR1–CFHR5 encoding five CFHR proteins are located in tandem in the regulators of complement activation cluster at chromosome 1q32 [39]. Gharavi et al. identified the gene deletion of CFHR3 and CFHR1 within the CFH locus by genome-wide association study (GWAS) [40]. This CFHR3–CFHR1 homozygous deletion is protective in the pathogenesis of IgAN, C3 glomerulopathy, and age-related macular degeneration [41, 42]. Recently, advanced data was reported, and minor allele A of rs6677604, which is a noncoding single nucleotide polymorphism in intron 11 of the CFHR gene, highly tagged CFHR3–CFHR1 deletion [43].
7.4 The Role of Complements in IgA Nephropathy: Local Tissue
Tomino et al. deduced that the polymeric form of IgA1 is predominantly eluted from the renal tissues of IgAN patients [44]. Tomino and Conley et al. also confirmed the predominantly glomerular deposition of IgA1, rather than that of IgA2 [45, 46]. A subsequent report showed that patients with mesangial deposits of IgA1 and C3c showed no deposits of C4, MBL, and MASP-1. AP is certainly activated in glomerulus [47]. Endo et al. first demonstrated that the glomerular deposition of MBL/MASP-1 occurred in 25 % of cases of IgAN and that this was not observed in the normal kidney and that the frequency was higher than that found in the other forms of glomerulonephritis [48]. Patients with glomerular deposits of MBL/MASP-1 were young, and the duration of the disease prior to renal biopsy was short compared with that in patients without MBL/MASP-1 deposition. Therefore, Roos et al. concluded that the glomerular activation of LP was associated with more severe renal damage, as demonstrated by proteinuria, decreased renal function, and more severe histological findings, such as mesangial proliferation, crescent formation, glomerular sclerosis, and interstitial fibrosis [26]. Because a recent cohort study also confirmed the significance of MBL deposition in IgAN, the glomerular deposition of MBL is a prognostic marker of IgAN [49].
Buy Membership for Nephrology Category to continue reading. Learn more here