CHAPTER 28 Cervical, Thoracic, and Lumbar Spinal Trauma of the Immature Spine
Epidemiology
Spine injuries involve 1% to 5% of children admitted to trauma centers, making these injuries relatively uncommon in pediatric patients.1,2 The true incidence may be higher given the challenges of obtaining a reliable examination and necessary images in children compared with adults.1–3 A high index of suspicion is warranted in children with polytrauma and children who have sustained a head injury; 40% to 50% of children with a head injury also have a cervical spine injury.3,4
The level of spine involvement and mechanism vary with age. The cervical spine is most commonly involved in all pediatric patients; children younger than 8 years of age are more susceptible to upper cervical spine injuries, and children older than 8 more commonly sustain subaxial cervical spine injuries.5 Thoracic and lumbar spine injuries also increase in incidence as children age and the spine takes on the biomechanical characteristics of an adult.5
Traffic-related incidents account for nearly one third of all pediatric spine injuries; however, other mechanisms of injury are unique to particular age groups.5 In neonates, birth trauma is the most common cause of spine trauma with an incidence of 1 in 60,000 live births.6 Excessive distraction or hyperextension of the cervical spine is thought to be the mechanism, occurring in breach deliveries and large neonates.7 Spinal cord injury should always be suspected in neonates with hypotonia or cardiopulmonary instability. Injuries in infants and young children should raise suspicion for nonaccidental trauma. Imaging studies show avulsion fractures of the spinous processes or multilevel compression fractures in conjunction with rib fractures, long bone fractures, cutaneous lesions, and other characteristic injuries of abuse.8,9 In older children and adolescents, sports-related injuries and diving accidents occur more frequently.5 Recognizing these unique mechanisms of injury helps to raise the suspicion of a spine injury for the treating clinician.
Developmental Anatomy and Biomechanics
Understanding the developmental anatomy allows identification of normal radiographic variants and synchondroses versus true pathology. The atlas and axis undergo unique development, whereas the subaxial cervical spine, thoracic spine, and lumbar spine follow a similar pattern of maturation. The atlas develops from three primary centers of ossification: the anterior arch and two neural arches.10 The two neural arches are visible at birth and develop into the lateral masses. The anterior arch is radiographically visible at birth in only 20% of infants, and in the remaining infants it ossifies over the subsequent year. Measuring the atlanto-dens interval (ADI) is unreliable in infants younger than 1 year old. The synchondrosis between the two neural arches located posteriorly closes by age 3. Ossification between the anterior arch and the two neural arches occurs by age 7.11
The axis (C2) is formed by five primary centers of ossification. The odontoid process is formed by two parallel ossification centers that fuse in utero during the 7th fetal month. A secondary ossification center occurs at the tip of the odontoid, termed the os terminale, arising between ages 3 and 6 years and fusing by age 12 years. The remaining primary centers of ossification are the body and two neural arches. The body typically fuses with the odontoid by age 6 years, but this synchondrosis can persist until age 11. The neural arches fuse anterior to the body by age 6 and posterior by age 3, similar to the atlas. Fractures can occur through the synchondrosis of the odontoid peg, recognized by soft tissue swelling and asymmetrical gapping of this synchondrosis with associated tilting of the dens.12
The subaxial cervical spine (C3-7), thoracic spine, and lumbar spine all develop in a similar fashion. There are three primary ossification centers: the two neural arches and the body. The neural arches fuse to the body anteriorly by age 6 and posteriorly by age 3. Secondary centers of ossification can exist at the tips of the transverse processes, spinous process, and superior and inferior aspects of the vertebral body. These areas ossify in early adulthood and can be mistaken for fracture.10,11 The vertebral bodies of the subaxial cervical spine first ossify posteriorly with progression anteriorly as the child ages. This process replaces a cartilage scaffold with bone, and the vertebral body takes on its characteristic rectangular shape by age 7. Until age 7, it is acceptable to have anterior wedging of the subaxial cervical vertebrae, and this should not be confused with anterior compression fractures. This normal wedging can be profound at C3.
Children with a spine injury are typically divided by age: younger than or older than 8 years old. The biomechanics and injury patterns seen in children younger than 8 years differ from the patterns seen in children older than 8. The spine typically assumes adult characteristics and size by age 8 to 10 years. From birth until age 8, children are usually more susceptible to upper cervical spine injuries. In addition, it is more common for children in this younger age group to have a neurologic injury and subluxation or complete dislocation rather than a fracture.2,13–16 Proposed reasons for the increased incidence of upper cervical spine injuries in this younger age group are listed in Table 28–1. These unique characteristics are important to keep in mind when transporting and evaluating a young child.
TABLE 28–1 Risk Factors in Children Younger than 8 Years Old for Developing Upper Cervical Spine Injuries
Transport and Evaluation
Herzenberg and colleagues17 were the first to note that the transport of young children (<8 years old) on a standard adult spine board tended to cause excessive flexion of the cervical spine. It was noted in all cases that the cervical spine was forced into relative kyphosis because of the disproportionately large head relative to the chest. This flexed position could potentially jeopardize the cervical cord if the mechanism of injury was a flexion force, which is often the case in motor vehicle accidents. To obtain a neutral position, Herzenberg and colleagues17 recommended pediatric spine boards with a cutout for the occiput. Alternatively, a standard spine board can be used with a towel roll placed under the shoulders allowing the head to drop into mild extension, as shown in Figure 28–1.
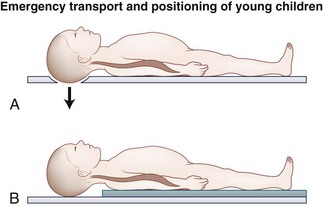
FIGURE 28–1 Emergency transport and positioning of young children.
(From Herzenberg JE, Hensinger RN, Dedrick DK, et al: Emergency transport and position of young children who have an injury of the cervical spine: The standard backboard may be hazardous. J Bone Joint Surg Am 71:15-22, 1989.)
In a subsequent study, Curran and colleagues18 prospectively evaluated these modified transportation methods for achieving neutral alignment of the cervical spine. They obtained supine lateral radiographs of the cervical spine in 118 pediatric trauma patients and measured the sagittal alignment using the Cobb method. They determined that only 60% of the patients were within 5 degrees of neutral alignment using this method. This study highlights the point that very young children may need even more relative chest elevation and as the child approaches age 8 this decreases. This point was confirmed by Nypaver and Treloar,19 who evaluated the height of back elevation needed in children younger than 4 years versus children older than 4 to achieve neutral cervical spine alignment. Children younger than four required 5 mm additional elevation.
Although it is unknown how alignment during transport affects outcome, the authors follow the recommendations of Herzenberg and colleagues17 regarding spine board immobilization, keeping in mind that very young children may need additional elevation to achieve neutral alignment. A clinical guideline is to evaluate the child from the side after immobilization, aligning the external auditory meatus with the shoulder. A well-fitting cervical collar should be placed, transport should occur on a spine board with a cutout for the occiput or alternatively elevating the shoulders, sand bags should be placed on either side of the head to prevent rotation, and the head should be taped to the spine board.
Clinical evaluation of a child thought to have an injury to the spine is often hampered by an inability to obtain an accurate history and a thorough physical examination. The clinician should have a heightened suspicion of spine injury in nonverbal children, owing to a 23-fold increased likelihood of missing a cervical spine injury.20 The mechanism of injury should be taken into account when considering the likelihood of an occult spine injury. Additionally, the presence of associated injuries, including facial trauma, head injuries, thoracic wall injuries, and abdominal injuries, increases the likelihood of spine trauma. Abdominal injuries, particularly injuries of the small bowel, are associated with flexion distraction injuries of the thoracolumbar spine.21 Spinal injuries can occur at multiple levels with noncontiguous fractures occurring in 16%.22
Plain Radiography of Cervical Spine
Imaging of the spine should be done if a child meets any of the following criteria: The child is nonverbal secondary to age or altered mental status, intoxication is present, a neurologic deficit exists or there is a history of a transient deficit, neck pain is present, there is a history of a high-risk mechanism of injury, physical signs of neck trauma or lap belt trauma are present, or painful distracting injuries are present.23 Additionally, unexplained cardiorespiratory instability can be an indication of a high cervical spine injury and should be evaluated appropriately.
The three-view cervical spine series may not be as applicable in pediatric patients with polytrauma, but likely still has a role in patients without polytrauma. The supine lateral cervical radiograph has a reported sensitivity of 79% to 85% in pediatric patients.24 This sensitivity is dependent on seeing all seven cervical vertebrae, including the occipitocervical and cervicothoracic junction. Lally and colleagues25 found that all seven cervical vertebrae were seen in only 57% of children on the initial cervical spine series. The addition of the anteroposterior and open-mouth odontoid views increases the sensitivity to approximately 94% if ideal images can be obtained, which can be extremely challenging in an uncooperative child.26 Buhs and colleagues27 showed that the open-mouth odontoid view did not provide additional information in children younger than 9 that was not already appreciated on the anteroposterior and lateral views. In its place, these authors recommended use of computed tomography (CT) from the occiput to C2.
The use of flexion and extension radiographs during the initial evaluation has come into question. Ralston and colleagues28 disputed the need for these dynamic studies in the acute setting. These investigators had blinded radiologists retrospectively review static and flexion-extension radiographs on 129 children. They found that if the static x-rays were normal, the flexion-extension views would reveal no abnormality. Dwek and Chung29 confirmed these findings, retrospectively evaluating 247 children in whom static and flexion-extension radiographs had been obtained in the trauma setting. They found the flexion-extension view helpful in ruling out an injury in only four patients who were originally thought to have abnormal static films. These dynamic studies are helpful in evaluating for instability after an appropriate time of closed treatment. In the delayed setting, flexion-extension radiographs can confirm either healing and resultant stability or the need for operative treatment.
Interpretation of the cervical spine series requires an understanding of the normal anatomy and the anatomic variants of the immature spine that can mimic trauma. Special attention should be given to the upper cervical spine including the atlantoaxial and atlanto-occipital region given the propensity of injuries to this area in children and the subtle radiographic findings.30 Several methods for evaluating the craniocervical junction using the lateral x-ray have been described. The “rule of 12’s” is thought to be the most sensitive method, whereby the distance between the basion and tip of the odontoid process should be 12 mm or less, and a line drawn parallel along the posterior aspect of the body of C2 including the odontoid should come within 12 mm of the basion. The distance from the tip of the odontoid to the basion is unreliable in children younger than 13 because of incomplete ossification of the odontoid; however, the line drawn parallel to the back of the C2 body and odontoid peg should be less than 12 mm from the basion.22 A gap of more than 5 mm from the occipital condyle to the C1 facet also represents a disruption of the occipitocervical junction.31
The atlantoaxial articulation is best evaluated with the ADI. In children, the normal ADI can be up to 5 mm. If the ADI exceeds 5 mm on lateral flexion and 4 mm on lateral extension, the transverse atlantal ligament is likely disrupted.32 When the ADI exceeds 10 to 12 mm, the alar and apical ligaments have failed with a high risk of cord compression.33 The extent of cord compression at the atlantoaxial joint can be determined using Steel’s rule of thirds,34 which is based on dividing the inner diameter of the C1 ring into thirds. One third of the space should be occupied by the odontoid; one third, by the spinal cord; and one third, by the space available for the cord. When the dens migrates posteriorly by greater than one third of this space, the transverse ligament has been disrupted, and the spinal cord is likely being compressed.
Swischuk35 described the posterior cervical line (spinolaminar line) to help diagnose pathologic angulation and translation in the upper cervical spine. The posterior cervical line is formed by drawing a line from the anterior aspect of the spinous process of C1 to the anterior aspect of the spinous process of C3. The line should pass within 1.5 mm of the anterior aspect of the spinous process of C2 on flexion-extension radiographs; otherwise, a true injury should be suspected.
Many normal anatomic variants should be recognized so as to not be confused with a “true” traumatic injury. A normal finding on extension radiographs in 20% of children is overriding of the anterior arch of the atlas on the odontoid. This finding is incomplete ossification of the dens.36 Another common variant noted on cervical spine x-rays in an immature spine is pseudosubluxation of C2 on C3 and less commonly C3 on C4. Cattell and Filtzer36 were the first to appreciate this normal finding in a study involving 160 pediatric patients with no history of cervical spine trauma. On dynamic radiographs, 46% of children younger than 8 years showed 3 mm of anteroposterior motion of C2 on C3; 14% of children had radiographic pseudosubluxation of C3 on C4.36,37 Based on this study and others, 4 mm of translation is considered normal.6,28,36,38
The absence of cervical lordosis on static lateral x-rays can be a normal finding in 14% of children up to age 16.22 This normal variant can be differentiated from a more ominous sign of posterior ligamentous injury by assessing the posterior intraspinous distance. Each intraspinous distance should not be more than 1.5 times greater than the intraspinous distance directly above and below a given level. The only exception to this rule is the C1-2 intraspinous distance, which can be greater than 1.5 times the distance of the level below. This normal increased mobility is thought to be secondary to the stout posterior ligaments linking C1 to the occiput.39,40
Another challenge unique to the developing spine is differentiating a synchondrosis that has not yet ossified from a true fracture. A synchondrosis typically shows well-corticated sclerotic margins. Another helpful aid in differentiating a subtle fracture from a synchondrosis is evaluation of the prevertebral soft tissues. The retropharyngeal space (at C2) should be less than 7 mm and the retrotracheal space (at C6) should be less than 14 mm in children. In simplistic terms, the retropharyngeal space should be one half the anteroposterior distance of a cervical vertebral body, and the retrotracheal space can be up to a full cervical vertebral body. The retropharyngeal soft tissue can be falsely increased with expiration, such as in a crying child.41 The lateral cervical spine x-ray should be repeated, attempting to obtain the radiograph during inspiration, to determine if a true abnormality exists.
Plain Radiography of Thoracic Spine
Burst fractures are best seen on a CT scan; subtle findings on conventional radiography include interpedicular widening on the anteroposterior projection and small cortical defects at the posterosuperior corner of the vertebral body on the lateral projection. Improper radiographs are the leading cause of missed injury and subsequent neurologic deterioration in large series of trauma patients, supporting the argument for increased use of CT.42,43
Computed Tomography and Magnetic Resonance Imaging of Spine
The use of helical CT has replaced conventional radiography as the screening tool of choice for the adult cervical spine in the setting of blunt trauma.44,45 One concern in children is the exposure to radiation and potential risk of developing thyroid cancer. Some estimates have been four times the radiation dose in the helical CT group compared with conventional radiographs.46,47 CT scan with reconstructions should be used for the correct indications.
There is growing evidence that CT is superior to plain radiography for evaluating the thoracolumbar spine in adults. This evidence has relevance in patients with polytrauma who are being evaluated with a traumagram. These studies, originally targeted for evaluation of the thoracic and abdominal contents, have been shown to be very sensitive at screening for thoracolumbar spine injury. Multiple prospective studies have shown a higher sensitivity for CT (93% to 100%) compared with plain radiography (33% to 74%) with improved interobserver variability.37,48–50 This information can likely be extrapolated to pediatric patients, but further studies need to be performed before CT scans with sagittal and coronal reconstructions replace conventional radiography for thoracolumbar spine clearance.
Magnetic resonance imaging (MRI) is useful for evaluating a neurologic deficit and soft tissue involvement, to help with preoperative planning, and to assist with cervical spine clearance in an obtunded patient. MRI in this age group can be difficult because these young patients often need sedation to prevent movement during data acquisition. MRI should be obtained in patients with evidence of a neurologic deficit, and some investigators would argue that it should be obtained in patients with a transient deficit. A transient deficit can be an indication of a more serious ligamentous and neurologic injury and subsequent need for immobilization.51
In a study of 74 children suspected to have a cervical spine injury based on plain radiography, MRI confirmed the plain radiography diagnosis in 66% and altered the diagnosis in 34%.52 Keiper and colleagues53 obtained MRI in children who had clinical symptoms consistent with a cervical spine injury despite having negative plain x-rays and CT studies. There were 16 abnormal results in 52 children, with posterior soft tissue injury being the most common injury. Four of these patients eventually underwent surgical stabilization, and MRI assisted with preoperative planning. MRI can be especially helpful in clearing the cervical spine in obtunded patients. Frank and colleagues54 showed the effectiveness of MRI at decreasing time to cervical spine clearance, length of time in the pediatric intensive care unit, and length of time in the hospital.
Spine Clearance
Early cervical spine clearance has multiple benefits and helps avoid complications. Cervical collars have known complications, including skin breakdown around the neck, dysphagia, pulmonary complications, increased intracranial pressure, and decubitus ulcers.38,55,56 After a cervical collar has been placed on a child, formal clearance must be obtained before it can be removed. Clinical clearance of the cervical spine has been evaluated using the National Emergency X-Radiography Utilization Study (NEXUS), which was originally developed for adults. NEXUS has been used as a decision-making instrument to determine the need for radiography. The criteria in adults for clinical clearance are absence of neck pain, neurologic symptoms, distracting injuries, or altered mental status (owing to injury, age, or intoxication). If any one of these four criteria is present, the patient is considered to be high risk and must be radiographically cleared. If none of these criteria are present, the collar can be cleared without further imaging.
Application of NEXUS criteria in pediatric patients was studied in a prospective multicenter study. All 30 cervical spine injuries were placed correctly into the high-risk group, and, more importantly, no cervical spine injuries were noted in the low-risk group. Additionally, use of NEXUS criteria decreased pediatric cervical spine imaging by 20%.57 These rules cannot be safely applied in children too young to cooperate with an examination, in children in the presence of a high-energy mechanism, or in children with associated injuries that heighten the suspicion of a spine injury. The Canadian C-Spine Rule has gained popularity in the adult literature, showing a higher sensitivity than NEXUS (99.4% vs. 90.7%) and a higher specificity (45.1% vs. 36.8%), but to the authors’ knowledge it has not been studied in children.58
It has been shown that an efficient, multidisciplinary approach can facilitate rapid clearance of the cervical spine, decreasing average time to 7.5 hours in nonintubated patients and 19.4 hours in intubated patients.23 This rapid clearance is dependent on a system that is safe and user-friendly enough to allow the primary team to perform the clearance. Anderson and colleagues59 evaluated the percent of cervical spines cleared by spine specialists before versus after initiation of a clearance protocol, noting a 60% increase in spines cleared by nonspine physicians without any late injuries detected.
Children with no evidence of injury on plain radiography or CT scan who have persistent pain should remain in the cervical collar with later clearance in the clinic with dynamic radiographs. In the presence of a neurologic deficit or a history of a transient deficit before arrival, MRI of the entire spine should be obtained. If spinal cord injury without radiographic abnormality (SCIWORA) is suspected, the cervical collar should remain in place, spine precautions should be continued, and the patient should be admitted for observation because there can be delayed deterioration.60,61
Clearing the cervical spine in an unconscious patient can be facilitated by a standard protocol. If the patient returns to a normal mental status, the protocol for a conscious patient can be used. There have been reports of delayed neurologic deterioration in patients with altered mental status and unrecognized ligamentous injury that subluxated on mobilization.62 The two modalities available for evaluating ligamentous injury in an obtunded patient include fluoroscopic flexion-extension and MRI. Fluoroscopic flexion-extension examination can be labor-intensive, and in an adult series, it was difficult to visualize the cervicothoracic junction.63 Because of these problems, many investigators believe that MRI is superior for evaluating ligamentous injury.
MRI has been found to be most sensitive for detecting a ligamentous injury at postinjury day 2 or 3.64 Stassen and colleagues65 used MRI within their protocol at a Level I adult trauma center for assisting in cervical spine clearance. All obtunded patients with a negative CT scan of the cervical spine underwent MRI on postinjury day 3 if they could not be clinically cleared. MRI allowed clearance of the cervical collar in 60% of subjects, and there was no delayed neurologic sequela. The remaining patients were treated in a cervical collar for 6 weeks without complication.
The best available evidence indicates that MRI is the most sensitive study for detecting posterior ligamentous disruption and should be the primary study in obtunded patients.66 Equivocal MRI findings can be evaluated further with flexion-extension radiographs, or the physician can forgo further study and treat the patient with a collar for 6 weeks. At the termination of the 6-week period, the patient should undergo a flexion-extension series to ensure adequate healing. Expedient clearance of the thoracolumbar spine in a child with polytrauma is also very important to prevent skin breakdown, respiratory complications, and ileus. At the authors’ institution, the CT traumagram, with sagittal and coronal reconstructions, is used to augment the clinical examination and clear the thoracolumbar spine.
Spinal Cord Injury in Children
The mechanism of spinal cord injury differs depending on age.22 Children younger than 8 years old more often sustain spinal cord insult involving the upper cervical spine, secondary to ligamentous injury often with no discernible bony changes. Children older than 8 have spinal cord injuries with associated fractures owing to biomechanics of the spine that more closely mirror the biomechanics of the adult spine. A thorough neurologic examination should be obtained to determine the level involved and whether the lesion is complete or incomplete. It is important to note whether there is sacral sparing as indicated by sensation at the anal mucocutaneous junction (S4-5 dermatome) on the left and the right side, ability to contract the anal sphincter voluntarily, and deep anal sensation. This sacral sparing indicates continuity of long tracts with improved likelihood for return of neurologic function.67 The Frankel classification and American Spinal Injury Association (ASIA) Impairment Scale have been used in pediatric patients to provide a standardized description of injury.
Damage to the spinal cord has been categorized into primary and secondary injury. Primary injury occurs at the time of the accident leading to structural damage of the neural elements and supporting blood supply. Secondary injury occurs within minutes of the trauma setting off a cascade of events that result in ischemia, increased cell membrane permeability, pathologic electrolyte shift, edema, and production of free radicals.68 This process continues to evolve over the subsequent days, and medical and surgical strategies are directed at mitigating secondary injury. Current treatments of interest include support of spinal cord perfusion, steroids, and early versus delayed decompression.
During transport and initial evaluation, it is crucial to prevent hypotension and to keep oxygen saturations high in patients suspected to have a spinal cord injury so as to help protect the spinal cord blood perfusion.69 Animal and adult studies have shown improved outcome in subjects aggressively managed to prevent spinal cord ischemia.70–72 This practice has been accepted by many pediatric trauma centers and includes admitting patients to the intensive care unit and accurately monitoring volume status and blood pressure. The mean arterial pressure should be maintained at greater than 80 mm Hg for 7 days.
Steroids are stabilizing agents; they act as a powerful anti-inflammatory decreasing edema and scavenging oxygen free radicals. High-dose methylprednisolone is administered to children based on data collected in adults. The recommendations on timing and dose are based on studies performed in patients as reported in the National Acute Spinal Cord Injury Study III.73,74 Methylprednisolone is administered intravenously at a loading dose of 30 mg/kg over 15 minutes followed by 5.4 mg/kg/hr continuous infusion. If administration of the steroid is initiated within 3 hours of injury, the infusion should be continued for 23 hours. If steroid administration is started at 3 to 8 hours after injury, it should be continued for the subsequent 48 hours.
Steroids should not be given if a child presents after 8 hours or if the spinal cord injury is secondary to penetrating trauma. In this setting, there are significant risks with little to no benefit.75 The National Acute Spinal Cord Injury Study III73,74 excluded all children younger than 13 years of age. There are no equivalent data in young children, and in this age group the use of steroids is not evidence based.
The indications for early operative intervention for pediatric patients with spinal cord injury include radiographic confirmation of spinal cord compression in the setting of an incomplete injury or progressive deficit, an open spinal injury, and a grossly unstable spine in patients who are neurologically intact. To the authors’ knowledge, there have been no well-designed prospective studies in pediatric patients evaluating the effect and timing of decompression.76
Functional outcomes depend on the level of injury and whether it is complete or incomplete. Patients with injuries above C4 may be dependent on a respirator, and phrenic nerve pacemakers can be implanted. Patients with C3 lesions can shrug their shoulders and often have neck motion, permitting operation of equipment with sip/puff controls, voice activation, eyebrow or eye blink, and head or chin controls. Patients with C6 lesions are often able to propel a manual wheelchair with the assistance of fusions and tendon transfers. At least partial recovery after a complete spinal cord injury has been reported in 10% to 25% of patients.22,69,75,77 In a series reported by Wang and colleagues,78 64% of patients showed at least partial recovery after spinal cord injury. Of patients with complete injuries, 25% eventually became ambulatory. Recovery was seen up to 1 year after injury.
Children are also better able to adapt to an injury. Anderson and colleagues79 interviewed 161 adults who had sustained a spinal cord injury as a child and found that 64% lived independently, and approximately 50% of patients reported being satisfied with their quality of life. Concerning outcomes from operative versus nonoperative treatment of spine fractures associated with spinal cord injuries, one study showed that patients in the operative group were able to use their wheelchair 5.2 weeks earlier.80 Early activity is crucial in paralyzed patients to avoid pulmonary complications, disuse osteopenia, and complications from insensate skin.67
Complications unique to pediatric patients with a spinal cord injury are susceptibility to development of post-traumatic deformity and growth arrest.81 Causes can be divided into intrinsic, extrinsic, and iatrogenic factors. Intrinsic factors include injury to the vertebral apophyses resulting in abnormal growth and change in biomechanics from altered shape of the vertebral body and loss of posterior ligamentous support. This change in biomechanics can alter the forces on the vertebral apophyses, exacerbating the deformity further.82 Extrinsic factors include weak trunk muscles in the setting of gravity and spasticity with a contracture. Iatrogenic factors include improperly instrumented segments and use of a laminectomy without fusion. The risk of developing a deformity, in the setting of a laminectomy without fusion, is approximately 50%, with a much higher incidence in the cervical and thoracic spine.83 Nonoperative treatment with a brace can help slow the progression of deformity, temporizing the situation and allowing the child maximum time to grow before performing a fusion.84 Performing surgical correction and fusion needs to take into account remaining growth. An estimate of remaining growth can be determined by multiplying 0.7 mm times the number of segments fused times the number of years of remaining growth.85
Posterior fusion in young children has the added drawback of crankshaft phenomenon. This phenomenon occurs with continued growth of the anterior column in the setting of a fused posterior column. With continued anterior growth, the apical vertebral body rotates producing a scoliotic curvature.86 Delaying surgery must be balanced with addressing curves while they are still supple. The goals of surgery include halting progression, obtaining correction, and balancing the spine and pelvis to equalize sitting skin pressure. Indications include a scoliotic curve of greater than 40 degrees and kyphotic curves of greater than 60 degrees. Anterior release may be needed if the deformity is rigid as shown on bending films. Including the sacrum in the fusion construct is recommended if pelvic obliquity exists.
Spinal Cord Injury Without Radiographic Abnormality
The biomechanical differences in the spine of children younger than 8 years place them at risk of SCIWORA.22 This entity was first described by Pang and Wilberger87 in 1982 before the use of MRI. The term SCIWORA was developed to describe spinal cord injuries without overt vertebral column disruption as displayed by conventional x-rays, CT scans, myelograms, and dynamic flexion-extension radiographs. SCIWORA excludes injuries secondary to penetrating trauma, secondary to electrical shock, secondary to obstetric complication, and in association with congenital anomalies. The incidence of SCIWORA in patients with spinal cord injury for ages birth to 17 was reported to be 35% in earlier literature.61 As MRI has become more widely used, the incidence in children with spinal cord injuries is reported to be 3%.5 This change likely reflects an improvement in imaging quality and the ability to detect subtle injuries previously missed, rather than a change in actual injury type. More recently, Bosch and colleagues88 found that recurrent SCIWORA was an uncommon entity, which occurred only in low-energy sports-related injuries, resulting in transient neurologic symptoms, with full recovery in all cases.
MRI is the study of choice for evaluation of patients suspected to have SCIWORA. There are extraneural soft tissue changes and intraneural changes. The changes seen on MRI are secondary to edema and methemoglobin, a processed form of hemoglobin. Edema is seen as isointense on T1 and hyperintense on T2; extracellular methemoglobin is seen as hyperintense on T1 and hyperintense on T2. MRI changes in the extraneural tissues can be detected within hours of injury because the blood is quickly metabolized into a form easily seen on MRI. Intraneural changes can take days to be detectable because of the delayed metabolism of a hemorrhage into a form visible on MRI.89,90 It is recommended that MRI be obtained at the time of presentation to rule out a compressive lesion that needs to be surgically addressed and at 6 to 9 days after injury to improve detection of intraneural injuries related to SCIWORA.61
The recommended duration of immobilization in a brace has been controversial, with the concern for delayed neurologic deterioration or reinjury motivating the recommendation for a brace. Bosch and colleagues88 found that immobilization did not prevent recurrent SCIWORA, and it did not improve the outcomes of primary SCIWORA after instability was properly ruled out. A meta-analysis by Launay and colleagues60 showed that patients immobilized for 8 weeks had a 17% chance of developing recurrent SCIWORA, whereas no patients immobilized for 12 weeks had recurrent SCIWORA develop. Based on this information, it is recommended that children be immobilized for 12 weeks with flexion and extension views of the injured region at 12 weeks to detect instability.
Atlanto-occipital Dislocation
Atlanto-occipital dislocations were previously thought to be rare injuries that were fatal and usually found on autopsy.91,92 More recently, this injury has been identified more frequently with a higher survival rate, particularly among children. Increased survival is possibly due to faster response by emergency personnel, improved cervical immobilization, and faster diagnosis by emergency department physicians.92–97
The atlanto-occipital joint is a condylar joint with minimal bony stability and is stabilized primarily by ligaments. In children, the occipital condyles are less cup-shaped, and the articulation is more horizontal, potentially explaining the higher incidence of dislocation among children compared with adults.30,31,95 Ligamentous stability is provided primarily by the tectorial membrane, the anterior longitudinal ligament, the nuchal ligament, and the paired alar ligaments.
On lateral plain films, the distance between the tip of the basion and dens is known as the dens-basion distance, which is normally less than or equal to 10 mm.98 Additionally, the Powers ratio is the distance from the basion to the posterior aspect of the arch of the atlas divided by the distance from the anterior tubercle of the atlas to the rim of the foramen magnum, which is normally between 0.7 and 1, with a value greater than 1 indicating anterior atlanto-occipital dislocation.99 These traditional radiographic criteria have been called into question because of accuracy, leading some to advocate using CT and MRI for diagnosis.93,94,100 More recently, it has been suggested that a condyle-C1 interval of greater than 4 mm, as measured on a reformatted CT scan, is valuable in identifying atlanto-occipital dislocation.100
Fractures of the Atlas
A fracture of the ring of C1 is a rare injury in children that can occur when an axial load forces the occipital condyles into the lateral masses, resulting in fractures that involve the anterior and posterior rings (Jefferson fracture).101–105 Children differ from adults because they can have plastic deformation of the ring and a single fracture and hinging on a synchondrosis. Instability exists when there is disruption of the transverse ligament. This disruption is determined on the anteroposterior cervical spine film by adding the overhang of the C1 lateral mass relative to the C2 lateral mass on the left and right sides. If the sum is greater than or equal to 7 mm, instability exists and should be treated with traction and a halo or Minerva cast. Cases with sums less than 7 mm can be treated with a well-fitting cervical orthosis.105 Surgical stabilization of C1 fractures is rarely required.
Atlantoaxial Instability
Instability at the level of C1-2 can be secondary to traumatic ligamentous injury or chronic disease processes, including inflammatory diseases, malignancy, bone dysplasias, and congenital craniofacial malformations. Down syndrome is well reported in the literature, and there are other reports of atlantoaxial subluxation associated with Reiter syndrome, Larsen syndrome, juvenile rheumatoid arthritis, Morquio syndrome, and Kniest syndrome.106–112
In traumatic atlantoaxial injury, the transverse ligament is disrupted, resulting in an increased ADI. The ADI is the distance as measured on a lateral cervical x-ray between the posterior aspect of the anterior ring of C1 and the anterior cortex of the dens. Active flexion views may be required to observe subluxation, and CT scans can show avulsions of the transverse ligament. In children, the normal distance is 4.5 mm, whereas in adults it is 3 mm. Acute rupture of the transverse ligament is rare in children, accounting for 10% of cervical spine injuries.113,114 Alternatively, the ligament itself may be intact but can be avulsed from its attachment to C1. Surgical stabilization of C1-2 after reduction in extension is generally recommended, followed by immobilization for 8 to 12 weeks in a halo brace, Minerva cast, or cervical orthosis. To document stability after fixation, flexion and extension views are recommended.
In Down syndrome, the frequency of atlantoaxial instability has been reported to approach 10% to 30% by adolescence.107,115–118 Instability in patients with Down syndrome is attributed to laxity of the transverse ligament and C1-2 joint capsules. Nearly 98% to 99% of patients are asymptomatic but should be followed closely,119–121 with some authors recommending yearly neurologic and radiographic examination.122 Surgery is indicated in symptomatic patients and asymptomatic patients with an ADI greater than 10 mm or with less than 14 mm of space available for the spinal cord on lateral films.123 There is a high reported surgical complication rate for these patients, including the risk of pseudarthrosis, wound infection and dehiscence, adjacent level disease, and neurologic injury.117,124
Surgical fixation for atlantoaxial arthrodesis originated with posterior wire stabilization and structural bone grafting, as popularized by Gallie in 1939125 and Brooks and Jenkins in 1978.126 This technique requires the passage of wires into the spinal canal, with the potential risk of spinal cord injury.127,128 Additionally, a wire construct often lacks sufficient stability129,130 resulting in nonunion rates of 30% even with a halo vest postoperatively.43,50,51,131–133
The C1-2 posterior transarticular screw, or Magerl screw (1986), has a decreased rate of nonunion127,134–138 but carries the risk of injuring the vertebral artery.139 Segmental C1-2 fixation was introduced by Goel and Laheria in 1994140 using a plate and lateral mass screws and then by Harms and Melcher in 2001141 using polyaxial C1 lateral mass screws and C2 pedicle screws with a rod. This approach offers the distinct advantage of being able to insert the screws and then achieve the reduction compared with the Magerl screw technique, in which an anatomic reduction must be achieved before screw insertion. Figure 28–2 shows a 6-year-old boy who had atlantoaxial subluxation.
Atlantoaxial Rotatory Subluxation
Atlantoaxial rotatory subluxation, also known as rotatory fixation if it has persisted for more than 3 months, is a frequent cause of torticollis in children. The most common etiologies are trauma and infection, although congenital and iatrogenic causes also exist. Patients present with neck pain; headaches; and a cock-robin position, in which the head is rotated to one side with some lateral flexion. In acute subluxations, movement is painful and accompanied by sternocleidomastoid spasms. The child often has the ability to make the deformity worse but cannot correct it. In fixed deformities, the pain subsides, but the lack of motion persists; neurologic deficits are rare.142
The mechanism of injury is often trivial trauma, whereby the neck is rotated and tilted, resulting in subluxation of C1 on C2.143,144 The infectious etiology is known as Grisel syndrome and most commonly occurs after an upper respiratory tract infection but can also occur after tonsillectomy, pharyngoplasty, or retropharyngeal abscess.145 Because of the anastomoses between the veins and lymphatics draining the pharynx and periodontoid plexus, inflammation in the pharynx can lead to attenuation of the transverse ligament or synovium or both surrounding C1-2, resulting in subluxation.
X-ray examination can be challenging because of the difficulty in positioning a patient with torticollis, but anteroposterior and open-mouth odontoid images should be obtained in as close to a neutral position as possible.146 The lateral masses appear different in size because one is rotated anteriorly and one posteriorly, and the distances from the lateral masses to the dens are asymmetrical. Two-dimensional and three-dimensional CT scans have largely replaced cineradiography and can show superimposition of the atlas on the axis in a rotated position.147,148
Treatment options depend on the timing of injury and duration of symptoms,149 with some children likely never receiving medical attention because of spontaneous reduction. In patients whose symptoms have persisted less than 1 week, they can be treated with a soft collar, anti-inflammatories, and home exercises. If spontaneous reduction does not occur after a week, the patients should be put in head halter traction and bed rest, which usually relieves the symptoms if they have persisted less than 1 month. In cases of atlantoaxial rotatory subluxation that have endured more than 1 month, reduction with head halter traction is unlikely,150 but halo traction can be used to attempt reduction. More weight can be used with the halo, while applying a rotation to the right and left to assist the reduction. Documentation of the reduction should be performed by CT scan, and then the patient must remain in the halo vest for 6 weeks. If reduction is unable to be maintained, posterior fusion of C1-2 is recommended. Additionally, fusion is recommended if the halo immobilization fails after 6 weeks, if the subluxation has been present for more than 3 months, or if the patient has instability or neurologic compromise.151
Odontoid Fractures
Odontoid fractures are one of the most common cervical spine fractures in children, reported to represent up to 75% of all cervical spine fractures owing to the large head-to-body size ratio.152 In children, the most common mechanism is falling, whereas in young adults the mechanism is typically high-velocity trauma such as motor vehicle accidents.
Fractures of the odontoid in children typically occur through the synchondrosis, which is a cartilage line at the base of the odontoid. Fractures that reduce spontaneously can look like a Salter I injury or appear to be normal on plain films. CT scans with three-dimensional reconstructions can assist in identifying minimally displaced odontoid fractures,153 and MRI can show bone and soft tissue edema around a minimally displaced fracture.
Most odontoid fractures are displaced anteriorly with an intact anterior periosteal sleeve that can help stabilize the fracture when immobilized in extension and help encourage healing.148,154–156 Treatment of displaced fractures is by closed reduction via an extension or hyperextension maneuver with immobilization in a halo cast or Minerva jacket for children younger than 3 years. Most fractures heal uneventfully after 6 to 8 weeks, at which time documentation of stability with flexion-extension views is recommended. In cases that are difficult to reduce, head halter or halo traction, and rarely manipulation under general anesthesia, is necessary. The need for surgical fixation is unusual because of the excellent results from closed treatment.157–159
Os Odontoideum
Os odontoideum is an unsupported round ossicle that is separated from the body of the axis by a transverse gap. Some authors propose that os odontoideum is due to an unrecognized fracture that is distracted by the alar ligaments and results in nonunion,33,142,160–168 whereas other authors suggest that it is congenital because of its association with congenital syndromes.169–171 Presentation ranges from asymptomatic to frank myelopathy. Diagnosis can usually be made on routine x-rays, with lateral flexion-extension views showing the degree of displacement of C1 on C2. Measuring the distance between C1 and the ossicle is not helpful, however, because they move together. Instead, one should evaluate the space available for the cord or the relationship of the body of the axis to the posterior aspect of the anterior arch of C1. More recently, investigators have suggested using dynamic (real-time) MRI to aid in diagnosis of instability.172
There is debate in the literature regarding management of patients with os odontoideum. Some authors advocate conservative management with yearly clinical and radiographic evaluation of patients with a stable os odontoideum.161,173–175 There are reports, however, of decompensation and death in asymptomatic patients who have been followed conservatively.161,176,177 Many surgeons favor surgical stabilization of patients with an os odontoideum.175,177–180 Options include methods for C1-2 arthrodesis as already discussed.180,181
Hangman’s Fracture
A hangman’s fracture, or traumatic spondylolisthesis, is a fracture of the bilateral pars interarticularis of C2. This name derives from the fact that the fracture resembles the injury associated with a judicial hanging. Because the fracture fragments separate and decompress the cord, neurologic injury is unusual. Most of these injuries occur in children younger than 2 years, likely because of the large head size and poor muscle control. X-rays often show anterior subluxation of C2 on C3 with lucencies anterior to the pedicles of the axis. Persistent synchondroses of the axis have been reported that can be confused with hangman’s fractures.133,182–184 Treatment consists of immobilization for 8 to 12 weeks in a Minerva jacket, halo, or cervical orthosis. Pizzutillo and colleagues185 reported healing in four of five children treated in a Minerva jacket or halo cast. If nonunion occurs, anterior or posterior arthrodesis can stabilize the fracture.151
Lower Cervical (Subaxial) Spine Injuries
Traumatic injuries to the lower cervical spine (C3-7) are rare in young children and infants but are more common in adolescents and older children. Children younger than age 9 account for only 22% to 31% of pediatric lower cervical spine injuries, whereas children older than age 10 account for 70% to 73%.186 One should suspect a cervical spine injury if the patient is unconscious, has cervical rigidity, has muscle guarding, has neck pain, has radicular pain, has numbness, or has neurologic deficits.186 In infants, motor weakness and hypotonia should raise suspicion of a cervical spine injury.
Compression Fractures
Compression fractures are the most common fracture in the pediatric lower cervical spine. Trauma causing flexion and axial loading leads to a loss of vertebral height. When evaluating a child, one must be careful not to mistake normal cervical wedging for a compression fracture. Ossification is incomplete until age 7 years, and normal anterior wedging of the lower cervical spine until this age is considered normal. This wedging is usually most notable at the C3 vertebra.187 Compression fractures are usually considered stable injuries and can be treated nonoperatively. With immobilization, these fractures normally heal in 3 to 6 weeks. Flexion and extension films should be obtained at 2 to 4 weeks to confirm stability and alignment of the fracture.
Facet Fractures and Dislocations
Facet fractures and dislocations are the second most common lower cervical spine injuries in children and most commonly occur in adolescents.151 Unilateral dislocations often affect the nerve root, whereas bilateral dislocations affect the spinal cord.188 The cartilaginous components are overlapped and locked causing a “perched facet” on x-ray, which indicates a true dislocation. Treatment for a unilateral dislocation is initially traction and reduction if the patient is awake and able to cooperate with an examination. If the dislocation cannot be reduced, open reduction and arthrodesis is warranted. Bilateral facet fractures and dislocations are considered unstable, and reduction and stabilization is required.151 Figure 28–3 shows a 6-year-old girl with bilateral lumbar facet dislocations.
Burst Fractures
Burst fractures in the subaxial spine are rare in pediatric patients. These injuries occur secondary to axial loading. Retropulsed fragments and occult laminar fractures can compromise the spinal canal. CT is a useful modality in determining canal compromise and bony involvement. A burst fracture with no neurologic deficit and minimal spinal canal compromise can be treated with traction followed by halo immobilization. If imaging shows significant canal compromise or the patient has neurologic deficits, surgical decompression and fixation is recommended typically via anterior arthrodesis. This is one of the few instances where anterior fusion is recommended in children because the need for stability outweighs the risk of developing a deformity secondary to destruction of the anterior growth plate. In older children and adolescents, anterior instrumentation can be used without as much risk of kyphotic deformity.151
Vertebral Growth Plate Fractures
In contrast to adults, lower cervical spine injuries in children can occur through the synchondrosis at the cartilaginous endplate. The primary ossification centers fuse by 7 to 8 years of age, but the secondary ossification centers can remain open until 25 years of age.189 MRI can be used to assess the growth plate because it may be difficult to differentiate normal lucency of a synchondrosis from a traumatic lesion on x-ray. Fracture of the synchondrosis can lead to anterior or posterior displacement of the endplate. Anterior displacement can be treated conservatively with a cervical orthosis until the fracture has healed. Posterior displacement of the endplate is treated with an anterior surgical approach to reduce the bony nucleus resulting in spinal decompression and alignment. The patient should remain in a cervical orthosis for 4 to 5 months.190
Pediatric Halo
Halo immobilization is often used in the treatment of pediatric cervical spine injuries, even in infants. Presized halos and vests are used in adults, and these often work for adolescents. Prefabricated halos and vests are also available for children and infants, but because of the wide variability in size in children, custom vests and rings may be necessary. CT is recommended before pin placement to help determine bone structure. The pediatric skull is thickest anterolaterally and posterolaterally, and pins should be placed accordingly, with attention to the supraorbital and supratrochlear nerves.191 The number of pins required varies with age. Children younger than 2 years require 8 to 10 pins, but by age 5 years only 4 pins may be necessary.188 Pins should be inserted perpendicular to the skull to improve pin-bone interface.192 The amount of torque required during pin insertion decreases with age, with 2 to 4 inch-pounds required for younger children and standard torque of 6 to 8 inch-pounds in adolescents. There can be great variation in pressures with different torque wrenches, however, and they should be calibrated before halo placement on a child.193 Pins should not be retightened at 48 hours in children. In adolescents, retightening can be done at 48 hours, however.151
Complications are common with halo immobilizers in children. Dormans and colleagues194 reported a complication rate of 68% but noted that most complications were minor, and all patients were able to wear the halo until fracture healing occurred. The most common complications include superficial pin tract infection and pin loosening. Serious but less common complications include dural penetration, supraorbital and supratrochlear nerve injury, pin scars, and deep pin infections.
Thoracolumbar Classification Systems
Denis195 described a three-column classification system. The anterior column consists of the anterior ligament, the anterior anulus fibrosus, and the anterior part of the vertebral body. The middle column contains the posterior longitudinal ligament, the posterior anulus fibrosus, and the posterior wall of the vertebral body. The posterior column comprises the supraspinous and infraspinous ligaments, the posterior capsule, and the ligamentum flavum. Denis195 used this system to classify thoracolumbar injuries as compression fractures, burst fractures, flexion-distraction injuries, or fracture-dislocation injuries. He also classified the stability of these fractures based on the number of columns affected. If two or more columns fail, the fracture is considered unstable according to Denis.195
The Spine Trauma Study Group developed the TLICS scale. Three primary axes were identified to help analyze and manage fracture patterns: (1) injury morphology, (2) integrity of the posterior ligamentous complex, and (3) neurologic status. The three primary axes are divided further into subgroups. Morphology is determined from radiographs, MRI, and CT scan using one of three morphologic categories: (1) compression, which can be classified further with a burst component, (2) translation and rotation, and (3) distraction. The integrity of the posterior ligamentous complex is categorized as (1) intact, (2) disrupted, or (3) indeterminate. The neurologic status is categorized as intact, nerve root injury, complete spinal cord injury, or incomplete spinal cord injury. The TLICS score is based on these principles, and specific values are assigned to each subgroup with lesser point values for less severe injuries. These scores can help guide surgical treatment. Patients with a score of 3 or less are generally treated nonoperatively depending on the type of injury. Patients with a score of 5 or greater generally require surgical fixation. Patients with a score of 4 fall in the intermediate zone where treatment is up to the discretion of the surgeon.187
Compression Fractures
Compression fractures are the most common thoracolumbar fracture pattern.196 The severity of the fracture is based on the percentage of height lost, but regardless of severity, these fractures are rarely associated with neurologic deficit. It is important to determine if the injury is acute or chronic because these injuries can commonly occur as a result of falls. MRI or a bone scan can be used to determine acuity. Compression fractures are nearly always stable injuries, but one must be sure to examine the posterior soft tissues to rule out a flexion-distraction injury. Additionally, a CT scan should be obtained to rule out a burst fracture. Treatment options depend on whether the fracture is isolated or if contiguous fractures are present. Isolated compression fractures are best treated with an extension orthosis. These fractures usually heal in 4 to 6 weeks, but x-rays should be obtained to monitor alignment. Contiguous compression fractures can result in kyphosis. If kyphosis greater than 40 degrees is present, surgical treatment should be considered. The preferred surgical treatment is generally posterior instrumentation spanning one to two levels above and below the injured vertebra, with decompression if a neurologic deficit exists.196
Burst Fractures
Burst fractures involve, at a minimum, the anterior and middle columns.195 These injuries are most common in the lower thoracic and upper lumbar regions. Cord injury can occur with retropulsion of bony fragments at the thoracolumbar junction resulting in conus medullaris or cauda equina syndromes. There have been few studies of burst fractures in immature patients. Most investigators believe that conservative treatment is warranted if the posterior ligament column is intact and there are no neurologic deficits. Treatment should include an extension molded cast or a thoracic, lumbar, or sacral orthosis. The goal is to allow the patient to maintain an upright position allowing ambulation.
If there is injury to the posterior ligamentous complex, surgical treatment may be used to protect the integrity of the spine. Anterior and posterior approaches can be used.197 The posterior approach allows for decompression and direct fracture reduction. The anterior approach allows direct canal decompression through corpectomy of the fractured vertebrae.196 Figure 28–4 shows a 13-year-old boy with a lumbar burst fracture.
Chance Fractures
Chance fractures, or flexion-distraction injuries, are most often caused by lap belt injuries in motor vehicle accidents. With frontal impact, the lap belt causes the axis of rotation to be the anterior spine, causing distractive forces on the posterior spine and anterior vertebral compression. According to the Denis classification, this is a three-column injury and is unstable.195 The classic finding on x-ray is an “empty facet” sign. The inferior articular process of the superior vertebrae is no longer in contact with the superior articular process of the inferior vertebrae, and the facet appears empty.196 Treatment of Chance fractures is based on severity of the injury. If only bony fractures are present with no injury to the ligamentous complexes, a hyperextension cast can be used. If ligamentous disruption is present, surgical treatment is necessary. Young children can be treated surgically with posterior wiring combined with a cast, whereas older children and adolescents can undergo segmental fixation.196,198 Figure 28–5 shows a Chance fracture in a 5-year-old boy.
Fracture-Dislocations
Fracture-dislocations in the thoracolumbar spine are three-column injuries and are very unstable.195 These injuries nearly always require surgical stabilization. If complete spinal cord injury is present, internal fixation is warranted because it may aid the rehabilitation process. Children younger than 10 years should undergo longer fusions to reduce the occurrence of paralytic scoliosis later on.
Limbus Fractures
Limbus fractures occur at the posterior vertebral endplate when disc material herniates between the unfused peripheral ring apophysis of the epiphyseal endplates and central cartilage. These fractures often manifest as low back pain in adolescents and young adults, although they can be identified in older patients as well.199 These lesions are usually located in the lower lumbar spine and appear on x-rays as a corner defect in the endplate and a wedge-shaped piece of bone posterior to the body or disc space. CT and MRI can more fully delineate the bony defect and disc injury. These injuries are less likely to resorb on their own and often require surgical decompression in symptomatic patients.
Depending on the size of the lesion, the disc (calcified or noncalcified) and bony fracture can be removed via an extended laminotomy, hemilaminectomy, or laminectomy. In the absence of routine disc herniation, the discectomy can be performed in standard fashion, thus identifying the fracture as the endplate is exposed.199
Key Points
1 Herzenberg JE, Hensinger RN, Dedrick DK, et al. Emergency transport and positioning of young children who have an injury of the cervical spine: The standard backboard may be hazardous. J Bone Joint Surg Am. 1989;71:15-22.
2 Brown RL, Brunn MA, Garcia VF. Cervical spine injuries in children: A review of 103 patients treated consecutively at a level one pediatric trauma center. J Pediatr Surg. 2001;36:1107-1114.
3 Bilston LE, Brown J. Pediatric spinal injury type and severity are age and mechanism dependent. Spine. 2007;32:2339-2347.
4 Vaccaro AR, Lehman RAJr, Hurlbert RJ, et al. A new classification of thoracolumbar injuries: The importance of injury morphology, the integrity of the posterior ligamentous complex, and neurologic status. Spine. 2005;30:2325-2333.
5 Mayfield JK, Erkkila JC, Winter RB. Spine deformity subsequent to acquired childhood spinal cord injury. J Bone Joint Surg Am. 1981;63:1401-1411.
1 Cirak B, Ziegfeld S, Knight V, et al. Spinal injuries in children. J Pediatr Surg. 2004;39:607-612.
2 Kokoska ER, Keller M, Rallo MC, et al. Characteristics of pediatric cervical spine injuries. J Pediatr Surg. 2001;36:100-105.
3 d’Amato C. Pediatric spinal trauma. Clin Orthop Relat Res, 432. 2005:34-40.
4 Givens TG, Polley K, Smith GF, et al. Pediatric cervical spine injury: A three-year experience. J Trauma. 1996;41:2310-2314.
5 Bilston LE, Brown J. Pediatric spinal injury type and severity are age and mechanism dependent. Spine. 2007;32:2339-2347.
6 Vogel LC. Unique management needs of pediatric spinal cord injury in patients: Etiology and pathophysiology. J Spinal Cord Med. 1997;20:10-13.
7 Leventhal HR. Birth injuries of the spinal cord. J Pediatr. 1960;56:447-453.
8 Ranjith RK, Mullet J, Burke TE. Hangman’s fracture caused by suspected child abuse. J Pediatr Orthop. 2002;11:329-332.
9 Rooks VJ, Sisler C, Burton B. Cervical spine injury in child abuse: Report of two cases. Pediatr Radiol. 1998;28:193-195.
10 Bailey DK. The normal cervical spine in infants and children. Radiology. 1952;59:712-719.
11 Herman MJ, Pizzutillo P. Cervical spine disorders in children. Clin Orthop Relat Res. 1999;30:457-466.
12 Bohn D, Armstrong D, Becker L, et al. Cervical spine injuries in children. J Trauma. 1990;30:463-469.
13 Eleraky M, Theodore N, Adams M, et al. Pediatric cervical spine injuries: Report of 102 cases and review of literature. J Neurosurg Spine. 2000;92:12-17.
14 Finch G, Barnes M. Major cervical spine injuries in children and adolescents. J Pediatr Orthop. 1998;186:811-814.
15 Nuckley D, Hersted S, Eck M, et al. Developmental biomechanics of the cervical spine: Tension and compression. J Biomech. 2005;38:2266-2275.
16 Ouyang J, Zhu Q, Zhao W, et al. Biomechanical assessment of the pediatric cervical spine under bending and tensile loading. Spine. 2005;30:716-723.
17 Herzenberg JE, Hensinger R, Dedrick DK, et al. Emergency transport and position of young children who have an injury of the cervical spine: The standard backboard may be hazardous. J Bone Joint Surg Am. 1989;71:15-22.
18 Curran C, Dietrich A, Bowman MJ, et al. Pediatric cervical spine immobilization: Achieving neutral position. J Trauma. 1995;39:729-732.
19 Nypaver M, Treloar D. Neutral cervical spine positioning in children. Ann Emerg Med. 1994;23:208-211.
20 Laham JL, Cotcamp D, Gibbons PA, et al. Isolated head injuries versus multiple trauma in pediatric patients: Do the same indications for cervical spine evaluation apply? Pediatr Neurosurg. 1994;21:221-226.
21 Slotkin JR, Lu Y, Wood KB. Thoracolumbar spinal trauma in children. Neurosurg Clin N Am. 2007;18:621-630.
22 Hadley MN, Zabramski JM, Browner CM, et al. Pediatric spinal trauma: Review of 122 cases of spinal cord and vertebral column injuries. J Neurosurg. 1988;68:18-24.
23 Lee SL, Sena M, Greenholz SK, et al. A multidisciplinary approach to the development of a cervical spine clearance protocol: Process, rationale, and initial results. J Pediatr Surg. 2003;38:358-362.
24 Bonadio WA. Cervical spine trauma in children: General concepts, normal anatomy, radiographic evaluation. Am J Emerg Med. 1993;11:158-165.
25 Lally KP, Senac M, Hardin WD, et al. Utility of the cervical spine radiograph in pediatric trauma. Am J Surg. 1989;158:540-542.
26 Baker C, Kadish H, Schunk JE. Evaluation of pediatric cervical spine injury. Am J Emerg Med. 1999;17:230-234.
27 Buhs C, Cullen M, Klein M, et al. The pediatric trauma C-spine: Is the “odontoid” view necessary? J Pediatr Surg. 2000;35:994-997.
28 Ralston ME, Chung K, Barnes PD, et al. Role of flexion-extension radiographs in blunt pediatric cervical spine injury. Acad Emerg Med. 2001;8:237-245.
29 Dwek JR, Chung C. Radiography of cervical spine injury in children: Are flexion-extension radiographs useful for acute trauma? AJR Am J Roentgenol. 2000;174:1617-1619.
30 Bucholz RW, Burkhead WZ. The pathological anatomy of fatal atlanto-occipital dislocations. J Bone Joint Surg Am. 1979;61:148-250.
31 Kaufman RA, Carroll CD, Buncher CR. Atlantooccipital junction: Standards for measurement in normal children. AJNR Am J Neuroradiol. 1987;8:995-999.
32 Roche C, Carty H. Spinal trauma in children. Pediatr Radiol. 2001;31:677-700.
33 Fielding JW, Cochran JB, Lawsing JF, et al. Tears of the transverse ligament of the atlas: A clinical and biomechanical study. J Bone Joint Surg Am. 1974;56:1683-1691.
34 Steel HH. Anatomical and mechanical considerations of the atlanto-axial articulation. J Bone Joint Surg Am. 1968;50:1481-1482.
35 Swischuk LE. Anterior displacement of C2 in children. Radiology. 1977;122:759-763.
36 Cattell HS, Filtzer D. Pseudosubluxation and other normal variations in the cervical spine in children. J Bone Joint Surg Am. 1965;47:1295-1309.
37 Sheridan R, Peralta R, Rhea J, et al. Reformatted visceral protocol helical computed tomographic scanning allows conventional radiographs of the thoracic and lumbar spine to be eliminated in the evaluation of blunt trauma patients. J Trauma. 2003;55:665-669.
38 Stambolis V, Brady S, Klos D, et al. The effects of cervical bracing upon swallowing in young, normal, healthy volunteers. Dysphagia. 2003;18:39-45.
39 Naidich JB, Naidich TP, Garfein C, et al. The widened interspinous distance: A useful sign of anterior cervical dislocation in the supine frontal projection. Radiology. 1977;123:113-116.
40 Bonadio W. Cervical spine trauma in children, II: Mechanisms and manifestations of injury, therapeutic considerations. Am J Emerg Med. 1993;11:256-278.
41 Ardran GM, Kemp F. The mechanism of changes of the cervical airway in infancy. Med Radiogr Photogr. 1968;44:26-38.
42 Davis JW, Phreaner DL, Hoyt DB, et al. The etiology of missed cervical spine injuries. J Trauma. 1993;34:342-346.
43 Gerrelts BD, Petersen EU, Mabry J, et al. Delayed diagnosis of cervical spine injuries. J Trauma. 1991;31:1622-1626.
44 Grogan EL, Morris JAJ, Dittus RS, et al. Cervical spine evaluation in urban trauma centers: Lowering institutional costs and complications through helical CT scan. J Am Coll Surg. 2005;200:160-165.
45 Nunez DBJ, Zuluaga A, Fuentes-Bernardo DA, et al. Cervical spine trauma: How much more do we learn by routinely using helical CT? RadioGraphics. 1996;16:1307-1318.
46 Fearon T, Vucih J. Normalized pediatric organ-absorbed doses from CT examinations. AJR Am J Roentgenol. 1987;148:171-174.
47 Huda W, Bissessur K. Effective dose equivalents, HE, in diagnostic radiology. Med Physics. 1990;17:998-1003.
48 Gestring ML, Gracias VH, Feliciano MA, et al. Evaluation of the lower spine after blunt trauma using abdominal computed tomographic scanning supplemented with lateral scanograms. J Trauma. 2002;53:9-14.
49 Hauser CJ, Visvikis G, Hinrichs C, et al. Prospective validation of computed tomographic screening of the thoracolumbar spine in trauma. J Trauma. 2003;55:228-234.
50 Wintermark M, Mouhsine E, Theumann N, et al. Thoracolumbar spine fractures in patients who have sustained severe trauma: Depiction with multi-detector row CT. Radiology. 2003;277:681-689.
51 Brown RL, Brunn MA, Garcia VF. Cervical spine injuries in children: A review of 103 patients treated consecutively at a level 1 pediatric trauma center. J Pediatr Surg. 2001;36:1107-1114.
52 Flynn JM, Closkey RF, Mahboubi S, et al. Role of magnetic resonance imaging in the assessment of pediatric cervical spine injuries. J Pediatr Orthop. 2002;22:573-577.
53 Keiper MD, Zimmerman RA, Bilaniuk LT. MRI in the assessment of the supportive soft tissues of the cervical spine in acute trauma in children. Neuroradiology. 1998;40:359-363.
54 Frank JB, Lim CK, Flynn JM, et al. The efficacy of magnetic resonance imaging in pediatric cervical spine clearance. Spine. 2002;27:1176-1179.
55 Davis JW, Parks SN, Detlefs CL, et al. Clearing the cervical spine in obtunded patients: The use of dynamic fluoroscopy. J Trauma. 1995;39:435-438.
56 Kolb JC, Summers RL, Galli RL. Cervical collar-induced changes in intracranial pressure. Am J Emerg Med. 1999;17:135-137.
57 Viccellio P, Simon H, Pressman BD, et al. A prospective multicenter study of cervical spine injury in children. Pediatrics. 2001;108:E20.
58 Stiell IG, Clement CM, McKnight RD, et al. The Canadian C-spine rule versus the NEXUS low-risk criteria in patients with trauma. N Engl J Med. 2003;349:2510-2518.
59 Anderson RC, Kan P, Hansen KW, et al. Cervical spine clearance after trauma in children. Neurosurg Focus. 2006;20:E3.
60 Launay F, Leet AI, Sponseller PD. Pediatric spinal cord injury without radiographic abnormality: A meta-analysis. Clin Orthop Relat Res. 2005;433:166-170.
61 Pang D. Spinal cord injury without radiographic abnormality in children, 2 decades later. Neurosurgery. 2004;55:1325-1342.
62 Levi AD, Hurlbert J, Anderson P, et al. Neurologic deterioration secondary to unrecognized spinal instability following trauma: A multicenter study. Spine. 2006;31:451-458.
63 Bolinger B, Shartz M, Marion D. Bedside fluoroscopic flexion and extension cervical spine radiographs for clearance of the cervical spine in comatose trauma patients. J Trauma. 2005;56:132-136.
64 Ajani AE, Cooper DJ, Scheinkestel CD, et al. Optimal assessment of cervical spine trauma in critically ill patients: A prospective evaluation. Anaesth Intensive Care. 1998;26:487-491.
65 Stassen NA, Williams VA, Grestring ML, et al. Magnetic resonance imaging in combination with helical computed tomography provides safe and efficient method of cervical spine clearance in the obtunded patient. J Trauma. 2006;60:171-177.
66 Diaz JJ, Aulino JM, Collier B, et al. The early work-up for isolated ligamentous injury of the cervical spine: Does computed tomography scan have a role? J Trauma. 2005;59:897-904.
67 Marino RJ, Ditunno JFJ, Donovan WH, et al. Neurologic recovery after traumatic spinal cord injury: Data from the Model Spinal Cord Injury Systems. Arch Physical Med Rehabil. 1999;80:1391-1396.
68 Amar AP, Levy ML. Pathogenesis and pharmacological strategies for mitigating secondary damage in acute spinal cord injury. Neurosurgery. 1999;44:1027-1039.
69 Zivin JA, DeGirolami U. Spinal cord infarction: A highly reproducible stroke model. Stroke. 1980;11:200-202.
70 Zach GA, Seiler W, Dollfus P. Treatment results of spinal cord injuries in the Swiss Paraplegic Centre of Basel. Paraplegia. 1976;14:58-65.
71 Vale FL, Burns J, Jackson AB, et al. Combined medical and surgical treatment after acute spinal cord injury: Results of a prospective pilot study to assess the merits of aggressive medical resuscitation and blood pressure management. J Neurosurg. 1997;87:239-246.
72 Levi L, Wolf A, Belzerg H. Hemodynamic parameters in patients with acute cervical cord trauma: Description, intervention, and prediction of outcome. Neurosurgery. 1993;33:1007-1017.
73 Bracken MB, Shepard MJ, Holford TR, et al. Administration of methylprednisolone for 24 or 48 hours or tirilazad mesylate for 48 hours in the treatment of acute spinal cord injury. JAMA. 1997;277:1597-1604.
74 Bracken MB, Shepard MJ, Holford TR, et al. Methylprednisolone or tirilazad mesylate administration after acute spinal cord injury: 1-year follow-up. J Neurosurg. 1998;89:699-706.
75 Heary RF, Vaccaro AR, Mesa JJ, et al. Steroids and gunshot wounds to the spine. Neurosurgery. 1997;41:576-583.
76 Fehlings MG, Perrin RG. The timing of surgical intervention in the treatment of spinal cord injury: A systematic review of recent clinical evidence. Spine. 2006;31:S28-S35.
77 Pang D, Pollack IF. Spinal cord injury without radiographic abnormality in children—the SCIWORA syndrome. J Trauma. 1989;29:654-664.
78 Wang MY, Hoh DJ, Leary SP, et al. High rates of neurological improvement following severe traumatic pediatric spinal cord injury. Spine. 2004;29:1493-1497.
79 Anderson CJ, Vogel LC, Willis KM, et al. Stability of transition to adulthood among individuals with pediatric-onset spinal cord injuries. J Spinal Cord Med. 2006;29:46-56.
80 Jacobs RR, Asher MA, Snider RK. Thoracolumbar spinal injuries: A comparative study of recumbent and operative treatment in 100 patients. Spine. 1980;5:463-477.
81 Pouliquen JC, Kassis B, Glorion C, et al. Vertebral growth after thoracic or lumbar fracture of the spine in children. J Pediatr Orthop. 1997;17:115-120.
82 Vaccaro AR, Silber JS. Post-traumatic spinal deformity. Spine. 2001;26:S111-S118.
83 Yasuoka S, Peterson HA, MacCarty CS. Incidence of spinal column deformity after multilevel laminectomy in children and adults. J Neurosurg. 1982;57:441-445.
84 Mayfield JK, Erkkila JC, Winter RB. Spine deformity subsequent to acquired childhood spinal cord injury. J Bone Joint Surg Am. 1981;63:1401-1411.
85 Moe JH, Kharrat K, Winter RB, et al. Harrington instrumentation without fusion plus external orthotic support for the treatment of difficult curvature problems in young children. Clin Orthop Relat Res. 1984;185:35-45.
86 Dubousset J, Herring JA, Shufflebarger H. The crankshaft phenomenon. J Pediatr Orthop. 1989;9:541-550.
87 Pang D, Wilberger JEJ. Spinal cord injury without radiographic abnormalities in children. J Neurosurg. 1982;57:114-129.
88 Bosch MD, Vogt MT, Ward WT. Pediatric spinal cord injury without radiographic abnormality. Spine. 2002;27:2788-2800.
89 Grabb PA, Pang D. Magnetic resonance imaging in the evaluation of spinal cord injury without radiographic abnormality in children. Neurosurgery. 1994;35:406-414.
90 Flanders AE, Schaefer DM, Doan HT, et al. Acute cervical spine trauma: Correlation of MR imaging findings with degree of neurologic deficit. Radiology. 1990;177:25-33.
91 Davis D, Bohlman H, Walker AE, et al. The pathological findings in fatal craniospinal injuries. J Neurosurg. 1971;34:603-613.
92 Montane I, Eismont FJ, Green BA. Traumatic occipitoatlantal dislocation. Spine. 1991;15:112-116.
93 Farley FA, Graziano GP, Hensinger RN. Traumatic atlanto-occipital dislocation in a child. Spine. 1992;17:1539-1541.
94 Matava MJ, Whitesides TE, Davis PC. Traumatic atlanto-occipital dislocation with survival. Spine. 1993;18:1897-1903.
95 Papadopoulos SM, Dickman CA, Sonntag VKH, et al. Traumatic atlanto-occipital dislocation with survival. Neurosurgery. 1991;28:574-579.
96 Rockswold GL, Sljeskog EL. Traumatic atlantooccipital dislocation with survival. Minn Med. 1979;62:151-154.
97 Woodring JH, Selke ACJ, Duff DE. Traumatic atlantooccipital dislocation with survival. AJR Am J Roentgenol. 1981;137:21-24.
98 Wholey MH, Bruwer AJ, Baker HLJ. The lateral roentgenogram of the neck (with comments on the atlanto-odontoid-basion relationship). Radiology. 1958;71:350-356.
99 Powers B, Miller MD, Kramer RS, et al. Traumatic anterior atlanto-occipital dislocations. Neurosurgery. 1979;4:12-17.
100 Gerlock AJJ, Mirfahkraee M, Benzel EC. Computed tomography of traumatic atlanto-occipital dislocation. Neurosurgery. 1983;12:316-319.
101 Judd DB, Liem LK, Petermann G. Pediatric atlas fracture: A case of fracture through a synchondrosis and review of the literature. Neurosurgery. 2000;46:991-995.
102 Jefferson G. Fracture of the atlas vertebra: Report of four cases and a review of those previously recorded. Br J Surg. 1919-1920;7:407-422.
103 Marlin AE, Gayle RW, Lee JF. Jefferson fractures in children. J Neurosurg. 1983;58:277-279.
104 Mikawa Y, Watanabe R, Yamano Y, et al. Fractures through a synchondrosis of the anterior arch of the atlas. J Bone Joint Surg Br. 1987;69:483.
105 Richards PG. Stable fractures of the atlas and axis in children. J Neurol Neurosurg Psychiatry. 1984;47:781-783.
106 Hensinger RN, DeVito PD, Tagsdale CG. Stable fractures of the atlas and axis in children. J Bone Joint Surg Am. 1986;68:189-198.
107 Burke SW, French HG, Roberts JM, et al. Chronic atlantoaxial instability in Down syndrome. J Bone Joint Surg Am. 1985;67:1356-1360.
108 Dawson EG, Smith L. Atlanto-axial subluxation in children due to vertebral anomalies. J Bone Joint Surg Am. 1979;61:582-587.
109 Hammerschlag W, Ziv I, Wald U, et al. Cervical instability in an achondroplastic infant. J Pediatr Orthop. 1988;8:481-484.
110 Kobori M, Takahashi H, Mikawa Y. Atlanto-axial dislocation in Down’s syndrome: A report of two cases requiring surgical correction. Spine. 1986;11:195-200.
111 Kransdorf MJ, Wherle PA, Moser RPJ. Atlantoaxial subluxation in Reiter’s syndrome. Spine. 1988;13:13-14.
112 Miz GS, Engler GL. Atlanto-axial subluxation in Larsen’s syndrome: A case report. Spine. 1987;12:411-412.
113 McGrory BJ, Klassen RA, Chao EY, et al. Acute fracture and dislocations of the cervical spine in children and adolescents. J Bone Joint Surg Am. 1993;75:988-995.
114 Lui TN, Lee ST, Wong CW, et al. C1-C2 fracture-dislocations in children and adolescents. J Trauma. 1996;40:408-411.
115 American Academy of Pediatrics. Atlanto-axial instability in Down syndrome: Subject review. Pediatrics. 1995;96:151-154.
116 Pueschel SM, Scolia FH. Atlantoaxial instability in individuals with Down syndrome: Epidemiologic, radiographic, and clinical studies. Pediatrics. 1987;4:555-560.
117 Segal LS, Drummond DS, Zanotti RM, et al. Complications of posterior arthrodesis of the cervical spine in patients who have Down syndrome. J Bone Joint Surg Am. 1991;73:1547-1560.
118 Windell J, Burke SW. Sports participation of children with Down syndrome. Orthop Clin North Am. 2003;34:439-443.
119 Pueschel SM, Scolia FH, Tupper TB, et al. Skeletal anomalies of the upper cervical spine in children with Down syndrome. J Pediatr Orthop. 1990;10:607-611.
120 Cohen WI. Atlantoaxial instability: What’s next? Arch Pediatr Adolesc Med. 1998;152:119-122.
121 Merrick I, Ezra E, Josef B, et al. Musculoskeletal problems in Down syndrome. European Paediatric Orthopaedic Society Survey: The Israeli sample. J Pediatr Orthop B. 2000;9:610-619.
122 Caird MS, Wills BPD, Dormans JP. Down syndrome in children: The role of the orthopaedic surgeon. J Am Acad Orthop Surg. 2006;14:610-619.
123 Ferguson RI, Putney ME, Allen BLJ. Comparison of neurologic deficits with atlanto-dens intervals in patients with Down syndrome. J Spinal Disord. 1997;10:246-252.
124 Sherk HH, Whitaker LA, Pasquariello PS. Fascial malformations and spinal anomalies: A predictable relationship. Spine. 1982;7:526-531.
125 Gallie W. Fractures and dislocation of the cervical spine. Am J Surg. 1939;46:495-499.
126 Brooks AL, Jenkins EB. Atlanto-axial arthrodesis by the wedge compression method. J Bone Joint Surg Am. 1978;60:279-284.
127 Coyne TJ, Fehlings MG, Wallace MC, et al. C1-C2 posterior cervical fusion: Long-term evaluation of results and efficacy. Neurosurgery. 1995;37:688-692.
128 Smith MD, Phillips WA, Hensinger RN. Complications of fusion to the upper cervical spine. Spine. 1991;16:702-705.
129 Crisco JJI, Panjabi MM, Oda T, et al. Bone graft translation of four upper cervical spine fixation techniques in a cadaveric model. J Orthop Res. 1991;9:835-836.
130 Grob D, Crisco JJD, Panjabi MM, et al. Biomechanical evaluation of four different posterior atlantoaxial fixation techniques. Spine. 1992;17:480-490.
131 Hajek PD, Lipka J, Hartline P, et al. Biomechanical study of C1-C2 posterior arthrodesis techniques. Spine. 1993;18:173-177.
132 Naderi S, Crawford NR, Song GS, et al. Biomechanical comparison of C1-C2 posterior fixations: Cable, graft, and screw combinations. Spine. 1998;23:1946-1955.
133 Smith T, Skinner SR, Shonnard NH. Persistent synchondrosis of the second cervical vertebra simulating a hangman’s fracture in a child. J Bone Joint Surg Am. 1993;75:1228-1230.
134 Dickman CA, Sonntag VK. Surgical management of atlantoaxial nonunions. J Neurosurg. 1995;83:248-253.
135 Grob D, Magerl F. Surgical stabilization of C1 and C2 fractures. Orthopade. 1987;16:46-54.
136 Grob D, Jeanneret B, Aebi M, et al. Atlanto-axial fusion with transarticular screw fixation. J Bone Joint Surg Br. 1991;73:972-976.
137 Jeanneret B, Magerl F. Primary posterior fusion C1/C2 in odontoid fractures: Indications, technique, and results of transparticular screw fixation. J Spinal Disord. 1992;5:464-475.
138 Stillerman CB, Wilson JA. Atlanto-axial stabilization with posterior transarticular screw fixation: Technical description and report of 22 cases. Neurosurgery. 1993;32:948-954.
139 Madawi AA, Casey AT, Solanki GA, et al. Radiological and anatomical evaluation of the atlantoaxial transarticular screw fixation technique. J Neurosurg. 1997;86:961-968.
140 Goel A, Laheria V. Plate and screw fixation for atlanto-axial subluxation. Acta Neurochir. 1994;129:47-53.
141 Harms J, Melcher RP. Posterior C1-C2 fusion with polyaxial screw and rod fixation. Spine. 2001;26:2467-2471.
142 Fielding JW, Hawkins RJ. Atlanto-axial rotary fixation (fixed rotary subluxation of the atlanto-axial joint). J Bone Joint Surg Am. 1977;59:37-44.
143 Coutts MB. Atlanto-epistropheal subluxations. Arch Surg. 1934;29:297-311.
144 Crook TB, Enyon CA. Traumatic atlantoaxial rotatory subluxation. Emerg Med J. 2005;22:671-672.
145 Wetzel FT, Larocca H. Grisel’s syndrome: A review. Clin Orthop Relat Res. 1989;240:141-152.
146 Maheshwaran S, Sgouros S, Jeyapalan K, et al. Imaging of childhood torticollis due to atlanto-axial rotatory fixation. Childs Nerv Syst. 1995;11:667-671.
147 Geehr RB, Rothman SLG, Kier EL. The role of computed tomography in the evaluation of upper cervical spine pathology. Comput Tomogr. 1978;2:79-97.
148 Scapinelli R. Three dimensional computed tomography in infantile atlantoaxial rotatory fixation. J Bone Joint Surg Br. 1994;76:367-370.
149 Phillips WA, Hensinger RN. The management of the rotatory atlanto-axial subluxation in children. J Bone Joint Surg Am. 1989;71:664-668.
150 Burkus JK, Deponte RJ. Chronic atlantoaxial rotatory fixation: Correction by cervical traction, manipulation, and branching. J Pediatr Orthop. 1986;6:631-635.
151 Warner WC, Hedequist DJ. Cervical spine injuries in children. In: Beaty JH, Kasser JR, editors. Rockwood and Wilkins’ Fractures in Children. 6th ed. Philadelphia: Lippincott Williams & Wilkins; 2006:776-816.
152 Sherk HH. Fractures of the atlas and odontoid process. Orthop Clin North Am. 1978;9:973-984.
153 Sherburn EW, Day RA, Kaufman BA, et al. Subdental synchondrosis fracture in children: The value of a 3-dimensional computerized tomography. Pediatr Neurosurg. 1996;25:256-259.
154 Apple JS, Kirks DR, Merten DF, et al. Cervical spine fractures and dislocations in children. Pediatr Radiol. 1987;17:45-49.
155 Ries MD, Ray S. Posterior displacement of an odontoid fracture in a child. Spine. 1986;11:1043-1044.
156 Shaw BA, Murphy KM. Displaced odontoid fracture in a 9-month-old child. Am J Emerg Med. 1999;1:73-75.
157 Godard J, Hadji M, Raul JS. Odontoid fractures in the child with neurologic injury: Direct osteosynthesis with a cortico-spongious screw and literature review. Childs Nerv Syst. 1997;13:105-107.
158 Price E. Fractured odontoid process with anterior dislocation. J Bone Joint Surg Br. 1960;42:410-413.
159 Schippers N, Konigs D, Hassler W, et al. Typical and atypical fractures of the odontoid process in young children: Report of two cases and a review of the literature. Acta Neurochir. 1996;138:524-530.
160 Fielding JW. Cineroentgenography of the normal cervical spine. J Bone Joint Surg Am. 1957;39:187-190.
161 Fielding JW, Hensinger RN, Hawkins RJ. Os odontoideum. J Bone Joint Surg Am. 1980;62:376-383.
162 Fielding JW, Stillwell WT, Chynn KY, et al. Use of computed tomography for the diagnosis of atlanto-axial rotatory fixation. J Bone Joint Surg Am. 1978;60:1102-1104.
163 Hawkins RJ, Fielding JW, Thompson WJ. Os odontoideum: Congenital or acquired. J Bone Joint Surg Am. 1976;58:413-414.
164 Hukda S, Ora H, Okabe N, et al. Traumatic atlantoaxial dislocation causing os odontoideum in infants. Spine. 1980;5:207-210.
165 Kuhns LR, Loder RT, Farley FA, et al. Nuchal cord changes in children with os odontoideum: Evidence for associated trauma. J Pediatr Orthop. 1998;18:815-819.
166 Ricciardi JE, Kaufer H, Louis DS. Acquired os odontoideum following acute ligament injury. J Bone Joint Surg Am. 1976;58:410-412.
167 Stillwell WT, Fielding JW. Acquired os odontoideum. Clin Orthop Relat Res. 1978;135:71-73.
168 Verska JM, Anderson PA. Os odontoideum: A case report of one identical twin. Spine. 1997;22:706-709.
169 Giannestras NJ, Mayfield FH, Maurer J. Congenital absence of the odontoid process. J Bone Joint Surg Am. 1964;46:839-843.
170 Sherk HH, Dawoud S. Congenital os odontoideum with Klippel-Feil anomaly and fatal atlanto-axial instability. Spine. 1981;6:42-45.
171 Wollin DG. The os odontoideum. J Bone Joint Surg Am. 1971;45:1459-1471.
172 Hughes TBJ, Richman JB, Rothfus WE. Diagnosis of os odontoideum using kinematic magnetic resonance imaging: A case report. Spine. 1999;24:715-718.
173 Clements WD, Mezue W, Matthew B. Os odontoideum: Congential or acquired? That’s not the question. Injury. 1995;26:640-642.
174 Spierings EL, Braakman R. The management of os odontoideum: Analysis of 137 cases. J Bone Joint Surg Br. 1982;64:422-428.
175 Dai L, Yuan W, Ni B, et al. Os odontoideum: Etiology, diagnosis, and management. Surg Neurol. 2000;53:106-108.
176 Michaels L, Prevost MJ, Crang DF. Pathologic changes in a case of os odontoideum (separate odontoid process). J Bone Joint Surg Am. 1996;51:956-972.
177 Klimo PJ, Kan P, Rao G, et al. Os odontoideum: Presentation, diagnosis, and treatment in a series of 78 patients. J Neurosurg Spine. 2008;9:332-342.
178 Lowry DW, Pollack IF, Clyde B, et al. Upper cervical spine fusion in the pediatric population. J Neurosurg. 1997;87:671-676.
179 Taggard DA, Menezes AH, Ryken TC. Treatment of Down syndrome-associated craniovertebral junction abnormalities. J Neurosurg. 2000;93:205-213.
180 Wang J, Vokshoor A, Kim S, et al. Pediatric atlantoaxial instability: Management with screw fixation. Pediatr Neurosurg. 1999;30:70-78.
181 Brockmeyer DL, York JE, Apfelbaum RI. Anatomic suitability of C1-C2 transarticular screw placement in pediatric patients. J Neurosurg. 2000;92:7-11.
182 Matthews LS, Vetter LW, Tolo VT. Cervical anomaly stimulating hangman’s fracture in a child. J Bone Joint Surg Am. 1982;64:299-300.
183 Nordstrom REA, Lahdenrants TV, KaitilaII, et al. Familial spondylolisthesis of the axis vertebra. J Bone Joint Surg Br. 1986;68:704-706.
184 Williams JPI, Baker DH, Miller WA. CT appearance of congential defect resembling the hangman’s fracture. Pediatr Radiol. 1990;29:549-550.
185 Pizzutillo PD, Rocha EF, D’Astous J, et al. Bilateral fractures of the pedicle of the second cervical vertebra in the young child. J Bone Joint Surg Am. 1986;68:892-896.
186 Dogan S, Safavi-Abbasi S, Theodore N, et al. Pediatric subaxial cervical spine injuries: Origins, management, and outcome in 51 patients. Neurosurg Focus. 2006;20:E1.
187 Rihn JA, Anderson DT, Harris E, et al. A review of the TCLIS system: A novel, user-friendly thoracolumbar trauma classification system. Acta Orthop. 2008;79:461-466.
188 McCall T, Fasset D, Brockmeyer D. Cervical spine trauma in children: A review. Neurosurg Focus. 2006;20:E5.
189 Lawson JP, Ogden JA, Bucholz RW, et al. Physeal injuries of the cervical spine. J Pediatr Orthop. 1987;7:428-435.
190 Vaille R, Mary P, Schmider L, et al. Spinal fracture through the neurocentral synchondrosis in battered children: A case report. Spine. 2006;31:E345-E349.
191 Garfin SR, Roux R, Botte MJ, et al. Skull osteology as it affects halo pin placement in children. J Pediatr Orthop. 1986;6:434-435.
192 Copley LA, Pepe MD, Tan V, et al. A comparison of various angles of halo pin insertion in an immature skull model. Spine. 1999;24:1777-1780.
193 Copley LA, Dormans JP, Pepe MD, et al. Accuracy and reliability of torque wrenches used for halo application in children. J Bone Joint Surg Am. 2003;85:2199-2204.
194 Dormans JP, Criscitiello AA, Drummond DS, et al. Complications in children managed with immobilization in a halo vest. J Bone Joint Surg Am. 1995;77:1370-1373.
195 Denis F. The three column spine and its significance in the classification of acute thoracolumbar spinal injuries. Spine. 1983;6:817-831.
196 Newton PO. Thoracolumbar spine fractures. In: Beatty JH, Kasser JR, editors. Rockwood and Wilkins’ Fractures in Children. 6th ed. Philadelphia: Lippincott Williams & Wilkins; 2006:816-830.
197 Wood K, Butterman G, Mehbod A, et al. Operative compared with nonoperative treatment of a thoracolumbar burst fracture without neurological deficit. J Bone Joint Surg Br. 2003;85:773-781.
198 Rumball K, Jarvis J. Seat belt injuries of the spine in young children. J Bone Joint Surg Br. 1992;74:571-575.
199 Epstein NE. Lumbar surgery for 56 limbus fractures emphasizing noncalcified type III lesions. Spine. 1992;17:1489-1496.