CHAPTER 23 Cerebral hemisphere
The two cerebral hemispheres are the largest major divisions of the brain. Each hemisphere consists of an external highly-convoluted cortex, beneath which lies an extensive internal mass of white matter that partly encloses the basal ganglia. Each hemisphere also contains a lateral ventricle that is continuous with the third ventricle through the interventricular foramen. The two hemispheres are linked by the commissural fibres of the corpus callosum.
The cerebral hemispheres are separated by a deep median cleft, the great longitudinal fissure, which contains a crescent-shaped fold of the dura mater, the falx cerebri (see Ch. 27).
GYRI, SULCI AND LOBES
The surface of the cerebral hemisphere shows a complex pattern of convolutions, or gyri, which are separated by furrows of varying depth known as fissures, or sulci (Fig. 23.1, Fig. 23.2). Some of these are consistently located, others less so. They provide part of the basis for the division of the hemisphere into lobes. The frontal, parietal, temporal and occipital lobes approximately correspond in surface extent to the overlying cranial bones from which they take their names. The anterior and posterior extremities of the hemispheres form the frontal and occipital poles respectively, and the temporal pole is the anterior extremity of the temporal lobe.
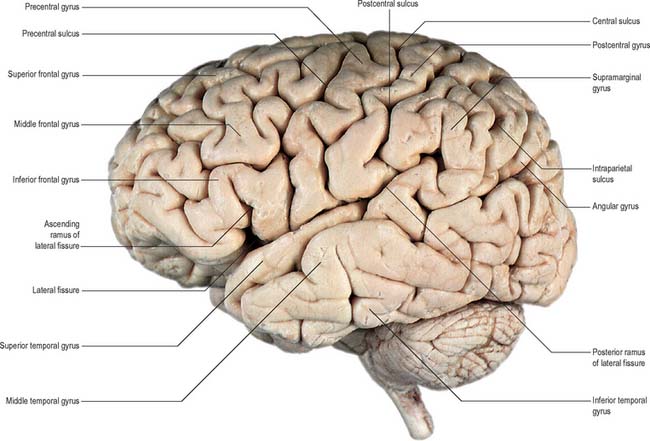
Fig. 23.1 Lateral aspect of the left cerebral hemisphere indicating the major gyri and sulci.
(Dissection by EL Rees; photograph by Kevin Fitzpatrick on behalf of GKT School of Medicine, London; figure enhanced by B. Crossman.)
The posterior region of the medial surface is traversed by the parieto-occipital and the calcarine sulci. These two deep sulci converge anteriorly to meet a little posterior to the splenium. The parieto-occipital sulcus marks the boundary between parietal and occipital lobes. It starts on the superomedial margin of the hemisphere approximately 5 cm anterior to the occipital pole, sloping down and slightly forwards to the calcarine sulcus. The calcarine sulcus starts near the occipital pole. Though usually restricted to the medial surface, its posterior end may reach the lateral surface. Directed anteriorly, it joins the parieto-occipital sulcus at an acute angle behind the splenium. Continuing forwards, it crosses the inferomedial margin of the hemisphere, and forms the inferior boundary of the isthmus, which connects the cingulate with the parahippocampal gyrus. The visual cortex lies above and below the posterior part of the calcarine sulcus, behind the junction with the parieto-occipital. The calcarine sulcus is deep and produces an elevation, the calcar avis, in the wall of the posterior horn of the lateral ventricle.
The inferior cerebral surface is divided by the stem of the lateral fissure into a small anterior and larger posterior part (Fig. 23.3, Fig. 23.4). The anterior part constitutes the orbital region of the frontal lobe. It is concave and lies above the cribriform plate of the ethmoid, the orbital plate of the frontal, and the lesser wing of the sphenoid bone. A rostrocaudal olfactory sulcus traverses it near its medial margin, overlapped by the olfactory bulb and tract. The medial strip thus demarked is the gyrus rectus. The rest of this surface bears irregular orbital sulci, generally H-shaped, which divide it into the anterior, medial, posterior and lateral orbital gyri.
The larger, posterior region of the inferior cerebral surface is partly superior to the tentorium but also to the middle cranial fossa. The collateral sulcus starts near the occipital pole, and extends anteriorly and parallel to the calcarine sulcus, separated from it by the lingual gyrus. The lingual gyrus, between the calcarine and collateral sulci, passes into the parahippocampal gyrus, which begins at the isthmus where it is continuous with the cingulate gyrus. Anteriorly, the parahippocampal gyrus continues into the hook-shaped uncus, which lies lateral to the midbrain and posterolateral to the anterior perforated substance. The uncus is part of the piriform cortex of the olfactory system, phylogenetically one of the oldest parts of the cortex, and is separated from the temporal pole by the rhinal sulcus (fissure) which marks the lateral limit of the entorhinal cortex (area) (Fig. 23.4).
CEREBRAL CORTEX
MICROSTRUCTURE
The microscopic structure of the cerebral cortex is an intricate blend of nerve cells and fibres, neuroglia and blood vessels. The neocortex essentially consists of three neuronal cell types. Pyramidal cells are the most abundant. Non-pyramidal cells, also called stellate or granule cells are divided into spiny and non-spiny neurones. All types have been subdivided on the basis of size and shape (Fig. 23.5).
Spiny stellate cells are the second most numerous cell type in the neocortex and for the most part occupy lamina IV. They have relatively small multipolar cell bodies, commonly 6 to 10 μm in diameter. Several primary dendrites, profusely covered in spines, radiate for variable distances from the cell body. Their axons ramify within the grey matter predominantly in the vertical plane. Spiny stellate cells probably use glutamate as their neurotransmitter.
Laminar organization
Typical neocortex is described as having six layers or laminae lying parallel to the surface (Fig. 23.6).
I The molecular or plexiform layer is cell sparse, containing only scattered horizontal cells and their processes enmeshed in a compacted mass of tangential, principally horizontal axons and dendrites. These are afferent fibres, which arise from outside the cortical area, together with intrinsic fibres from cortical interneurones, and the apical dendritic arbors of virtually all pyramidal neurones of the cerebral cortex. In histological sections stained to show myelin, layer I appears as a narrow horizontal band of fibres.
II The external granular lamina contains a varying density of small neuronal cell bodies including both small pyramidal and non-pyramidal cells; the latter may predominate. Myelin fibre stains show mainly vertically arranged processes traversing the layer.
III The external pyramidal lamina contains pyramidal cells of varying sizes, together with scattered non-pyramidal neurones. The size of the pyramidal cells is smallest in the most superficial part of the layer and greatest in the deepest part. This lamina is frequently further subdivided into IIIa, IIIb and IIIc, with IIIa most superficial and IIIc deepest. As in layer II, myelin stains reveal a mostly vertical organization of fibres.
IV The internal granular lamina is usually the narrowest of the cellular laminae. It contains densely packed, small, round cell bodies of non-pyramidal cells, notably spiny-stellate cells and some small pyramidal cells. Within the lamina, in myelin stained sections, a prominent band of horizontal fibres (outer band of Baillarger) is seen.
V The internal pyramidal (ganglionic) lamina typically contains the largest pyramidal cells in any cortical area, though actual sizes vary considerably from area to area. Scattered non-pyramidal cells are also present. In myelin stains, the lamina is traversed by ascending and descending vertical fibres, and also contains a prominent central band of horizontal fibres (inner band of Baillarger).
VI The multiform (or fusiform/pleiomorphic) layer consists of neurones with a variety of shapes, including recognizable pyramidal, spindle, ovoid and many other shaped somata. Typically, most cells are small to medium in size. This lamina blends gradually with the underlying white matter, and a clear demarcation of its deeper boundary is not always possible.
Five regional variations are described in neocortical structure (Fig. 23.7). While all are said to develop from the same six-layered pattern, two types, granular and agranular, are regarded as virtually lacking certain laminae, and are referred to as heterotypical. Homotypical variants, in which all six laminae are found, are called frontal, parietal and polar, names that link them with specific cortical regions in a somewhat misleading manner (e.g. the frontal type also occurs in parietal and temporal lobes).
It is customary to refer to some discrete cortical territories not only by their anatomical location in relation to gyri and sulci, but also in relation to their cytoarchitectonic characteristics (Brodmann’s areas) (Fig. 23.8). Some of the areas so defined, e.g. the primary sensory and motor cortices, have clear relevance in terms of anatomical connections and functional significance, others less so.
OVERVIEW OF CORTICAL CONNECTIVITY
The primary somatosensory, visual and auditory areas give rise to ipsilateral corticocortical connections to the association areas of the parietal, occipital and temporal lobes, respectively, which then progressively project towards the medial temporal limbic areas, notably the parahippocampal gyrus, entorhinal cortex and hippocampus. Thus, the first (primary) somatic sensory area (SI) projects to the superior parietal cortex (Brodmann’s area 5), which in turn projects to the inferior parietal cortex (area 7). From here connections pass to cortex in the walls of the superior temporal sulcus, and so on to the posterior parahippocampal gyrus, and on into limbic cortex. Similarly, for the visual system, the primary visual cortex (area 17) projects to the parastriate cortex (area 18), which in turn projects to the peristriate region (area 19). Information then flows to inferotemporal cortex (area 20), to cortex in the walls of the superior temporal sulcus, then to medial temporal cortex in the posterior parahippocampal gyrus, and so to limbic areas. The auditory system shows a similar progression from primary auditory cortex to temporal association cortex and so to the medial temporal lobe.
FRONTAL LOBE
The frontal lobe is the rostral region of the hemisphere, anterior to the central sulcus and above the lateral fissure. The precentral gyrus runs parallel to the central sulcus on the superolateral surface and extends onto the medial surface, and is limited anteriorly by the precentral sulcus. The area of the frontal lobe anterior to the precentral sulcus is divided into the superior, middle and inferior frontal gyri (Fig. 23.1). The frontal pole lies in front of these gyri. The ventral surface of the frontal lobe, the orbitofrontal cortex, overlies the bony orbit. The medial surface extends from the frontal pole to the paracentral lobule and consists of the medial frontal cortex and the anterior cingulate cortex.
Primary motor cortex
The primary motor cortex (MI) corresponds to the precentral gyrus (area 4), and is the area of cortex with the lowest threshold for eliciting contralateral muscle contraction by electrical stimulation. It contains a detailed topographically organized map (motor homunculus) of the opposite body half, with the head represented most laterally, and the leg and foot represented on the medial surface of the hemisphere in the paracentral lobule (Fig. 23.9). A striking feature is the disproportionate representation of body parts in relation to their physical size: large areas represent the muscles of the face and hand, which are capable of finely controlled or fractionated movements.
Premotor cortex
Immediately in front of the primary motor cortex lies Brodmann’s area 6. It extends onto the medial surface, where it becomes contiguous with area 24 in the cingulate gyrus, anterior and inferior to the paracentral lobule. A number of functional motor areas are contained within this cortical region. Lateral area 6, the area over most of the lateral surface of the hemisphere, corresponds to the premotor cortex.
Frontal eye field
The frontal eye field corresponds to parts of Brodmann’s areas 6, 8 and 9 (Fig. 23.10). It receives its major thalamic projection from the parvocellular mediodorsal nucleus, with additional afferents from the medial pulvinar, the ventral anterior nucleus and the suprageniculate–limitans complex, and connects with the paracentral nucleus of the intralaminar group. The thalamocortical pathways to the frontal eye field form part of a pathway from the superior colliculus, the substantia nigra and the dentate nucleus of the cerebellum. The frontal eye field has extensive ipsilateral corticocortical connections, receiving fibres from several visual areas in the occipital, parietal and temporal lobes, including the medial temporal area (V5) and area 7a. There is also a projection from the superior temporal gyrus, which is auditory rather than visual in function. From within the frontal lobe, the frontal eye field receives fibres from the ventrolateral and dorsolateral prefrontal cortices. It projects to the dorsal and ventral premotor cortices and to the medial motor area, probably to the supplementary eye field adjacent to the supplementary motor area proper. It projects prominently to the superior colliculus, to the pontine gaze centre within the pontine reticular formation, and to other oculomotor related nuclei in the brain stem. As its name implies, it is important in the control of eye movements.
Supplementary motor cortex
Stimulation of the supplementary motor area in conscious patients has been reported to elicit the sensation of an urge to move, or of anticipation that a movement is about to occur. A region anterior to the supplementary motor area for face representation (areas 44, 45) is important in vocalization and speech production (Fig. 23.10).
Prefrontal cortex
The prefrontal cortex on the lateral surface of the hemisphere comprises predominantly Brodmann’s areas 9, 46 and 45 (Fig. 23.8). In non-human primates, two subdivisions of the lateral prefrontal cortex are recognized, a dorsal area equivalent to area 9, and perhaps including the superior part of area 46, and a ventral area, consisting of the inferior part of area 46 and area 45. Areas 44 and 45 are particularly notable in man since, in the dominant hemisphere, they constitute the motor speech area (Broca’s area). Both the dorsolateral and ventrolateral prefrontal areas receive their major thalamic afferents from the mediodorsal nucleus, and there are additional contributions from the medial pulvinar, the ventral anterior nucleus and from the paracentral nucleus of the anterior intralaminar group. The dorsolateral area receives long association fibres from the posterior and middle superior temporal gyrus (including auditory association areas), from parietal area 7a, and from much of the middle temporal cortex. From within the frontal lobe it also receives projections from the frontal pole (area 10), and from the medial prefrontal cortex (area 32) on the medial surface of the hemisphere. It projects to the supplementary motor area, the dorsal premotor cortex and the frontal eye field. All these thalamic and corticocortical connections are reciprocal. Commissural connections are with the homologous area, and with the contralateral inferior parietal cortex. The ventrolateral prefrontal area receives long association fibres from both area 7a and area 7b of the parietal lobe, from auditory association areas of the temporal operculum, from the insula and from the anterior part of the lower bank of the superior temporal sulcus. From within the frontal lobe it receives fibres from the anterior orbitofrontal cortex and projects to the frontal eye field and the ventral premotor cortex. It connects with the contralateral homologous area via the corpus callosum. These connections are probably all reciprocal.