CHAPTER 51 Cervical Instability
INTRODUCTION
The definition of spinal instability has been a subject of considerable debate for decades, even among experts. According to White and Panjabi, ‘the loss of the ability of the spine under physiologic loads to maintain relationships between vertebrae in such a way that there is neither initial nor subsequent damage to the spinal cord or nerve root, and in addition, there is neither development of incapacitating deformity or severe pain.’1 In this definition, physiologic loads refer to those incurred during normal activity of the patients being examined. They preferred to emphasize the relationship between the mechanical derangement and pain or neurologic deficit. They also preferred a checklist to standardize the evaluation of cervical instability and show a systematic approach.
Earlier, Allen et al.2 presented a mechanistic classification system of injuries to the lower cervical spine. In their opinion the skeletal injury, the neurologic injury, associated injuries, medical disorders, and unique individual factors should be analyzed in each case to determine acute injury and risk for late instability.
Instability has been defined in anatomical, biomechanical, and clinical terms, but the variability of clinical presentations and the inability of sequential laboratory lesions to replicate clinical experiences indicate that instability should be defined in anatomical terms. Larson has emphasized that the stable spinal column is symmetrical in movement and configuration, whether normal or abnormal, and does not change with time.3,4 In considering spinal instability, it is necessary to generally review the column theory of vertebral stability. The two-column or three-column concepts of Holdsworth, White, Denis, and Louis are frequently used to conceptualize the mechanical integrity of the spinal column.1,5–7 The definition of the extent of injury to the soft tissues and bony components of the spinal column will assist the clinician in determining the risk to neural structures from alterations in stability or alignment. Although inclusion of a middle column has a theoretical advantage in thoracic and lumbar injuries, these considerations are not as anatomically important in the cervical spine and it is reasonable to use the two-column model in the cervical region (Fig. 51.1). As previously noted, laboratory studies of cervical instability determined through a sequential pattern of component section of a specific column are not usually representative of clinical injury which may include only selective elements of each column.8 The degree and character of a column compromise, however, are important indicators alerting the clinician to the potential risk of instability. Additionally, this information, especially the potential role of ligamentous injury, will assist in recognizing the possible development of delayed instability (subacute or chronic) which may be difficult to establish in the early post-trauma period. The degree of instability, irrespective of temporal occurrence, will be an important influence upon the threat or occurrence of neurologic injury. Therefore, clarification of the degree of injury and a more precise measurement of displacement will assist in determining the threat to neurologic integrity.
UPPER CERVICAL SPINE
Anatomic and biomechanical considerations
The anatomic stability of occipito-atlanto-axial joint complex is gained mainly by the ligament, capsule, and structural membrane (Fig. 51.2). The stability of occiput–C1 joint is provided by their capsule, along with the anterior and posterior atlanto-occipital membrane, enhanced by the additional structures such as the alar ligament, apical ligament, and the tectorial membrane. The transverse, dentate, and apical ligaments play an important role in structural stability of the atlantoaxial joint. The injury or dysfunction of the transverse ligament leads to the translation of C1 on C2 and widening of the atlantodens interval (ADI), which can be detected by lateral X-ray of the cervical spine. Functional loss of the alar ligaments indicates a potential for rotatory instability, which, however, must be determined in conjunction with other clinical findings, such as neurological dysfunction, pain, and deformity.9
Knowledge of the normal movements of the occipito-atlanto-axial joint complex is important for evaluating clinical cases that may be potentially unstable. The occiput and axis are sometimes described as two rotating squares with the atlas as a bearing between them. The joints between the atlas and axis are almost horizontal and therefore allow for a wide range of motion in rotation, whereas rotation between the occiput and atlas is completely prevented by the geocentric anatomy of the articulation. The investigations of measurement of range of motion of C0–C1–C2 are shown in Tables 51.1 and 51.2. Both joints, C0–C1 and C1–2, participate in flexion and extension. Extension at occiput–C1 is one of the largest single motions in the spine. Lateral bending occurs at occiput–C1, but is almost negligible at C1–2. The greatest intervertebral motion in the spine is axial rotation at the C1–2 joint.10,11
Table 51.1 Average Rotation in Degrees at the Atlanto-occipital Joint of C0–1 According to Different Investigators
Table 51.2 Average Rotation in Degrees at the Atlanto-occipital Joint of C1–2 According to Different Investigators
The mean occipitocervical angles were 24.2°, 44.0°, and 57.2° in flexion, neutral, and extension, respectively. The mean occipitocervical distances were 21.5 mm in neutral, 28.0 mm in flexion, and 14.8 mm in extension.10 These are simple to determine on routine lateral radiographs. The differences in the occipitocervical angle and occipitocervical distance in neutral, flexion, and extension are significant. These measurements should be a valuable intraoperative tool for achieving occipitocervical fusion in appropriate alignment.
The term coupled motion denotes that motion about one axis consistently occurs simultaneously with motion about another axis (rotation or translation).12 It is most dramatic in the cervical spine. Two kinds of coupled motion are especially well known in the cervical spine: axial rotation in the same direction as applied lateral bending, and lateral bending in the same direction as applied axial rotation. Posture affects motion coupling patterns of the upper cervical spine. The most dramatic change due to modification in posture is found in coupled sagittal plane rotation, which changes from extension at extended posture to flexion at flexed posture at both levels and in response to both load types. For the axial torque, the main axial rotation and coupled lateral bending changes little with posture.
Diagnosis
In daily clinical practice, one often deals with patients with neck pain or stiffness resulting from injury or the ongoing degenerative changes rather than those with no relevant complaint. Active motion in patients with complaints of neck pain or stiffness is usually restricted by the motion-induced pain. Due to the closely related trigeminus nuclei, segmental functional disorders in the suboccipital area cause, in addition to local neck pain, frontoparietal and retro-orbital pain that can also irradiate to the upper and lower jaw. In addition, tendomyoses with trigger points and difficulties in swallowing may occur in the anterior part of the neck.13 The historical information collected from the patient is important in making the diagnosis, even given the aid of radiologic technology.
A few recognized criteria of radiology for C0–C1–C2 instability have been established. For instance, when the ADI value, which may describe the range of C1–2 translation, is larger than 3 mm in lateral flexion and extension view, the atlantoaxis is considered unstable.14 Asymmetry of the odontoid lateral mass interspace on open-mouth view is also an important clue for diagnosis. One should also assess whether vertical migration of the odontoid process exists, especially in rheumatoid patients. For evaluation of the function of the upper cervical spine, especially assessment of the range of motion, functional X-rays films are useful in addition to the clinical examination (Fig. 51.3). For the diagnosis of segmental instability, passive motion should be induced in order to obtain the full range. If anterior instability of the upper cervical spine is suspected, flexion–extension X-rays in the lateral view are appropriate. If a lesion of the alar ligaments is suspected, then lateral flexion X-ray films should be taken. In the normal situation, the atlas glides in the direction of bending, coupled by forced rotation of the axis. In cases with rotatory instability of the upper cervical spine, functional computed tomography should be performed. Atlantoaxial rotation of more than 52° should be considered pathological as a result of a lesion of the alar ligaments. For examination of the relationship between the spinal cord and bony structures or inflammatory tissue in patients with rheumatoid arthritis, functional MRIs are helpful.
Functional magnetic resonance tomography is an important diagnostic method for assessing the cervical spine in patients with rheumatoid arthritis. In particular, fusion and instabilities as well as compressions of the spinal cord or medulla often can only be detected with the help of functional MRI. Compared with static MRI examinations in patients with rheumatoid arthritis, functional magnetic resonance imaging identifies the extension of pannus tissue cranial, ventral, and dorsal to the dens with possible displacing and impinging effects on the spinal cord during flexion and extension. In addition, it is suitable for demonstration of the degree of instability in the atlanto-occipital and atlantoaxial planes. In contrast to conventional X-rays, CT, and static MRI, basilary impression as well as compressions and angulations of the spinal cord are better visualized by cinematic magnetic resonance tomography.15 Cineradiography is a valuable continuous recording tool and has been used in motion analysis of the musculoskeletal system (Fig. 51.4).16 Cineradiography has advantages over other techniques for analyzing the details of cervical spinal motion and diagnosis of spinal instability.17–20 The outcome from the cineradiographic motion analysis of the cervical spine can be taken into account to determine the level of spinal fusion for cervical spondylosis. An instantaneous center of rotation deduced from cineradiographic films has also been used to clarify the quality of motion and to justify therapy for the neck region.
Hino et al. investigated the kinematics of the normal and pathologic atlantoaxial joints by cineradiography to determine the in vivo kinematic parameters for the quantification of atlantoaxial instability.21 The kinematics of the atlantoaxial joints were evaluated by cineradiography in healthy volunteers and in patients with atlantoaxial subluxation. The results revealed the different onset points of a rapid increase in motion between flexion-from-E and extension-from-F in atlantoaxial motion. They were defined, respectively, as points A and B (Fig. 51.5). The discrepancy between these points (i.e. zone A–B) was significantly more remarkable in patients with atlantoaxial subluxation than in the volunteers. Furthermore, in most of the cases with atlantoaxial instability, subluxation occurred when the cervical spine was in a more extended position, and it was reduced in a more flexed position.
Upper cervical instability in congenital diseases
Atlantoaxial instability occurs in 10–20% of patients with Down syndrome who are at risk for atlantoaxial subluxation and subsequent complications during anesthetic induction and during positioning and manipulation associated with surgery. To identify patients who are at risk for atlantoaxial subluxation, guidelines have been adapted from the recommendations of the American Academy of Pediatrics and the Special Olympics Inc, which include preoperative neurologic assessments and cervical roentgenograms in the neutral, flexion, and extension positions. Children with an atlantodental interval of greater than 4.5 mm or with peripheral neurologic findings should have further evaluation.22 All patients with Down syndrome should have a preoperative neurologic assessment screening by the operating surgeon and/or a cervical roentgenogram in the lateral, extension, and flexion positions. Any abnormality should be investigated before surgery.
For children with Goldenhar’s syndrome, a high incidence of congenital malformations of the cervical spine, including odontoid hypoplasia, put them at particular risk during general anesthesia. Unfortunately, children with Goldenhar’s syndrome have many other malformations that would necessitate surgery under general anesthesia. Once diagnosed, children with C1–2 instability can be monitored by flexion–extension views at 6-month interval, and activity can be modified to minimize the risk of a catastrophic event. If the C1 displacement exceeds 6 mm, C1–2 fusion should be considered. The anteroposterior (AP) and lateral views of the c-spine, flexion–extension views of C1–2 and CT scan of C1–2 are recommended to assess and monitor such cervical instability.23 The similar situation also occurs in children and adolescents with cervical spine congenital synostosis as in Klippel-Feil syndrome (KFS). The more numerous the occipito-C1 abnormalities the more significant the neurologic risk.24
LOWER CERVICAL SPINE
Anatomic and biomechanical considerations
Stability and kinematics in the cervical spine depend on the integrity and configuration of several anatomic structures including the intervertebral disc, uncovertebral joints, and posterior longitudinal ligament (PLL) (Fig. 51.6). The biomechanical effects of discectomy and uncovertebral joint resection have been studied.25–28 The relative biomechanical contributions of the anterior column elements also have been explored. A clear understanding of each element’s biomechanical contribution is necessary in order to predict how surgery will affect spinal movement and stability, and to determine whether an internal fixation device is needed.
Motions in the subaxial cervical spine occurs at all levels and in all six degrees of freedom. However, most of the flexion–extension motion occurs in the central region with C5–6 considered to have the greatest range. The loss of motion due to degeneration or post-traumatic change has been observed to result in compensatory increase of motion in adjacent segments. The range of motion in axial rotation and lateral bending tend to be less in the more caudal segment.29
Intervertebral disc
With discectomy, the increase in flexion–extension ROM (10.5°) was much larger than during lateral bending (2°) or axial rotation (1.8°).30 Therefore, the disc serves more as a stabilizer against flexion and extension than as a stabilizer against lateral bending or axial rotation.
Uncovertebral joints
When the unilateral and then the contralateral uncovertebral joint was resected, the increase in flexion–extension ROM was about twice that of lateral bending or axial rotation. Thus, the uncovertebral joints also stabilize more against flexion and extension than against lateral bending or axial rotation. Flexion and extension increased more than axial rotation or lateral bending after resection of the uncovertebral joints.27,31,32 However, the uncovertebral joints contributed more to preventing lateral bending than in the other study,28 a difference perhaps attributable to different loading methods and magnitudes.
In all, the effect differs because of anatomic variations in uncovertebral joints. The major biomechanical function of uncovertebral joints includes the regulation of extension and lateral bending motion, followed by torsion, which is mainly provided by the posterior uncovertebral joints. The uncovertebral joint is also considered a primary source of cervical coupling, as are the facet joints.27 During torque to induce primarily axial rotation, uncovertebral joint resection doubled the coupled flexion and moderately increased the coupled lateral bending. The intact uncovertebral joints may therefore maintain normal spinal kinematics after discectomy.
Posterior longitudinal ligament
With the PLL resected, ROM increased significantly during flexion–extension, lateral bending, and axial rotation, all by about the same magnitude.28 The PLL therefore contributes to stability during all three motions. The slight changes in coupling with PLL resection indicate that the PLL plays no major role in spinal kinematics (Table 51.3). However, the 4 mm posterior shift in the extension IAR after PLL resection indicates that the PLL helps to maintain normal joint rotation during extension.
Clinical considerations
The intervertebral disc helps to maintain posture, as indicated by significant increases in NZ and ROM during flexion–extension after discectomy. Between tests, the destabilized spine sagged noticeably in a flexed posture with loss of lordosis. Clinically, the intervertebral space usually collapses slightly after discectomy, but the preoperative lordosis can be maintained without fusion. The strong paraspinal muscles therefore may be significant for maintaining posture and stability after surgery.33–35 After extensive discectomy without fusion, a rigid cervical orthosis can apply dorsally and rostrally directed forces to help support the paraspinal musculature in extension, thereby reducing the chance of muscle overexertion and spasm.
The uncovertebral joints appear to contribute substantially to cervical stability and kinematics, but may require resection during foraminotomy to decompress cervical nerve roots. If radiculopathic signs or symptoms are absent, surgeons should consider preserving these structures, especially during discectomy without fusion. The extent of disease will dictate the extent of decompression.36,37 However, resection of uncovertebral joints may be necessary to access osteophytes or other pathology.
Diagnosis
White and Panjabi38 developed a checklist for the diagnosis of clinical instability of the lower cervical spine based on the radiological finding and the neurological status. This systematic checklist approach is recommended as a useful method for evaluating clinical instability, given the currently available knowledge. This methodology and current knowledge about the complex problem of spinal instability have limitations. Progress will come with more biomechanical experimental studies and controlled prospective clinical studies.
Radiographic evaluation of the cervical spine begins with AP, lateral, and odontoid views. Unless the junction of C7–T1 can be adequately visualized on the cross-table lateral view, a swimmer’s view is often performed. However, in cases of trauma, a CT scan is the best method to evaluate the C7–T1 junction. After fractures and subluxations have been excluded, spinal stability may be evaluated with stressed-view radiographs such as a flexion–extension series. However, there is currently no protocol for evaluating the distal spinal stability in patients in whom traditional flexion–extension radiographs fail to visualize the important distal C7–T1 juncture. Further study of this technique as a supplement to the conventional flexion and extension views in the stressed assessment of the entire cervical spine is recommended.39
Over 3000 cases of soft tissue injuries of the cervical spine are reported annually to SUVA (Swiss accident insurance).40 Although the majority of the patients are pain free within 4 weeks, it appears that approximately one-quarter of those injured still experience neck pain even years after the accident. The initial radiological assessment should include an AP and a lateral plain X-ray of the cervical spine, and in the case of radicular symptomatology oblique views are also recommended. Should the symptoms persist for more than 6–8 weeks after the accident, functional X-rays in flexion–extension and lateral flexion should also be performed. If no instability can be demonstrated by plain X-rays and symptoms are still present and severe enough to limit the patient’s working capacity after 3–6 months despite conservative therapy, further neuroradiological investigations, including functional CTs, are indicated. The decision to perform these investigations should lie with an interdisciplinary spinal team. Close cooperation between the clinicians and the radiologists is of utmost importance to ensure that the optimal radiological investigation can be performed on the basis of the clinical findings.
The study by Kanayama et al.41 serves as the first investigation to quantify cervical motion patterns in normal and pathologic spines using cineradiography technique. Different cervical motion patterns were observed in spines of normal subjects and those of patients with cervical instability. The normal cervical motion pattern consisted of well-regulated stepwise motions that initiated at the C1–2 segment and transmitted to the lower cervical segments. These results were similar to the dynamic motion data in the lumbar and lumbosacral regions. Kanayama et al.41 have recently reported in their cineradiographic study that lumbar segmental motions occur not simultaneously but stepwise from the upper level, with motion lags during flexion. In pathologic cervical spines, however, cervical motion initiated at the unstable levels. In rheumatoid arthritis patients with atlantoaxial subluxation, motion in the unstable C1–2 segment initiate significantly earlier than that in the C2–3 segment. In spines with subaxial instability, motion in the unstable segments preceded that in the upper intact segments.
Ogon et al.42 recently performed an in vitro dynamic motion analysis using human lumbar spine specimens. They observed discontinuous acceleration and deceleration during intersegmental motion, termed a ‘jerk.’ In the intact functional spinal unit, this dynamic motion parameter is located at the neutral position. However, the jerk shifts from the neutral position toward the beginning of the motion in increasing instability. They concluded this jerk shift is a sign of spinal instability. The current results in pathologic cervical spines are consistent with the jerk shift occurring toward the beginning of motion.
In a clinical setting, functional radiographs are commonly used to evaluate spinal instability and treatment results such as solidity of spinal fusion. However, Woesner and Mitts43 documented that some cervical abnormalities are not visible on conventional radiographs but are detected by cineradiography. Brunton et al.44 also reported that cineradiography is the more accurate diagnostic technique for determining the level indicated for anterior fusion. Although further investigations are required, the cineradiographic method provides the capability of diagnosing and evaluating spinal instability that cannot be identified by conventional radiographic examination.
Surgical indications and principle of internal fixation
Numerous fixation techniques and devices have been developed over the years for stabilizing the lower cervical spine. The first techniques introduced were for posterior fixation. The wire technique introduced by Gallie for the upper cervical spine was also applicable in a modified way for use in the lower cervical spine. The posterior wire technique has been developed over the years, introducing different types of cables secured to the posterior lamina and spinous process,45–48 and is still the operative method of choice in many spine centers. However, these techniques provide for one-point fixation and depend on a structural bone graft.
Posterior wiring provided significantly better flexion stability in two- rather than three-column disruptions.49 Posterior wiring reduced posterior displacement in two-column partial disruptions to 25% of control. In three-column dissociations, posterior wiring only reduced posterior displacement to 50% of control. In extension, posterior wiring was ineffective in preventing displacement. Anterior plating, used alone, tolerated only 37% of the maximum flexion moment before early failure. On the other hand, combined anterior plating and posterior Roger’s wiring reduced posterior displacement in flexion to 20% of control, while reducing anterior displacement in extension to 50% of control. After the insertion of the bone graft, a significant decrease in motion was seen in the effected segment in extension (±45.9%), with similar reductions in lateral bending and axial rotation and a smaller reduction in flexion. The application of an anterior metal plate in addition to the bone graft at the injured level provided significant reduction in motion (±70%) in all load modalities. These data may have clinical relevance regarding the role of internal fixation in cases of severe spine instability26 The stiffness of the simulated bone graft construct decreased progressively during flexion and lateral bending after each foraminotomy (p<0.05). Increased bone graft height of 79% returned stability to the preforaminotomy level.28 To avoid this, various plates were developed for posterior fixation of the cervical spine.
GUIDELINES OF THE DIAGNOSIS FOR CERVICAL INSTABILITY
The following recommendations are a series of evidence-based guidelines for the safest and most effective means for identifying significant injuries of the cervical spine following trauma. Injuries which are most likely to lead to neurologic damage by causing or exacerbating trauma to the spinal cord are a particular focus, including bony, ligamentous, and other soft tissue abnormalities. Trauma patients at risk can be categorized according to their clinical presentation into four categories that are at special risk for various types of injuries, or at minimal or no risk for injury. The following guidelines present category of patients and recommendations specific to those categories.50–53 See also Figure 51.7.
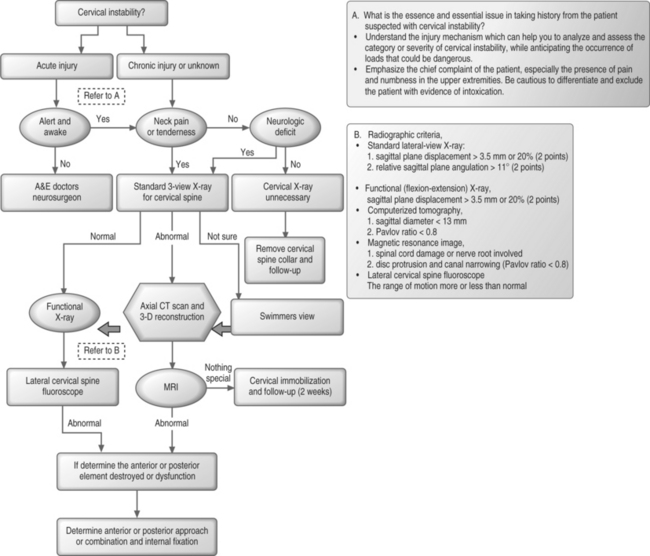
Fig. 51.7 Standardized flowchart for diagnosis of cervical instability.
1. Alert, awake, not intoxicated, neurologically normal, no midline neck pain or tenderness even with full range of motion of neck and palpation of cervical spine54,55
Guidelines – general
2. Alert, awake, complaints of neck pain
Guidelines
4 Altered mental status and return of normal mental status not anticipated for 2 days or more (e.g. severe traumatic or hypoxic, ischemic brain injury)
Guidelines
Addendum
1 White AA, Panjabi MM. Biomechanics of spine, 2nd edn. Philadelphia: JB Lippincott, 1990.
2 Allen BL, Ferguson RL, Lehmann TR, et al. A mechanistic classification of closed, indirect fractures and dislocations of lower cervical spine. Spine. 1982;7(1):1-27.
3 Larson SL. Vertebral injury and instability. In: Holtzman RNN, editor. Spinal instability. New York: Springer; 1993:101-137.
4 Larson SJ, Maiman DJ. Surgery of the lumbar spine. New York: Thieme, 1999;334.
5 Holdsworth H. Fractures, dislocations and fractures–dislocations of the spine. J Bone Joint Surg [Br]. 1963;B45:6-20.
6 Denis F. Three-column spine and its significance in classification of acute thoracolumbar spinal injuries. Spine. 1983;8:817-831.
7 Louis R. Spinal stability as defined by three-column spine concept. Anat Clin. 1985;7:33-42.
8 White AIII, Johnson R, Panjabi M, et al. Biomedical analysis of clinical stability in cervical spine. Clin Orthop. 1975;109:85-95.
9 Panjabi M, Dvorak J, Crisco JJ3rd, et al. Effects of alar ligament transection on upper cervical spine rotation. J Orthop Res. 1991;9(4):584-593.
10 Phillips FM, Phillips CS, Wetzel FT, et al. Occipitocervical neutral position. Possible surgical implications. Spine. 1999;24(8):775-778.
11 Panjabi M, Dvorak J, Duranceau J, et al. Three-dimensional movements of the upper cervical spine. Spine. 1988;13(7):726-730.
12 Panjabi MM, Oda T, Crisco JJ3rd, et al. Posture affects motion coupling patterns of the upper cervical spine. J Orthop Res. 1993;11(4):525-536.
13 Baumgartner H. The upper cervical spine. Symptomatology, clinical diagnosis and therapy of functional disorder. Orthopade. 1991;20(2):127-132.
14 Dvorak J. Functional roentgen diagnosis of the upper cervical spine. Orthopade. 1991;20(2):121-126.
15 Allmann KH, Schafer O, Uhl M, et al. Kinematic versus static MRI study of the cervical spine in patients with rheumatoid arthritis. Rofo Fortschr Geb Rontgenstr Neuen Bildgeb Verfahr. 1999;170(1):22-27.
16 Neer CS, Foster CR. Inferior capsular shift for involuntary inferior and multi-directional instability of the shoulder. J Bone Joint Surg [Am]. 1980;62:897-907.
17 Fielding JM. Cineradiography of the normal cervical spine. J Bone Joint Surg [Am]. 1957;39:1280-1288.
18 Brunton FJ, Wilkinson JA, Wise KSH, et al. Cineradiography in cervical spondylosis as a mean of determining the level for anterior fusion. J Bone Joint Surg [Br]. 1982;64:399-404.
19 van Mameren H, Drukker J, Sanches H, et al. Cervical spine motion in the sagittal plane (I). Range of motion of actually performed movements, an X-ray cineradiographic study. Eur J Morphol. 1990;28:47-68.
20 Hino H, Abumi K, Kanayama M, et al. Dynamic motion analysis of normal and unstable cervical spine using cineradiography: an in vivo study. Spine. 1999;24:163-168.
21 Hino H, Abumi K, Kanayama M, et al. Dynamic motion analysis of normal and unstable cervical spines using cineradiography: an in vivo study. Spine 24(2):163–168.
22 Harley EH, Collins MD. Neurologic sequelae secondary to atlantoaxial instability in Down syndrome. Implications in otolaryngologic surgery. Arch Otolaryngol Head Neck Surg. 1994;120(2):159-165.
23 Healey D, Letts M, Jarvis JG. Cervical spine instability in children with Goldenhar’s syndrome. Can J Surg. 2002;45(5):341-344.
24 Rouvreau P, Glorion C, Langlais J, et al. Assessment and neurologic involvement of patients with cervical spine congenital synostosis as in Klippel-Feil syndrome: study of 19 cases. J Pediatr Orthop B. 1998;7(3):179-185.
25 Richter M, Wilke HJ, Kluger P, et al. Load-displacement properties of the normal and injured lower cervical spine in vitro. Eur Spine J. 2000;9(2):104-108.
26 Ng HW, Teo EC, Lee KK, et al. Finite element analysis of cervical spinal instability under physiologic loading. J Spinal Disord Tech. 2003;16(1):55-65.
27 Penning L, Wilmink JT. Rotation of the cervical spine: A CT study in normal subjects. Spine. 1987;12:732-738.
28 Panjabi MM, Vasavada A, White AAIII. Cervical spine biomechanics. Semin Spine Surg. 1993;5:10-16.
29 Dai L. Disc degeneration and cervical instability. Zhonghua Wai Ke Za Zhi. 1999;37(3):180-182.
30 Schulte K, Clark CR, Goel VK. Kinematics of the cervical spine following discectomy and stabilization. Spine. 1989;14(10):1116-1121.
31 Kotani Y, McNulty PS, Abumi K, et al. The role of anteromedial foraminotomy and the uncovertebral joints in the stability of the cervical spine: A biomechanical study. Spine. 1998;23:1559-1565.
32 Clausen JD, Goel VK, Traynelis VC, et al. Uncinate processes and Luschka joints influence the biomechanics of the cervical spine: Quantification using a finite element model of the C5–C6 segment. J Orthop Res. 1997;15:342-347.
33 Sonntag VKH, Klara P. Controversy in spine care: Is fusion necessary after anterior cervical discectomy? Spine. 1996;21:1111-1113.
34 Nolan JPJr, Sherk HH. Biomechanical evaluation of the extensor musculature of the cervical spine. Spine. 1988;13:9-11.
35 Schulte K, Clark CR, Goel VK. Kinematics of the cervical spine following discectomy and stabilization. Spine. 1989;14:1116-1121.
36 Hadley MN, Sonntag VKH. Cervical disc herniations: The anterior approach to symptomatic interspace pathology. Neurosurg Clin North Am. 1993;4:45-52.
37 Denis F. The three column spine and its significance in the classification of acute thoracolumbar spinal injuries. Spine. 1983;8:817-831.
38 White AA3rd, Panjabi MM. Update on the evaluation of instability of the lower cervical spine. Instr Course Lect. 1987;36:513-520.
39 Davidorf J, Hoyt D, Rosen P. Distal cervical spine evaluation using swimmer’s flexion–extension radiographs. J Emerg Med. 1993;11(1):55-59.
40 Dvorak J. Radiological assessment protocol in injuries of the cervical vertebrae. Schweiz Med Wochenschr. 1990;120(51–52):1989-1998.
41 Kanayama M, Abumi K, Kaneda K, et al. Phase lag of the intersegmental motion in flexion–extension of the lumbar and lumbosacral spine. An in vivo study. Spine. 1996;21:1416-1422.
42 Ogon M, Bender BR, Hooper DM, et al. A dynamic approach to spinal instability. Part II: Hesitation and giving-way during interspinal motion. Spine. 1997;22:2859-2866.
43 Woesner ME, Mitts MG. The evaluation of cervical spine motion below C2: A comparison of cineroentgenographic and conventional roentgenographic methods. Am J Roentgenol. 1972;115:148-154.
44 Brunton FJ, Wilkinson JA, Wise KSH, et al. Cineradiography in cervical spondylosis as a means of determining the level for anterior fusion. J Bone Joint Surg [B]. 1982;64:399-404.
45 Bohlman HH. The management of cervical spine fractures and dislocation. American Academy of Orthopedic Surgeons. Instruction Course Lecture, vol 34.. CV Mosby, St. Louis, 1985;163-187.
46 Davey JR, Rorabeck CH, Bailey SI, et al. A technique of posterior cervical fusion for instability of cervical spine. Spine. 1985;10:722-728.
47 Stathoulis B, Govender S. The triple wire technique for bifacet dislocation of the cervical spine. Injury. 1997;28(2):123-125.
48 Weis JC, Cunningham BW, Kanayama M, et al. In vitro biomechanical comparison of multistrand cables with conventional cervical stabilization. Spine. 1996;21(18):2108-2114.
49 McLain RF, Aretakis A, Moseley TA, et al. Sub-axial cervical dissociation. Anatomic and biomechanical principles of stabilization. Spine. 1994;19(6):653-659.
50 Ajani AE, Cooper DJ, Scheinkestel CD, et al. Optimal assessment of cervical spine trauma in critically ill patients: a prospective evaluation. Anaesth Intensive Care. 1998;26:487-491.
51 Beirne JC, Butler PE, Brady FA. Cervical spine injuries in patients with facial fractures: a 1-year prospective study. Int J Oral Maxillofac Surg. 1995;24:26-29.
52 Blacksin MF, Lee HJ. Frequency and significance of fractures of the upper cervical spine detected by CT in patients with severe neck trauma. Am J Roentgenol. 1995;165:1201-1204.
53 Davis JW, Parks SN, Detlefs CL, et al. Clearing the cervical spine in obtunded patients: the use of dynamic fluoroscopy. J Trauma. 1995;39:435-438.
54 Feldborg Nielsen C, Annertz M, Persson L, et al. Fusion or stabilization alone for acute distractive flexion injuries in the mid to lower cervical spine? Eur Spine J 199; 6(3):197–202.
55 Jonsson HJr, Cesarine K, Petren-Mallmin M, et al. Locking screw-plate fixation of cervical spine fractures with and without ancillary posterior plating. Arch Orthop Trauma Surg. 1991;111(1):1-12.