10 Chest X-Ray Interpretation
Pearls
• The chest radiograph must be used in conjunction with careful, clinical examination of the patient.
• Nurses should develop a systematic method of reviewing chest radiographs and use that format for examining every film.
• Loss of the cardiac silhouette indicates lung opacification (e.g., pneumonia, atelectasis).
• Remember that free air shifts or rises to superior portions of the chest, whereas free fluid tends to shift or fall to dependent (inferior) portions of the chest.
• Atelectasis represents a volume loss; therefore, structures are often shifted toward the area of atelectasis.
• Pneumothorax and hemothorax are conditions that represent a volume gain; therefore, structures are often shifted away from the area of pneumothorax or hemothorax.
• Because x-rays, CT scans, and fluoroscopy all use ionizing radiation, they should be used judiciously and with a specific indication or clinical question to be answered. (For more information, refer to the Image Gently campaign, available at: http://www.pedrad.org/associations/5364/ig/.)
Introduction
This chapter presents a systematic approach to interpretation of the radiograph and highlights the important factors that the nurse evaluates with this approach. This chapter will not discuss treatment of the problems identified on the radiograph. Evaluation of a chest radiograph may appear to be simple, but is in fact a complex task requiring careful observation, sound understanding of chest anatomy, and knowledge of the principles of physiology and pathology. The value of chest radiographs for critically ill pediatric patients is based on the diagnostic efficacy (the influence of a test result on diagnosis) and therapeutic efficacy (the effect of a test result on clinical management).2 A systematic approach to the review of a chest radiograph will help prevent errors in interpretation and diagnosis.
Definitions
X-rays are a form of short wavelength radiant energy. Images are produced when an x-ray beam is directed through an object to a film cassette. The image produced on the film is determined by the composition, or density, of the object through which the beam passes. The thickness of components of the body and structures in the body vary in their radiodensity, or ability to block or absorb the x-ray beam. To begin the interpretation of a radiograph, the nurse must be able to recognize the different densities that are produced on a chest radiograph and be aware of terms used to describe these differing densities and their radiographic appearances (Box 10-1). This will help differentiate between normal and abnormal findings on the radiograph.
Box 10-1 Glossary of Terms
• Density: Whiteness or any area of whiteness on an image
• Lucency: Blackness or any area of blackness on an image
• Shadow: Anything visible on an image; any specific density or lucency
• Edge: Any visible demarcation between structures of differing density; sharpest edges are created when there is density on one side and a lucency on the other
• Silhouette: Synonym for edge; the loss of an edge constitutes the silhouette sign
• Line: Thin density with lucency on both sides or a thin lucency with density on both sides
If the object is extremely dense, it will block or absorb a significant portion of the beam and prevent it from reaching and reacting with the film; this creates a gray or white shadow on the film. The more x-ray beam an object blocks or absorbs the more radiopaque or radiodense that object is. An object that is not very dense does not block or absorb much of the x-ray beam; it is radiolucent.19 When the beam passes through such an object, most of the beam reacts with the film, and the resultant image on the film will have a dark gray or black appearance.
Most complex objects are not of uniform density; they contain a variety of substances of varying densities, which produce shadows on a radiograph. There are four major categories of radiographic densities that appear on a radiograph; in order of decreasing density these are metal or bone, water, fat, and gas (or air).3 Metal is extremely dense, and it is the most radiopaque of materials. Pure metals block or absorb the entire x-ray beam and produce a bright white shadow on the radiograph. Because bones contain a large amount of calcium, they are nearly as dense and radiopaque as metal and also produce white images on the radiograph. Water and other fluids are fairly radiodense; they block a significant amount of the x-ray beam and have a gray appearance. Body tissues or cavities containing water or fluid (such as the heart) will produce a very light or white image on the radiograph. However, because water and fluids are not as dense as bones, bones and metals will still create whiter (more opaque) images than water. Because fat (including muscle) is not as dense as water, it does not block as much of the x-ray beam and consequently is less radiopaque or more radiolucent than bone or fluid. As a result, the radiograph shadow that fat produces is less dense than that produced by bone or water. Fat is contained in subcutaneous tissue and in some muscle; the x-ray image produced by these fat-containing tissues will have a dark gray or charcoal appearance. Gas or air is the least dense of substances visible on the chest radiograph. Because gas does not absorb much of the x-ray beam, it is radiolucent and produces a black image. Gas or air density is normally seen in the lung fields and in the air-filled stomach. The ability to discern muscle, blood, internal organs, fat, and tissues requires the understanding of normal anatomy.5,7,16,17
All parts of the body contain one or more of the four densities—metal or bone, fluid, fat, or gas. The juxtaposition of body parts or chambers of differing densities will create contrasts on the radiograph. When objects of varying densities are in contact, their borders will be apparent because of the contrasts in their radiographic images, and the difference in their images creates an edge or silhouette.3 When structures are visible on a radiograph, it is easy to evaluate their size, shape, and position. When two objects with similar densities are in contact or in the same plane as the x-ray beam, no spectrum of density is seen; therefore, there is no visible border between the objects on the radiograph.17 This finding may be normal based on normal anatomy. The silhouette sign describes the loss of a normal interface or silhouette, which is suggestive of abnormal tissues densities, often indicating a pathologic condition.6,16 This observation of abnormal density or silhouette sign can often confirm a diagnosis of inappropriate tissue, fluid, or air accumulation. The silhouette sign is commonly applied to the heart, mediastinum, chest wall, and diaphragm.
An x-ray film creates a two-dimensional image of three-dimensional objects as it compresses the image into one plane. As a result, the depth of structures often cannot be appreciated by evaluation of only one radiograph view. Many times, studies must be taken from two or more views so that the images can be compared to better evaluate the relative position of objects.14 Healthcare providers are aware that it is impossible to confirm correct placement of a tracheal tube from an anteroposterior (AP) or a posteroanterior (PA) x-ray alone, because such a film compresses the anterior-posterior dimension. The AP film can be used to evaluate depth of insertion and placement in or displacement from the midline. Only on the lateral view of the chest will it be possible to correctly evaluate the position of the tracheal tube relative to the trachea and esophagus.
An AP chest film is the view most frequently obtained in the critical care unit because it can be taken with a portable x-ray machine. When an AP view is obtained, the film cassette is placed under or behind the child and the child faces the x-ray tube. The child optimally should be in a sitting position; however, if there is difficulty in positioning the child because of acute illness or general immobility, the child is positioned supine. The x-ray beam is then directed from in front of the child through the child and to the film. As a result, the front of the child (anterior aspect) is nearest the x-ray tube when the film is taken. The AP film tends to magnify anterior chest structures, including the heart, because of the divergence of the x-ray beam; this makes it difficult to compare heart size in an AP versus a PA film. Heart size is most magnified in an AP supine film because of horizontal elongation of the ribs, higher placement of the diaphragm, and the impression of decreased lung volume.17
Whenever heart size is evaluated on chest radiographs, it is important to know whether the film was taken with a PA or an AP approach, although the difference is not as significant in infants and small children as it is in older children and adults.19 Changes in heart size, or in the size of any organ, can best be appreciated by comparing two images obtained using the same approach. Characteristically, chest films obtained in the radiology department under controlled conditions are clearer and of better quality than those obtained with a portable machine. Therefore, the films should be obtained in the radiology department whenever practical.
When PA or AP chest films are obtained, evaluation of lateral relationships of structures is possible, but assessment of AP relationships is not possible because of the compression of the image onto a single plane. If a determination of depth is necessary or if localization of a density is required, a lateral film is taken. Lateral chest films are usually obtained as part of a complete radiographic study of the heart and lungs. To obtain a lateral film, the patient is upright, the film cassette is placed on one side of the patient—typically the patient’s left side—and the beam is directed from the other side of the patient. The lateral film is labeled according to the side of the patient that is nearest the x-ray tube. If the patient’s left side is against the film cassette and the right side is nearest the X-ray tube, the resultant film is a right lateral film. Conversely, if the patient’s right side is nearest the film cassette and left side is nearest the x-ray tube, the film is a left lateral film.22
Lateral views allow evaluation of the AP relationships of body structures, but they do not allow determination of their lateral relationships. Therefore, the comparison and evaluation of both a PA and a lateral film provide much more information than either film does separately. An additional advantage of obtaining radiographs in two views is that thin structures (such as fissures) may be visible on only one film if the x-ray beam strikes the structure parallel to its long axis.9,24 When films of the same object are obtained using two different views, there is a greater chance that small structures will be apparent on one of the views.
A lateral decubitus view is obtained with the patient lying on one side or the other. The film cassette is placed at the patient’s back, and the x-ray beam is then aimed horizontally or parallel to the floor.9 A lateral decubitus view can be obtained in the critical care unit, and it is often helpful in determining the presence of air-fluid levels in the chest, such as those be seen when a lung abscess or pleural effusion is present. Positioning has a significant influence on the appearance of air, fluid, and blood vessels within the chest.
Interpretation of film technique
Patient Position and View
The evaluation of a chest radiograph requires knowledge of the exposure conditions of the film, the angle of the x-ray beam, and the alignment and position of the patient. If the x-ray tube is positioned close to the film and the patient, the x-ray image of the patient will be magnified. Conversely, if the x-ray tube is farther away from the film and the patient, the image of the patient will be smaller but sharper. The x-ray tube should be positioned so that the x-ray beam is exactly perpendicular to the plane of the film. If the patient is positioned properly, the x-ray beam will be perpendicular to the horizontal or vertical axis of the patient. If the x-ray beam or patient is slanted, and not perpendicular, a lordotic (oblique) view will be obtained and will show the body or chest at an angle. An oblique view is undesirable if it is obtained unintentionally, because structures farthest from the beam will be shortened while those closest to the beam will be enlarged.22 The child’s nurse should assist the radiology technologist in ensuring that the child is not rotated during exposure of the image. This can reduce the need for additional radiographs, with the associated increase in radiation to the child.
In a lordotic film, the clavicles are projected higher than normal and may be seen to lie above the lung apices, and the ribs appear more horizontal. In addition, it may cause hazy opacification at the lung bases as a result of overlapping soft tissues with magnification of the heart and mediastinum. If the apices of the lungs are not visible above the clavicles on an AP or a PA chest film, a lordotic view has been obtained, and this must be considered when evaluation of lung size and chest expansion is made.17
The alignment and the position of the patient at the time the film is taken also must be considered (Fig. 10-1). To determine whether a patient was positioned appropriately during the radiograph exposure, several structures should be evaluated. This evaluation includes the position of the trachea, curvature of the spine, position of the clavicles, length and symmetry of the ribs, and cardiac landmarks. If the patient is positioned straight and not rotated on the image, the trachea should project in the midline within the upper third of the chest. The spine should be straight with the intervertebral bodies outlined as separate from the disc spaces. With good alignment, the clavicles are of equal size and length. The medial ends of the clavicles should be equidistant from the thoracic spinous processes; they are normally projected over the lung apices and overlap the anterior first ribs. If the clavicles both appear horizontal and of equal size and length, a true AP or PA view was obtained.14 If, on the other hand, one clavicle appears to be smaller than the other (because it was farther away from the x-ray tube at the time the film was taken), or if one clavicle is at a different angle than the other, the patient was probably rotated, and an oblique view of chest structures appears on the radiograph, creating the appearance that one side of the chest image (and one clavicle) is larger than the other. As noted previously, if the apices of the lungs are not visible above the clavicles on an AP or a PA chest film, a lordotic view has been obtained.17
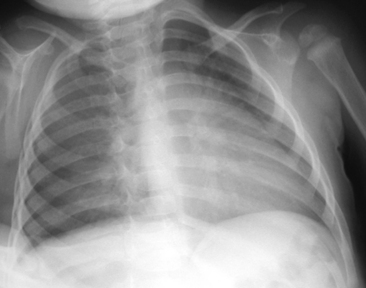
Fig. 10-1 In this chest radiograph the child is rotated to the left. Compared with Fig. 10-2, A, which demonstrates evidence of proper positioning, in this film the proximal ends of the clavicles are not symmetrical in appearance. The patient’s left clavicle appears larger. The ribs on the patient’s right side are more curved posteriorly, and ribs in the patient’s left chest are more elongated with greater visualization of the anterior ribs. The cardiac silhouette is more prominent in the left chest with a normal cardiothoracic ratio, but the heart shape is altered by the rotated view.
Air tends to rise to the highest point within the chest cavity. As a result, in an upright view, free pleural air (such as a pneumothorax) will rise toward the apex of the lung. On a supine film, the highest point in the chest lies adjacent to the heart and mediastinum. When the child is supine for an AP film, free pleural air usually is seen along the diaphragm or the sides of the lung fields. Free air will typically cause increased lucency at these points, often with no lung edges or lung vascular markings visible.9,14 If free air is suggested on a supine view, it can be confirmed by a decubitus view. When the child is positioned on the dependent side opposite to the free air, any air in the pleural cavity will rise to lie along the lateral chest wall.17
Film Technique
When the exposure of the film is not correct, the change in exposure will affect the entire film appearance. If the chest radiograph is underpenetrated (underexposed), all the structures on the radiograph will appear lighter in appearance or diffusely opaque (i.e., too white). The resulting images are difficult to interpret, preventing a detailed view of the mediastinal, retrocardiac, and spinal anatomy. Pathologic findings in the left lower lobe can be missed easily.27 In addition, pulmonary vascular markings will appear to be more prominent, with less distinct borders; thus, they may be mistakenly interpreted to be increased or hazy resulting from interstitial edema. If a film is overpenetrated (overexposed), all the images on the radiograph will be darker or diffusely lucent. The lungs will appear blacker than usual. Overpenetration can obliterate shadows and can make pulmonary vascular markings appear to be reduced.16
If the film is obtained during expiration, the heart will appear larger and less well defined. The lung fields will appear to be hazier and the pulmonary vascular markings more prominent. Hypoinflation of the lung fields can lead to misinterpretation of the images and misdiagnosis of a basilar pneumonia or cardiomegaly. Furthermore, if maximal inspiration is not present, the lungs can appear more congested and the trachea will appear to buckle to the right.21,24 Figure 10-2 illustrates these differences with two views obtained from a normal child during inspiration and expiration.
The child must not move while the film is taken because motion can blur cardiothoracic structures. Blurring of the diaphragm can mimic the appearance of pulmonary infiltrates.19 If the nurse or radiology technologist suspects that the child moved when the film was taken, this should be noted for consideration when the film is interpreted. If excessive motion artifact is present, another film should be obtained.
Interpretation of chest radiograph
Systematic Approach
When an organized systematic approach is used to examine the radiographic image, the nurse will be less likely to overlook significant abnormalities. It is often advisable to initially ignore the most striking or obvious features of a radiograph to avoid overlooking other equally important features. Table 10-1 provides one method of organizing the review of a chest film. Because there are many correct sequences that can be used to interpret the film, the nurse must develop an individual, comfortable style. The most important thing about any approach is that it must include all aspects of the film review. Once a healthcare provider develops a systematic and comprehensive approach to reviewing a film, the provider should use this method every time to ensure that nothing is missed during the review.
Table 10-1 An Organized Approach to Chest Radiograph Examination
Focus of Examination | Aspects of Examination |
Technique | 1. Check alignment and position (check clavicles) |
2. Check degree of inspiration (9 to10 ribs should be visible with full inspiration) | |
3. Check penetration (vertebral bodies should be easily visualized) | |
Soft tissues of chest and neck | 1. Check for subcutaneous emphysema |
2. Examine extrathoracic structures | |
Bony thorax and intercostal spaces | 1. Examine clavicles, scapula, ribs, humeri, and cervical and thoracic vertebrae |
2. Check for fractures | |
3. Evaluate width of intercostal spaces (should be equal) | |
Diaphragm and area below diaphragm | 1. Note clarity of diaphragm and location (check for elevation) |
2. Check for location of gastric bubble (normal: patient’s left) | |
Pleura and Costophrenic angles | 1. Check for presence of fluid or air between pleural layers (look between bony thorax and lung) |
2. Costophrenic angle should be sharp | |
Mediastinum | 1. Borders should be sharp |
2. Check for lateral shift | |
Trachea | 1. Should be straight (may buckle to left on expiratory film if right aortic arch is present) |
2. Check for narrowing or deviation | |
Heart and great vessels | 1. Borders of heart and aorta should be distinct |
2. Obliteration of heart borders is called silhouette sign (see Table 10-2) | |
3. Measure cardiothoracic ratio—normally approximately 0.5 for children and adults (half the width of the chest) | |
Lung fields | 1. Compare right with left |
2. Note presence and location of any opacification; describe it | |
3. Look for air-fluid levels | |
Hili and pulmonary vascularity | 1. Pulmonary vascularity is most prominent at hili |
2. Peripheral pulmonary vascular markings usually are visible in proximal two thirds of lung fields (note if markings are increased or decreased) | |
3. Prominent but hazy pulmonary vascular markings may result from pulmonary edema or pulmonary venous congestion | |
Check location and continuity of all tubes, catheters and wires | 1. Note position of head when evaluating endotracheal tube position |
2. Tip of endotracheal tube should be at level of third rib (1-2 cm above carina) | |
Compare with previous films |
Soft Tissues and Bony Thorax
Examine the soft tissues of the chest wall for evidence of subcutaneous emphysema (air density between the skin and bony thorax), which can result from an air leak around the chest tube or from a penetrating chest wound. In addition, tissue swelling can indicate the presence of an injury. Check the soft tissues of the neck for subcutaneous emphysema. In addition, if the child is intubated, note the position of the child’s head, because flexion or extension of the head can change the position of the tip of the endotracheal (ET) tube.15
Examine the bony thorax and the shape of the chest. Infants and young children normally have round chests with a horizontal orientation of their ribs (see Fig. 10-2). However, older children and adults have chests that are wider than they are deep, and their ribs angle downward from back to front. A round chest in an older child or adult is abnormal and may be the result of chronic respiratory disease with air trapping. As discussed, nine or ten ribs should appear above the dome of the diaphragm if a good inspiratory film has been obtained.
Check the continuity of vertebrae, ribs, scapulas, and clavicles for fractures and bony destruction. The ribs and intercostal spaces should be symmetrical. A fracture often will create a dark line in the bone because of separation of the bone fragments (Fig. 10-3). The vertebral bodies, particularly the cervical vertebrae, should be checked closely for fractures if the child has been admitted following trauma. The presence of multiple rib fractures, especially both posterior and anterior, and in various stages of healing is pathognomonic (positive predictive value of 95% or higher in children 3 years of age and younger) for child abuse.4 Healing fractures often have an enlarged area consisting of cartilage and woven bone creating a callus. Rib notching, or erosion of the underside of ribs caused by enlargement of the intercostal arteries, can be seen in older children with coarctation of the aorta, because the intercostal arteries become enlarged to carry collateral circulation around the area of coarctation into the descending aorta. Abnormalities of the ribs may be noted if the child has had surgery to alter the size or shape of the rib cage. Splinting can produce narrowing of the intercostal spaces on one side of the chest.
Evaluate the width of the intercostal spaces. Following a thoracotomy, muscle spasm or sutures can reduce the width of the intercostal space at and near the site of surgery. In addition, significant atelectasis can cause narrowing of the intercostal spaces on the involved side and widening of the spaces on the noninvolved side.9,14 Hyperinflation, associated with air trapping, will cause widening of the intercostal spaces bilaterally.
Diaphragm and Abdomen
Examine the position and appearance of the diaphragms, including the shape, height, and angles. The patient’s right hemidiaphragm is usually approximately 1 to 3 cm higher than the left hemidiaphragm because the liver lies under the right diaphragm. The diaphragms should appear smooth and domed, with sharply defined costophrenic angles (the angle produced by the lateral downward curve of each hemidiaphragm at its periphery). Unilateral elevation of the diaphragm may be caused by diaphragm paralysis; this may be congenital in origin or result from chest trauma or injury to the phrenic nerve during thoracic surgery (Fig. 10-4