CHAPTER 23 Cerebral hemisphere
The two cerebral hemispheres are the largest major divisions of the brain. Each hemisphere consists of an external highly-convoluted cortex, beneath which lies an extensive internal mass of white matter that partly encloses the basal ganglia. Each hemisphere also contains a lateral ventricle that is continuous with the third ventricle through the interventricular foramen. The two hemispheres are linked by the commissural fibres of the corpus callosum.
The cerebral hemispheres are separated by a deep median cleft, the great longitudinal fissure, which contains a crescent-shaped fold of the dura mater, the falx cerebri (see Ch. 27).
GYRI, SULCI AND LOBES
The surface of the cerebral hemisphere shows a complex pattern of convolutions, or gyri, which are separated by furrows of varying depth known as fissures, or sulci (Fig. 23.1, Fig. 23.2). Some of these are consistently located, others less so. They provide part of the basis for the division of the hemisphere into lobes. The frontal, parietal, temporal and occipital lobes approximately correspond in surface extent to the overlying cranial bones from which they take their names. The anterior and posterior extremities of the hemispheres form the frontal and occipital poles respectively, and the temporal pole is the anterior extremity of the temporal lobe.
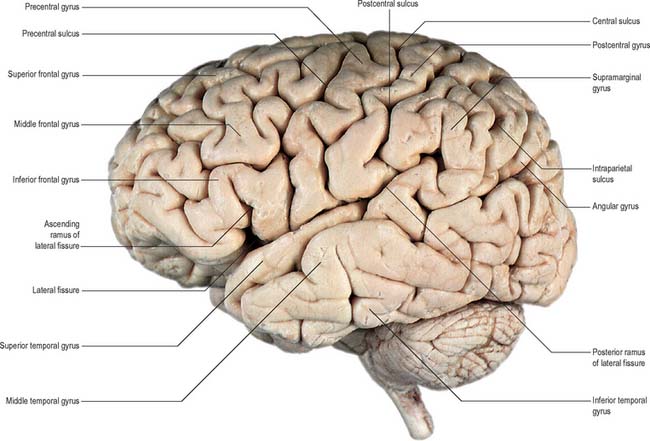
Fig. 23.1 Lateral aspect of the left cerebral hemisphere indicating the major gyri and sulci.
(Dissection by EL Rees; photograph by Kevin Fitzpatrick on behalf of GKT School of Medicine, London; figure enhanced by B. Crossman.)
The posterior region of the medial surface is traversed by the parieto-occipital and the calcarine sulci. These two deep sulci converge anteriorly to meet a little posterior to the splenium. The parieto-occipital sulcus marks the boundary between parietal and occipital lobes. It starts on the superomedial margin of the hemisphere approximately 5 cm anterior to the occipital pole, sloping down and slightly forwards to the calcarine sulcus. The calcarine sulcus starts near the occipital pole. Though usually restricted to the medial surface, its posterior end may reach the lateral surface. Directed anteriorly, it joins the parieto-occipital sulcus at an acute angle behind the splenium. Continuing forwards, it crosses the inferomedial margin of the hemisphere, and forms the inferior boundary of the isthmus, which connects the cingulate with the parahippocampal gyrus. The visual cortex lies above and below the posterior part of the calcarine sulcus, behind the junction with the parieto-occipital. The calcarine sulcus is deep and produces an elevation, the calcar avis, in the wall of the posterior horn of the lateral ventricle.
The inferior cerebral surface is divided by the stem of the lateral fissure into a small anterior and larger posterior part (Fig. 23.3, Fig. 23.4). The anterior part constitutes the orbital region of the frontal lobe. It is concave and lies above the cribriform plate of the ethmoid, the orbital plate of the frontal, and the lesser wing of the sphenoid bone. A rostrocaudal olfactory sulcus traverses it near its medial margin, overlapped by the olfactory bulb and tract. The medial strip thus demarked is the gyrus rectus. The rest of this surface bears irregular orbital sulci, generally H-shaped, which divide it into the anterior, medial, posterior and lateral orbital gyri.
The larger, posterior region of the inferior cerebral surface is partly superior to the tentorium but also to the middle cranial fossa. The collateral sulcus starts near the occipital pole, and extends anteriorly and parallel to the calcarine sulcus, separated from it by the lingual gyrus. The lingual gyrus, between the calcarine and collateral sulci, passes into the parahippocampal gyrus, which begins at the isthmus where it is continuous with the cingulate gyrus. Anteriorly, the parahippocampal gyrus continues into the hook-shaped uncus, which lies lateral to the midbrain and posterolateral to the anterior perforated substance. The uncus is part of the piriform cortex of the olfactory system, phylogenetically one of the oldest parts of the cortex, and is separated from the temporal pole by the rhinal sulcus (fissure) which marks the lateral limit of the entorhinal cortex (area) (Fig. 23.4).
CEREBRAL CORTEX
MICROSTRUCTURE
The microscopic structure of the cerebral cortex is an intricate blend of nerve cells and fibres, neuroglia and blood vessels. The neocortex essentially consists of three neuronal cell types. Pyramidal cells are the most abundant. Non-pyramidal cells, also called stellate or granule cells are divided into spiny and non-spiny neurones. All types have been subdivided on the basis of size and shape (Fig. 23.5).
Spiny stellate cells are the second most numerous cell type in the neocortex and for the most part occupy lamina IV. They have relatively small multipolar cell bodies, commonly 6 to 10 μm in diameter. Several primary dendrites, profusely covered in spines, radiate for variable distances from the cell body. Their axons ramify within the grey matter predominantly in the vertical plane. Spiny stellate cells probably use glutamate as their neurotransmitter.
Laminar organization
Typical neocortex is described as having six layers or laminae lying parallel to the surface (Fig. 23.6).
I The molecular or plexiform layer is cell sparse, containing only scattered horizontal cells and their processes enmeshed in a compacted mass of tangential, principally horizontal axons and dendrites. These are afferent fibres, which arise from outside the cortical area, together with intrinsic fibres from cortical interneurones, and the apical dendritic arbors of virtually all pyramidal neurones of the cerebral cortex. In histological sections stained to show myelin, layer I appears as a narrow horizontal band of fibres.
II The external granular lamina contains a varying density of small neuronal cell bodies including both small pyramidal and non-pyramidal cells; the latter may predominate. Myelin fibre stains show mainly vertically arranged processes traversing the layer.
III The external pyramidal lamina contains pyramidal cells of varying sizes, together with scattered non-pyramidal neurones. The size of the pyramidal cells is smallest in the most superficial part of the layer and greatest in the deepest part. This lamina is frequently further subdivided into IIIa, IIIb and IIIc, with IIIa most superficial and IIIc deepest. As in layer II, myelin stains reveal a mostly vertical organization of fibres.
IV The internal granular lamina is usually the narrowest of the cellular laminae. It contains densely packed, small, round cell bodies of non-pyramidal cells, notably spiny-stellate cells and some small pyramidal cells. Within the lamina, in myelin stained sections, a prominent band of horizontal fibres (outer band of Baillarger) is seen.
V The internal pyramidal (ganglionic) lamina typically contains the largest pyramidal cells in any cortical area, though actual sizes vary considerably from area to area. Scattered non-pyramidal cells are also present. In myelin stains, the lamina is traversed by ascending and descending vertical fibres, and also contains a prominent central band of horizontal fibres (inner band of Baillarger).
VI The multiform (or fusiform/pleiomorphic) layer consists of neurones with a variety of shapes, including recognizable pyramidal, spindle, ovoid and many other shaped somata. Typically, most cells are small to medium in size. This lamina blends gradually with the underlying white matter, and a clear demarcation of its deeper boundary is not always possible.
Five regional variations are described in neocortical structure (Fig. 23.7). While all are said to develop from the same six-layered pattern, two types, granular and agranular, are regarded as virtually lacking certain laminae, and are referred to as heterotypical. Homotypical variants, in which all six laminae are found, are called frontal, parietal and polar, names that link them with specific cortical regions in a somewhat misleading manner (e.g. the frontal type also occurs in parietal and temporal lobes).
It is customary to refer to some discrete cortical territories not only by their anatomical location in relation to gyri and sulci, but also in relation to their cytoarchitectonic characteristics (Brodmann’s areas) (Fig. 23.8). Some of the areas so defined, e.g. the primary sensory and motor cortices, have clear relevance in terms of anatomical connections and functional significance, others less so.
OVERVIEW OF CORTICAL CONNECTIVITY
The primary somatosensory, visual and auditory areas give rise to ipsilateral corticocortical connections to the association areas of the parietal, occipital and temporal lobes, respectively, which then progressively project towards the medial temporal limbic areas, notably the parahippocampal gyrus, entorhinal cortex and hippocampus. Thus, the first (primary) somatic sensory area (SI) projects to the superior parietal cortex (Brodmann’s area 5), which in turn projects to the inferior parietal cortex (area 7). From here connections pass to cortex in the walls of the superior temporal sulcus, and so on to the posterior parahippocampal gyrus, and on into limbic cortex. Similarly, for the visual system, the primary visual cortex (area 17) projects to the parastriate cortex (area 18), which in turn projects to the peristriate region (area 19). Information then flows to inferotemporal cortex (area 20), to cortex in the walls of the superior temporal sulcus, then to medial temporal cortex in the posterior parahippocampal gyrus, and so to limbic areas. The auditory system shows a similar progression from primary auditory cortex to temporal association cortex and so to the medial temporal lobe.
FRONTAL LOBE
The frontal lobe is the rostral region of the hemisphere, anterior to the central sulcus and above the lateral fissure. The precentral gyrus runs parallel to the central sulcus on the superolateral surface and extends onto the medial surface, and is limited anteriorly by the precentral sulcus. The area of the frontal lobe anterior to the precentral sulcus is divided into the superior, middle and inferior frontal gyri (Fig. 23.1). The frontal pole lies in front of these gyri. The ventral surface of the frontal lobe, the orbitofrontal cortex, overlies the bony orbit. The medial surface extends from the frontal pole to the paracentral lobule and consists of the medial frontal cortex and the anterior cingulate cortex.
Primary motor cortex
The primary motor cortex (MI) corresponds to the precentral gyrus (area 4), and is the area of cortex with the lowest threshold for eliciting contralateral muscle contraction by electrical stimulation. It contains a detailed topographically organized map (motor homunculus) of the opposite body half, with the head represented most laterally, and the leg and foot represented on the medial surface of the hemisphere in the paracentral lobule (Fig. 23.9). A striking feature is the disproportionate representation of body parts in relation to their physical size: large areas represent the muscles of the face and hand, which are capable of finely controlled or fractionated movements.
Premotor cortex
Immediately in front of the primary motor cortex lies Brodmann’s area 6. It extends onto the medial surface, where it becomes contiguous with area 24 in the cingulate gyrus, anterior and inferior to the paracentral lobule. A number of functional motor areas are contained within this cortical region. Lateral area 6, the area over most of the lateral surface of the hemisphere, corresponds to the premotor cortex.
Frontal eye field
The frontal eye field corresponds to parts of Brodmann’s areas 6, 8 and 9 (Fig. 23.10). It receives its major thalamic projection from the parvocellular mediodorsal nucleus, with additional afferents from the medial pulvinar, the ventral anterior nucleus and the suprageniculate–limitans complex, and connects with the paracentral nucleus of the intralaminar group. The thalamocortical pathways to the frontal eye field form part of a pathway from the superior colliculus, the substantia nigra and the dentate nucleus of the cerebellum. The frontal eye field has extensive ipsilateral corticocortical connections, receiving fibres from several visual areas in the occipital, parietal and temporal lobes, including the medial temporal area (V5) and area 7a. There is also a projection from the superior temporal gyrus, which is auditory rather than visual in function. From within the frontal lobe, the frontal eye field receives fibres from the ventrolateral and dorsolateral prefrontal cortices. It projects to the dorsal and ventral premotor cortices and to the medial motor area, probably to the supplementary eye field adjacent to the supplementary motor area proper. It projects prominently to the superior colliculus, to the pontine gaze centre within the pontine reticular formation, and to other oculomotor related nuclei in the brain stem. As its name implies, it is important in the control of eye movements.
Supplementary motor cortex
Stimulation of the supplementary motor area in conscious patients has been reported to elicit the sensation of an urge to move, or of anticipation that a movement is about to occur. A region anterior to the supplementary motor area for face representation (areas 44, 45) is important in vocalization and speech production (Fig. 23.10).
Prefrontal cortex
The prefrontal cortex on the lateral surface of the hemisphere comprises predominantly Brodmann’s areas 9, 46 and 45 (Fig. 23.8). In non-human primates, two subdivisions of the lateral prefrontal cortex are recognized, a dorsal area equivalent to area 9, and perhaps including the superior part of area 46, and a ventral area, consisting of the inferior part of area 46 and area 45. Areas 44 and 45 are particularly notable in man since, in the dominant hemisphere, they constitute the motor speech area (Broca’s area). Both the dorsolateral and ventrolateral prefrontal areas receive their major thalamic afferents from the mediodorsal nucleus, and there are additional contributions from the medial pulvinar, the ventral anterior nucleus and from the paracentral nucleus of the anterior intralaminar group. The dorsolateral area receives long association fibres from the posterior and middle superior temporal gyrus (including auditory association areas), from parietal area 7a, and from much of the middle temporal cortex. From within the frontal lobe it also receives projections from the frontal pole (area 10), and from the medial prefrontal cortex (area 32) on the medial surface of the hemisphere. It projects to the supplementary motor area, the dorsal premotor cortex and the frontal eye field. All these thalamic and corticocortical connections are reciprocal. Commissural connections are with the homologous area, and with the contralateral inferior parietal cortex. The ventrolateral prefrontal area receives long association fibres from both area 7a and area 7b of the parietal lobe, from auditory association areas of the temporal operculum, from the insula and from the anterior part of the lower bank of the superior temporal sulcus. From within the frontal lobe it receives fibres from the anterior orbitofrontal cortex and projects to the frontal eye field and the ventral premotor cortex. It connects with the contralateral homologous area via the corpus callosum. These connections are probably all reciprocal.
PARIETAL LOBE
The lateral aspect of the parietal lobe is divided into three areas by postcentral and intraparietal sulci (Fig. 23.1). The postcentral sulcus, often divided into upper and lower parts, is posterior and parallel to the central sulcus. Inferiorly, it ends above the posterior ramus of the lateral fissure. The postcentral gyrus or primary somatosensory cortex lies between the central and postcentral sulci. Posterior to the postcentral sulcus there is a large area, subdivided by the intraparietal sulcus. It usually starts in the postcentral sulcus near its midpoint and extends posteroinferiorly across the parietal lobe, dividing the latter into superior and inferior parietal lobules.
Somatosensory cortex
The primary somatosensory cortex contains within it a topographical map of the contralateral half of the body. The face, tongue and lips are represented inferiorly, the trunk and upper limb are represented on the superolateral aspect, and the lower limb on the medial aspect of the hemisphere, giving rise to the familiar ‘homunculus’ map (Fig. 23.9).
Superior and inferior parietal lobules
Posterior to the postcentral gyrus, the superior part of the parietal lobe is composed of areas 5, 7a and 7b (Fig. 23.8). Area 5 receives a dense feedforward projection from all cytoarchitectonic areas of SI in a topographically organized manner. The thalamic afferents to this area come from the lateral posterior nucleus and from the central lateral nucleus of the intralaminar group. Ipsilateral corticocortical fibres from area 5 go to area 7, the premotor and supplementary motor cortices, the posterior cingulate gyrus, and the insular granular cortex. Commissural connections between area 5 on both sides tend to avoid the areas of representation of the distal limbs. The response properties of cells in area 5 are more complex than in SI, with larger receptive fields and evidence of submodality convergence. Area 5 contributes to the corticospinal tract.
TEMPORAL LOBE
The three parallel gyri on the lateral surface of the temporal lobe are the superior (area 22); middle (area 21); and inferior (area 20) temporal gyri. The temporal pole (area 38) lies in front of the termination of these gyri. Along its superior margin the superior temporal gyrus is continuous with gyri in the floor of the posterior ramus of the lateral sulcus. These vary in number, and extend obliquely anterolaterally from the circular sulcus around the insula as the transverse temporal gyri of Heschl (Fig. 23.11). The anterior transverse temporal gyrus and adjoining part of the superior temporal gyrus are auditory in function, and are considered to be Brodmann’s area 42. The anterior gyrus is approximately area 41.
Auditory cortex
The inferior temporal cortex, area 20, is a higher visual association area. The posterior inferior temporal cortex receives major ipsilateral corticocortical fibres from occipitotemporal visual areas, notably V4. It contains a coarse retinotopic representation of the contralateral visual field, and sends a major feedforward pathway to the anterior part of the inferior temporal cortex. The anterior inferior temporal cortex projects onto the temporal pole and to paralimbic areas on the medial surface of the temporal lobe. Additional ipsilateral association connections of the inferior temporal cortex are with the anterior middle temporal cortex, in the walls of the superior temporal gyrus, and with visual areas of the parietotemporal cortex. Frontal lobe connections are with area 46 in the dorsolateral prefrontal cortex (posterior inferior temporal), and with the orbitofrontal cortex (anterior inferior temporal). The posterior area also connects with the frontal eye field. Reciprocal thalamic connections are with the pulvinar nuclei; the posterior part is related mainly to the inferior and lateral nuclei, and the anterior part to the medial and adjacent lateral pulvinar. Intralaminar connections are with the paracentral and central medial nuclei. Other subcortical connections conform to the general pattern of all cortical regions. Callosal connections are between corresponding areas and the adjacent visual association areas of each hemisphere.
INSULA
The insula (Fig. 23.12) lies deep in the floor of the lateral fissure, almost surrounded by a circular sulcus, and overlapped by adjacent cortical areas, the opercula. The frontal operculum is between the anterior and ascending rami of the lateral fissure, forming a triangular division of the inferior frontal gyrus. The frontoparietal operculum, between ascending and posterior rami of the lateral fissure, consists of the posterior part of the inferior frontal gyrus, the lower ends of the precentral and postcentral gyri, and the lower end of the anterior part of the inferior parietal lobule. The temporal operculum, below the posterior ramus of the lateral fissure, is formed by superior temporal and transverse temporal gyri. Anteriorly, the inferior region of the insula adjoins the orbital part of the inferior frontal gyrus.
Claustrum
The claustrum (see Fig. 23.27) is a thin sheet of grey matter lying deep to the insula. It is approximately coextensive with the insula, from which it is separated by the extreme capsule. Medially, the claustrum is separated from the putamen by the external capsule. It is thickest anteriorly and inferiorly, where it becomes continuous with the anterior perforated substance, amygdala and prepiriform cortex. In animals, it has reciprocal, topographically organized, connections with many regions of the neocortex. Little is known about the connections and functional significance of the claustrum in the human brain.
OCCIPITAL LOBE
The occipital lobe lies behind an arbitrary line joining the preoccipital incisure and the parieto-occipital sulcus (Fig. 23.1, Fig. 23.2) and is comprised almost entirely of Brodmann’s areas 17, 18 and 19. Area 17, the striate cortex, is the primary visual cortex (VI). A host of other distinct visual areas reside in the occipital and temporal cortex. Functional subdivisions V2, V3 (dorsal and ventral) and V3A lie within Brodmann’s area 18. Other functional areas at the junction of the occipital cortex with the parietal or temporal lobes lie wholly or partly in area 19.
The primary visual cortex receives afferent fibres from the lateral geniculate nucleus via the optic radiation (see Fig. 23.27). The latter curves posteriorly and spreads through the white matter of the occipital lobe. Its fibres terminate in strict point-to-point fashion in the striate area. The cortex of each hemisphere receives impulses from two hemi-retinae, which represent the contralateral half of the binocular visual field. Superior and inferior retinal quadrants are connected with corresponding areas of the striate cortex. Thus, the superior retinal quadrants (representing the inferior half of the visual field) are connected with the visual cortex above the calcarine sulcus, and the inferior retinal quadrants (representing the upper half of the visual field) are connected with the visual cortex below the calcarine sulcus. The peripheral parts of the retinae activate the most anterior parts in the visual cortex. The macula impinges upon a disproportionately large posterior part around the occipital pole.
The striate cortex is granular. Layer IV, bearing the stria of Gennari, is commonly divided into three sublayers. Passing from superficial to deep, these are IVA, IVB (which contains the stria), and IVC. The densely cellular IVC is further subdivided into a superficial IVCα and a deep IVCβ. Layer IVB contains only sparse, mainly non-pyramidal, neurones. The input to area 17 from the lateral geniculate nucleus terminates predominantly in layers IVA and IVC. Other thalamic afferents, from the inferior pulvinar nucleus and the intralaminar group, pass to layers I and VI. Geniculocortical fibres terminate in alternating bands. Axons from geniculate laminae which receive information from the ipsilateral eye (laminae 2, 3 and 5), are segregated from those of laminae which receive input from the contralateral eye (laminae 1, 4 and 6). Neurones within layer IVC are monocular, i.e. they respond to stimulation of either the ipsilateral or contralateral eye, but not both. This horizontal segregation forms the anatomical basis of the ocular dominance column in that neurones encountered in a vertical strip from pia to white matter, although binocular outside layer IV, exhibit a preference for stimulation of one or other eye. The other major functional basis for visual cortical columnar organization is the orientation column. This describes the observation that an electrode passing through the depth of the cortex at right angles to the plane from pia to white matter, encounters neurones that all respond preferentially to either a stationary or a moving straight line of a given orientation within the visual field. Cells with simple, complex and hypercomplex receptive fields occur in area 17. Simple cells respond optimally to lines in a narrowly defined position. Complex cells respond to a line anywhere within a receptive field, but with a specific orientation. Hypercomplex cells are similar to complex cells except that the length of the line or bar stimulus is also critical for an optimal response. There is a relationship between the complexity of response and the position of cells in relation to the cortical laminae. Simple cells are mainly in layer IV and complex and hypercomplex cells predominate in either layers II and III or layers V and VI.
LIMBIC LOBE
The limbic lobe (Fig. 23.13) includes large parts of the cortex on the medial wall of the hemisphere, principally the subcallosal, cingulate and parahippocampal gyri. It also includes the hippocampal formation, which consists of the hippocampus proper (Ammon’s horn or ‘cornu ammonis’), the dentate gyrus, the subicular complex (subiculum, presubiculum, parasubiculum) and the entorhinal cortex (area 28). There is a close relationship between these phylogenetically old cortical structures and the termination of the olfactory tract in the frontal and medial temporal lobes.
On the basis of emotional disturbances displayed by patients who presented with damage to the hippocampus and cingulate gyrus, Papez (1937) described a closed circuit (the Papez circuit), which linked the hippocampus with the cingulate cortex, via the mammillary bodies and anterior thalamus. He proposed that emotional expression is organized in the hippocampus, experienced in the cingulate gyrus and expressed via the mammillary bodies. The hypothalamus was considered to be the site where hippocampal processes gain access to the autonomic outflow that controls the peripheral expression of emotional states. The Papez circuit is now widely accepted to be involved with cognitive processes, including mnemonic functions and spatial short-term memory.
The cingulate gyrus is the area that shows the most consistent pain-evoked changes in synaptic activity related to regional cerebral blood flow (rCBF) as measured by either positron emission tomography (PET) or functional magnetic resonance imaging (fMRI) (Derbyshire et al 1997). It must be remembered that pain evokes a multidimensional response in the brain including cognitive, emotional, autonomic and motor components, and so it is not always possible to assign specific functions to parts of the brain that generate PET or fMRI signals in response to pain (Peyron et al 2000). However, in many experimental paradigms, a combination of signals from the cingulate gyrus, somatosensory area SII and the insula appears to be involved in the conscious appreciation of nociception and neuropathic pain.
Olfactory pathways
The organization of the olfactory system reflects its phylogenetically ancient lineage: afferent olfactory pathways proceed directly to the cerebral cortex, bypassing the thalamus, and its terminal fields are primitive cortical areas that are considered to be parts of the limbic system. Details of the relationship between the olfactory pathways and the limbic system are shown in Fig. 23.4.
The olfactory nerves arise from olfactory receptor neurones in the olfactory mucosa (see Ch. 32). The axons collect into numerous small bundles ensheathed by a population of unique glia and surrounded by layers of meninges, and enter the anterior cranial fossa by passing through the foramina in the cribriform plate of the ethmoid bone. They attach to the inferior surface of the olfactory bulb, which is situated at the anterior end of the olfactory sulcus on the orbital surface of the frontal lobe, and terminate in the bulb. Olfactory receptor neurones are continually replaced throughout life by differentiation of stem cells in the olfactory mucosa. The olfactory bulb is continuous posteriorly with the olfactory tract, through which the output of the bulb passes directly to the olfactory cortex.
There is a clear laminar structure in the olfactory bulb (Fig. 23.14). From the surface inwards the laminae are the olfactory nerve layer, glomerular layer, external plexiform layer, mitral cell layer, internal plexiform layer and granule cell layer. The olfactory nerve layer consists of the unmyelinated axons of the olfactory neurones. The continuous turnover of receptor cells means that axons in this layer are at different stages of growth, maturity or degeneration. The glomerular layer consists of a thin sheet of glomeruli where the incoming olfactory axons divide and synapse on terminal dendrites of secondary olfactory neurones, i.e. mitral, tufted and periglomerular cells. The external plexiform layer contains the principal and secondary dendrites of mitral and tufted cells. The mitral cell layer is a thin sheet composed of the cell bodies of mitral cells, each of which sends a single principal dendrite to a glomerulus, secondary dendrites to the external plexiform layer, and a single axon to the olfactory tract. It also contains a few granule cell bodies. The internal plexiform layer contains axons, recurrent and deep collaterals of mitral and tufted cells, and granule cell bodies. The granule cell layer contains the majority of the granule cells and their superficial and deep processes, together with numerous centripetal and centrifugal nerve fibres which pass through the layer.
The main types of interneurones in the olfactory bulb are the periglomerular cells and granule cells. The majority of periglomerular cells are dopaminergic (cell group A15), but some are GABAergic: their axons are distributed laterally and terminate within extraglomerular regions. Granule cells are similar in size to periglomerular cells. Their most characteristic feature is the absence of an axon, and they therefore resemble amacrine cells in the retina. Granule cells have two principal spine-bearing dendrites which pass radially in the bulb: they appear to be GABAergic. The granule cell is likely to be a powerful inhibitory influence on the output neurones of the olfactory bulb.
Hippocampal formation
The hippocampus lies above the subiculum and medial parahippocampal gyrus, forming a curved elevation, approximately 5 cm long, along the floor of the inferior horn of the lateral ventricle (Fig. 23.15). Its anterior end is expanded, and here its margin may present two or three shallow grooves that give a paw-like appearance, the pes hippocampi. The ventricular aspect is convex. It is covered by ependyma, beneath which fibres of the alveus converge medially on a longitudinal bundle of fibres, the fimbria of the fornix. Passing medially from the collateral sulcus, the neocortex of the parahippocampal gyrus merges with the transitional juxtallocortex of the subiculum (Fig. 23.16). The latter curves superomedially to the inferior surface of the dentate gyrus, then laterally to the laminae of the hippocampus. This curvature continues, first superiorly, then medially above the dentate gyrus, and ends pointing towards the centre of the superior surface of the dentate gyrus. The dentate gyrus is a crenated strip of cortex related inferiorly to the subiculum, laterally to the hippocampus and, more medially, to the fimbria of the fornix (Fig. 23.16, Fig. 23.17). The form of the fimbria is quite variable, but medially it is separated from the crenated medial margin of the dentate gyrus by the fimbriodentate sulcus (Fig. 23.18). The hippocampal sulcus, of variable depth, lies between the dentate gyrus and the subicular extension of the parahippocampal gyrus. Posteriorly, the dentate gyrus is continuous with the gyrus fasciolaris and thus with the indusium griseum. Anteriorly, it is continued into the notch of the uncus, turning medially across its inferior surface, as the tail of the dentate gyrus (band of Giacomini), and vanishes on the medial aspect of the uncus (Fig. 23.17). The tail separates the inferior surface of the uncus into an anterior uncinate gyrus and posterior intralimbic gyrus.
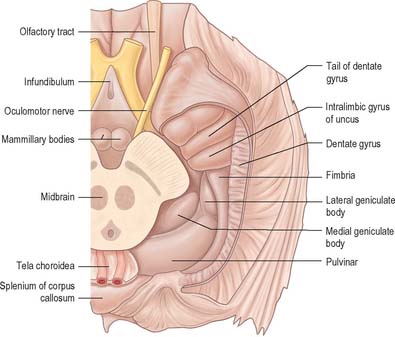
Fig. 23.17 Basal aspect of the brain dissected to display the dentate gyrus, uncus and fimbria on the left.
The trilaminar cortex of the dentate gyrus is the least complex of the hippocampal fields (Fig. 23.19). Its major cell type is the granule cell, found in the dense granule cell layer. Granule cells have unipolar dendrites that extend into the overlying molecular layer. The latter receives most of the afferent projections to the dentate gyrus, primarily from the entorhinal cortex (Fig. 23.20). The granule cell and molecular layers are sometimes referred to as the fascia dentata. The polymorphic layer, or hilus of the dentate gyrus, contains cells that give rise primarily to ipsilateral association fibres. They remain within the dentate gyrus and do not extend into other hippocampal fields.
The hippocampus is trilaminar archicortex. It consists of a single pyramidal cell layer, with plexiform layers above and below it. It may best be divided into three distinct fields, CA1, CA2 and CA3 (Fig. 23.18, Fig. 23.19). Field CA3 borders the hilus of the dentate gyrus at one end, and field CA2 at the other. Field CA3 pyramidal cells are the largest in the hippocampus and receive the mossy fibre input from dentate granule cells on their proximal dendrites. The whole pyramidal cell layer in this field is about 10 cells thick. The border between CA3 and CA2 is not well marked. The CA2 field has the most compact layer of pyramidal cells. It completely lacks a mossy fibre input from dentate granule cells and receives a major input from the supramammillary region of the hypothalamus. Field CA1 is usually described as the most complex of the hippocampal subdivisions and its appearance varies along its transverse and rostrocaudal axes. The CA1/CA2 border is not sharp, and at its other end CA1 overlaps the subiculum for some distance. Approximately 10% of neurones in this field are interneurones.
It is common to describe several strata within the layers of the hippocampus. Starting from the ventricular aspect, these are the alveus (contains subicular and hippocampal pyramidal cell axons converging on the fimbria of the fornix); stratum oriens (mainly the basal dendrites of pyramidal cells and some interneurones); stratum pyramidalis; stratum lucidum (contains mossy fibres which make contact with the proximal dendrites of pyramidal cells in field CA3); and the stratum radiatum and stratum lacunosum-moleculare. The stratum lucidum is not as prominent in man as it is in other primates, and is not present in fields CA1 and CA2.
The entorhinal cortex (Brodmann’s area 28, Fig. 23.19) extends rostrally to the anterior limit of the amygdala. Caudally it overlaps a portion of the hippocampal fields. The more primitive levels of the entorhinal cortex (below the amygdala) receive projections from the olfactory bulb. More caudal regions do not generally receive primary olfactory inputs. The entorhinal cortex is divisible into six layers and is quite distinct from other neocortical regions. Layer I is acellular and plexiform. Layer II is a narrow cellular layer, which consists of islands of large pyramidal and stellate cells. These cell islands are a distinguishing feature of the entorhinal cortex. They form small bumps on the surface of the brain that can be seen by the naked eye (verrucae hippocampae), and provide an indication of the boundaries of the entorhinal cortex. Layer III consists of medium-sized pyramidal cells. There is no internal granular layer (another classic feature of entorhinal cortex); in its place is an acellular region of dense fibres called the lamina dissecans, which is sometimes called layer IV. Layers III and V are apposed in regions where the lamina dissecans is absent. Layer V consists of large pyramidal cells five or six deep. Layer VI is only readily distinguishable from layer V close to the border with the perirhinal cortex. Its cells continue around the angular bundle (subcortical white matter deep to the subicular complex made up largely of perforant path axons) to lie beneath the pre- and parasubiculum.
There are many peptide-containing neurones in the hippocampal formation. Granule cells in the dentate gyrus appear to contain the opioid peptide dynorphin, which is also present in mossy fibres running to the CA3 field. Enkephalin, or a related peptide, may be present in fibres arising in the entorhinal cortex. There is a dense plexus of somatostatin-immunoreactive fibres in the molecular layer of the dentate gyrus and also in the stratum lacunosum-moleculare of the hippocampus. The polymorphic layer of the dentate gyrus, stratum oriens of the hippocampus and the deep layers of the entorhinal cortex, all contain somatostatin-immunoreactive neurones. Vasoactive intestinal polypeptide (VIP)-immunoreactive neurones are plentiful in many hippocampal fields, especially in the superficial layers of the entorhinal cortex. Cells containing cholecystokinin (CCK)-immunoreactivity are found in the hilar region of the dentate gyrus, in all layers of the hippocampus, especially in the pyramidal cell layer, and also throughout the subicular complex and entorhinal cortex. There are also substantial plexuses of CCK-immunoreactive fibres in the stratum lacunosum-moleculare, subicular complex and entorhinal cortex. Hippocampal CCK-immunoreactive cells may give rise to extrinsic projections, e.g. to the lateral septum and medial mammillary nucleus, because CCK-immunoreactive fibres are found in the fimbria/fornix.
Septum
The septum is a midline and paramedian structure (Fig. 23.13, Fig. 22.6). Its upper portion corresponds largely to the bilateral laminae of fibres, sparse grey matter and neuroglia, known as the septum pellucidum, which separates the lateral ventricles. Below this, the septal region is made up of four main nuclear groups: dorsal, ventral, medial and caudal. The dorsal group is essentially the dorsal septal nucleus, the ventral group consists of the lateral septal nucleus, the medial group contains the medial septal nucleus and the nucleus of the diagonal band of Broca, and the caudal group contains the fimbrial and triangular septal nuclei.
Projections from the lateral septum run to the medial and lateral preoptic areas, anterior hypothalamus, supramammillary and midbrain ventral tegmental area, via the medial forebrain bundle. There is also a projection to the medial habenular nucleus and some midline thalamic nuclei via the stria medullaris thalami, which runs on the dorsomedial wall of the third ventricle. The projections from the habenula via the fasciculus retroflexus to the interpeduncular nucleus and adjacent ventral tegmental area in the midbrain provide a route through which forebrain limbic structures can influence midbrain nuclear groups.
Amygdala
The amygdala (amygdaloid nuclear complex) consists of lateral, central and basal nuclei that lie in the dorsomedial temporal pole, anterior to the hippocampus, close to the tail of the caudate nucleus (Fig. 23.13) and partly deep to the gyrus semilunaris, gyrus ambiens and uncinate gyrus (Fig. 23.4). Collectively the nuclei form the ventral, superior and medial walls of the tip of the inferior horn of the lateral ventricle. The amygdala is partly continuous above with the inferomedial margin of the claustrum. Fibres of the external capsule and substriatal grey matter, including the cholinergic magnocellular nucleus basalis (of Meynert), incompletely separate it from the putamen and globus pallidus. Laterally, it is close to the optic tract.
The central nucleus is present through the caudal half of the amygdaloid complex. It lies dorsomedial to the basal nucleus and is divided into medial and lateral parts. The medial part, which contains larger cells than the lateral part, resembles the adjacent putamen. The medial and central nuclei appear to have an extension across the basal forebrain, as well as within the stria terminalis, which merges with the bed nucleus of the stria terminalis. This extensive nuclear complex is sometimes referred to as the ‘extended amygdala’ (Fig. 23.21). It can be considered as a macrostructure formed by the centromedial amygdaloid complex (medial nucleus, medial and lateral parts of the central nucleus), the medial bed nucleus of the stria terminalis, and the cell columns that traverse the sublenticular substantia innominata, which lies between them. It has been suggested that portions of the medial nucleus accumbens may be included in the extended amygdala.
Afferent connections
The anterior temporal lobe provides the largest proportion of the cortical input to the amygdala, predominantly to the lateral nucleus. Rostral parts of the superior temporal gyrus, which may represent unimodal auditory association cortex, project to the lateral nucleus. There are also projections from polymodal sensory association cortices of the temporal lobe, including perirhinal cortex (areas 35 and 36), the caudal half of the parahippocampal gyrus, the dorsal bank of the superior temporal sulcus, and both the medial and lateral areas of the cortex of the temporal pole.
WHITE MATTER OF CEREBRAL HEMISPHERE
ASSOCIATION FIBRES
Association fibres (Fig. 23.22, Fig. 23.23) may be either short association (arcuate or ‘U’) fibres, which link adjacent gyri, or long association fibres, which connect more widely separated gyri.
COMMISSURAL FIBRES
Corpus callosum
The corpus callosum (Fig. 23.2, Fig. 23.24, Fig. 23.25; see Fig. 22.2) is the largest fibre pathway of the brain. It links the cerebral cortex of the two cerebral hemispheres, and it roofs much of the lateral ventricles. It forms an arch approximately 10 cm long, with an anterior end approximately 4 cm from the frontal poles and a posterior end approximately 6 cm from the occipital poles. Its anterior portion is known as the genu. This recurves posteroinferiorly in front of the septum pellucidum, then diminishes rapidly in thickness and is prolonged to the upper end of the lamina terminalis as the rostrum. The trunk of the corpus callosum arches back and is convex above. It ends posteriorly in the expanded splenium, which is its thickest part.
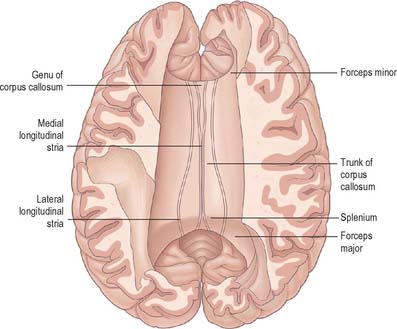
Fig. 23.24 The superior aspect of the corpus callosum revealed by partial removal of the cerebral hemispheres.
The superior surface of the callosal trunk (Fig. 23.24) is covered by a thin layer of grey matter, the indusium griseum. This extends anteriorly around the genu and then continues into the paraterminal gyrus on the inferior aspect of the rostrum. It contains narrow longitudinal bundles of fibres on each side, the medial and lateral longitudinal striae. Posteriorly, the indusium griseum is continuous with the dentate gyrus and hippocampus through the gyrus fasciolaris (Fig. 23.25).
Connections that link the same, or similar, areas on each side are termed homotopic connections. The corpus callosum also interconnects heterogeneous cortical areas on the two sides (heterotopic connections). These may serve to connect functionally similar, but anatomically different, loci in the two hemispheres, and/or to connect functional areas in one hemisphere with regions that are specialized for a unilaterally confined function in the other.
Anterior commissure
The anterior commissure is a compact bundle of myelinated nerve fibres which crosses anterior to the columns of the fornix and is embedded in the lamina terminalis, where it is part of the anterior wall of the third ventricle (Fig. 23.13). In sagittal section it is oval, its long (vertical) diameter is approximately 1.5 mm. Laterally it splits into anterior and posterior bundles. The smaller anterior bundle curves forwards on each side to the anterior perforated substance and olfactory tract. The posterior bundle curves posterolaterally on each side in a deep groove on the anteroinferior aspect of the lentiform complex, and subsequently fans out into the anterior part of the temporal lobe, including the parahippocampal gyrus. Areas thought to be connected via commissural fibres include: the olfactory bulb and anterior olfactory nucleus; the anterior perforated substance, olfactory tubercle and diagonal band of Broca; the prepiriform cortex; the entorhinal area and adjacent parts of the parahippocampal gyrus; part of the amygdaloid complex (especially the nucleus of the lateral olfactory stria); the bed nucleus of the stria terminalis and the nucleus accumbens; the anterior regions of the middle and inferior temporal gyri.
PROJECTION FIBRES
Projection fibres connect the cerebral cortex with lower levels in the brain and spinal cord. They include large numbers of both corticofugal and corticopetal projections. Corticofugal projection fibres converge from all directions to form the dense subcortical white matter mass of the corona radiata (Fig. 23.26). Large numbers of fibres pass to the corpus striatum and the thalamus, intersecting commissural fibres of the corpus callosum en route. The corona radiata is continuous with the internal capsule, which contains the majority of the cortical projection fibres.
Internal capsule
In horizontal cerebral sections the internal capsule appears as a broad white band, with a lateral concavity, which accommodates the lentiform complex (Fig. 23.27, Fig. 23.28, Fig. 23.29). It has an anterior limb, genu, posterior limb, and retrolenticular (retrolentiform) and sublenticular (sublentiform) parts. Both anterior and posterior limbs are medial to the lentiform complex. The head of the caudate nucleus is medial to the anterior limb, and the thalamus is medial to the posterior limb. Cortical efferent fibres of the internal capsule continue to converge as they descend. Fibres derived from the frontal lobe tend to pass posteromedially, while temporal and occipital fibres pass anterolaterally. Many, but not all, corticofugal fibres pass into the crus cerebri of the ventral midbrain. Here, corticospinal and corticobulbar fibres are located in the middle half of the crus. Frontopontine fibres are located medially, whereas corticopontine fibres from temporal, parietal and occipital cortices are found laterally.
The sublenticular part of the internal capsule contains temporopontine and some parietopontine fibres, the auditory radiation from the medial geniculate body to the superior temporal and transverse temporal gyri (areas 41 and 42), and a few fibres that connect the thalamus with the temporal lobe and insula. Fibres of the auditory radiation sweep anterolaterally below and behind the lentiform complex to reach the cortex.
NEUROPSYCHOLOGICAL FUNCTIONS
The neuropsychological functions of perception, spatial analysis, learned skilled movement, language, memory, problem solving (all executive functions) and emotion, are organized within the cerebral hemispheres (Fig. 23.30).
Patients with chronic epilepsy, who have undergone surgical section of the corpus callosum in order to relieve their seizures, portray few difficulties under normal circumstances. However, when these ‘split brain’ patients undergo psychological testing, the two halves of the brain appear to behave relatively autonomously, e.g. visual information directed to the right cerebral hemisphere alone does not evoke a verbal response, and consequently individuals cannot name objects or read words solely presented to the left visual field. Destruction of the splenium of the corpus callosum and its connections to the left occipital cortex, either by stroke or tumour, leads to the posterior disconnection syndrome of ‘alexia without agraphia’. Such individuals speak and write without difficulty but cannot understand written material (alexia). Disconnection of visual processes in the right hemisphere from the verbal processes of the dominant left cerebral hemisphere is thought to explain the syndrome.
Alheid GF, Heimer L. New perspectives in basal forebrain organization of special relevance for neuropsychiatric disorders: the striatopallidal, amygdaloid and corticopetal components of the substantia innominata. Neuroscience. 1988;27:1-39.
Derbyshire SW, Jones AK, Gyulai F, Clark S, Townsend D, Firestone LL. Pain processing during three levels of noxious stimulation produces differential patterns of central activity. Pain. 1997;73:431-445.
Papez JW. A proposed mechanism of emotion. Arch Neurol Psychiat. 1937;38:725-743.
The classic description of the mechanism of emotion in man..
Passingham RE. The Frontal Lobes and Voluntary Action. Oxford: Oxford University Press, 1993.
Penfield W, Rasmussen T. The Cerebral Cortex of Man. New York: Macmillan, 1950.
Peyron R, Laurent B, Garcia-Larrea L. Functional imaging of brain responses to pain. A review and meta-analysis. Neurophysiol Clin. 2000;30:263-288.
Sperry RW. Lateral specialization in the surgically separated hemisphere. In: Schmidt FO, Worden FG. The Neurosciences. Third study program. Cambridge, Mass: MIT Press; 1974:5-19.
Sperry RW. Consciousness, personal identity and the divided brain. Neuropsychologia. 1984;17:153-166.
Toga AW, Thompson PM, Mori S, Amunts K, Zilles K. Towards multimodal atlases of the human brain. Nature Rev Neurosci. 2006;7:952-966.