Cells and Cellular Activities of the Immune System
Lymphocytes and Plasma Cells
At the conclusion of this chapter, the reader should be able to:
• Differentiate and compare the function of primary and secondary lymphoid tissues.
• Describe the structure and function of a lymph node.
• Explain the role of the thymus in T lymphocyte maturation.
• Describe the maturation of a B lymphocyte from origination to plasma cell development.
• Compare the function of T lymphocytes and B lymphocytes in immunity.
• Explain the function of natural killer (NK) cells.
• Define the term cluster of differentiation (CD) and explain the purpose of detecting this marker.
• Differentiate the characteristics of T lymphocyte subsets on the basis of antigen structures and function.
• Describe the evaluation of suspected lymphocytic or plasma cell defects.
• Name and compare disorders of immunologic (lymphocytic or plasma cell) origin.
• Compare various categories of immunodeficiency disorders.
• Analyze and apply knowledge from this chapter to a representative case study.
• Correctly answer case study–related multiple choice questions.
• Be prepared to participate in a discussion of critical thinking questions.
Lymphoid and Nonlymphoid Surface Membrane Markers
Before 1979, human lymphocytes could be classified as T or B cells based on observation of these cells with electron microscopy (Fig. 4-1). T lymphocytes have a relatively smooth surface compared with the rough pattern of the B lymphocytes.
The introduction of monoclonal antibody (MAb) testing (see Chapter 2) led to the present identification of surface membrane markers on lymphocytes and other cells. In practical terms, surface markers are used to identify and enumerate various lymphocyte subsets, establish lymphocyte maturity, classify leukemias, and monitor patients on immunosuppressive therapy.
In this system, a surface marker that identifies a particular lineage or differentiation stage with a defined structure, and that can be identified with a group or cluster of MAbs, is called a member of a cluster of differentiation (CD; Fig. 4-2). Markers can be categorized as follows:
• Some markers are specific for cells of a particular lineage or maturational pathway.
• Some markers vary in expression, depending on the state of activation or differentiation of the same cells—for example, when CD antigen identification is used to classify lymphocyte subsets (e.g., CD4 and CD8).
• Promotion of cell to cell interactions and adhesion
• Transduction of signals that lead to lymphocyte activation
Sites of Lymphocyte Development
In mammalian immunologic development, the precursors of lymphocytes arise from progenitor cells of the yolk sac and liver (Fig. 4-3). Later in fetal development, and throughout the life cycle, the bone marrow becomes the sole provider of undifferentiated progenitor cells, which can further develop into lymphoblasts. Continued cellular development and proliferation of lymphoid precursors occur as the cells travel to the primary and secondary lymphoid tissues.
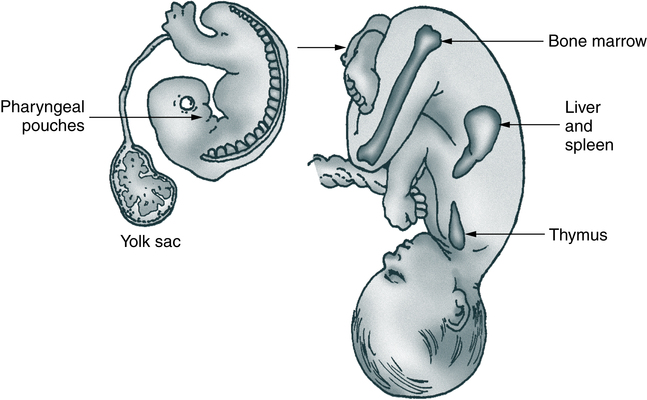
The anatomy of the human fetus illustrates the development of the mammalian immune system. Cells of the pharyngeal pouches migrate into the chest and form the thymus. Precursors of lymphocytes originate early in embryonic life in the yolk sac and eventually migrate to the bone marrow via the spleen and liver.
Primary Lymphoid Tissue
In mammals, both the bone marrow (and/or fetal liver) and thymus are classified as primary or central lymphoid organs (Fig. 4-4).
Thymus
Involution of the thymus is the first age-related change occurring in the human immune system. In postnatal life, the thymus is the primary organ that produces naïve T cells for the peripheral T cell pool but production of cells declines as early as 3 months of age. The thymus gradually loses up to 95% of its mass during the first 50 years of life (Fig. 4-5). The accompanying functional changes of decreased synthesis of thymic hormones and the loss of ability to differentiate immature lymphocytes are reflected in an increased number of immature lymphocytes within the thymus and as circulating peripheral blood T cells. Most changes in immune function, such as dysfunction of T and B lymphocytes, elevated levels of circulating immune complexes, increases in autoantibodies, and monoclonal gammopathies are correlated with involution of the thymus (see Chapter 27). Immune senescence may account for the increased susceptibility of older adults to infections, autoimmune disease, and neoplasms.
Secondary Lymphoid Organs
Secondary lymphoid organs provide a unique microenvironment for the initiation and development of immune responses. The secondary lymphoid tissues include lymph nodes, spleen, GALT, thoracic duct, bronchus-associated lymphoid tissue (BALT), skin-associated lymphoid tissue, and blood. Mature lymphocytes and accessory cells (e.g., antigen-presenting cells) are found throughout the body, although the relative percentages of T and B cells vary in different locations (Table 4-1).
Table 4-1
Approximate Percentage of Lymphocytes in Lymphoid Organs
Lymphoid Organ | T Lymphocytes (%) | B Lymphocytes (%) |
Thymus | 100 | 0 |
Blood | 80 | 20 |
Lymph nodes | 60 | 40 |
Spleen | 45 | 55 |
Bone marrow | 10 | 90 |
Adapted from Claman HN: The biology of the immune response, JAMA 268:2790–2796, 1992.
Tumor necrosis factor (TNF) and lymphotoxin are essential to the formation and maintenance of secondary organs. These cytokines are produced by B and T lymphocytes. Proliferation of the T and B lymphocytes in the secondary or peripheral lymphoid tissues (Fig. 4-6) is primarily dependent on antigenic stimulation.
The T lymphocytes or T cells populate the following:
1. Perifollicular and paracortical regions of the lymph nodes
2. Medullary cords of the lymph nodes
The B lymphocytes or B cells multiply and populate the following:
1. Follicular and medullary (germinal centers) of the lymph nodes
Lymph Nodes
Lymph nodes act as lymphoid filters in the lymphatic system. Lymph nodes respond to antigens introduced distally and routed to them by afferent lymphatics (Fig. 4-7). Generalized lymph node reactivity can occur after systemic antigen challenge (e.g., serum sickness).
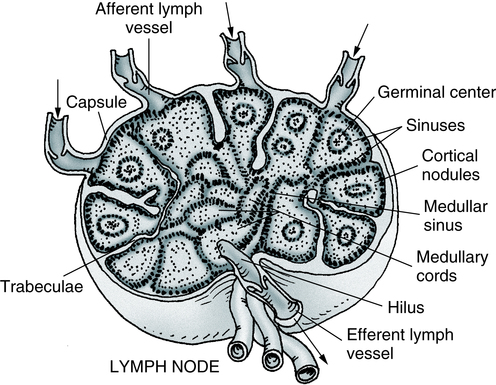
Several valved afferent lymphatics bring lymph to the node. An efferent lymphatic leaves the node at the hilus. Note that the artery and vein enter and leave at the hilus. (Adapted from Anthony CP, Thibodeau GA: Textbook of anatomy and physiology, ed 12, St Louis, 1987, Mosby.)
Circulation of Lymphocytes
Recirculation of lymphocytes back to the blood is through the major lymphatic ducts. Lymphocytes enter the lymph node from the blood circulation via arterioles and capillaries to reach the specialized postcapillary venules. From the venule, the lymphocytes enter the node and remain in the node or pass through the node and return to the circulating blood. Lymphatic fluid, lymphocytes, and antigens from certain body sites enter the lymph node through the afferent lymphatic duct and exit the lymph node through the efferent lymphatic duct (see Fig. 4-7).
Virgin or Naïve Lymphocytes
Memory cells are populations of long-lived T or B cells that have been stimulated by antigen. They can make a quick response to a previously encountered antigen. Memory B cells carry surface IgG as their antigen receptor; memory T cells express the CD45RO variant of the leukocyte common antigen and increased levels of cell-adhesion molecules (CAMs), chemical mediators involved in inflammatory processes throughout the body (Fig. 4-8).
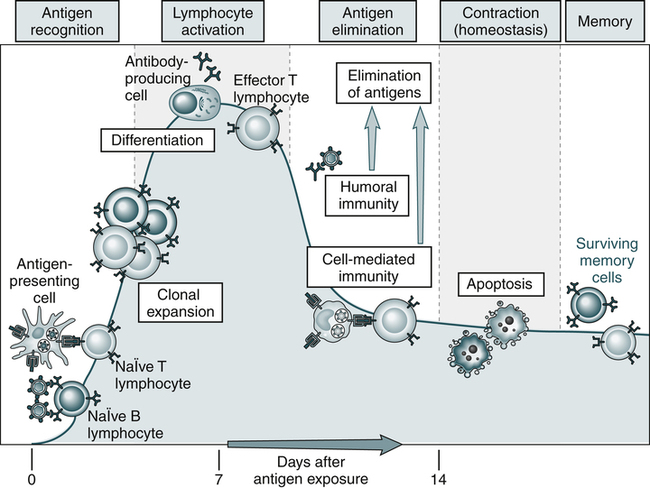
An adaptive immune response consists of distinct phases; the first three are the recognition of antigen, activation of lymphocytes, and elimination of antigen (effector phase). The response declines as antigen-stimulated lymphocytes die by apoptosis, restoring homeostasis, and the antigen-specific cells that survive are responsible for memory. The duration of each phase may vary in different immune responses. The y-axis represents an arbitrary measure of the magnitude of the response. These principles apply to humoral immunity (measured by B lymphocytes) and cell-mediated immunity (mediated by T lymphocytes). (From Abbas AK, Lichtman AH: Basic immunology: functions and disorders of the immune system, updated edition, ed 3, Philadelphia, 2011, Saunders.)
Development of T Lymphocytes
Most lymphocytes (see Color Plate 8) found in the circulating blood are T cells derived from bone marrow progenitor cells that mature in the thymus gland (Table 4-2). These cells are responsible for cellular immune responses and are involved in the regulation of antibody reactions in conjunction with B lymphocytes.
Table 4-2
Type | Function(s) | Phenotypic Marker | Peripheral Blood (% of Total) |
Helper T (Th) cells | Stimulate B cell growth and differentiation (humoral immunity); macrophage activation by secreted cytokines (cell-mediated immunity) | CD3+, CD4+, CD8− | 50-60 |
Cytotoxic T (Tc) cells | Lysis of virus-infected cells, tumor cells, and allografts (cell-mediated immunity); macrophage activation by secreted cytokines (cell-mediated immunity) | CD3+, CD4−, CD8+ | 20-25 |
Natural killer (NK) cells | Lysis of virus-infected cells, (antibody-dependent cellular cytotoxicity) | Fc receptor for IgG or cells CD16 | ∼10 |
B cells | Antibody production (humoral immunity) | Fc receptors, MHC class II, CD19, CD21 | 10-15 |
Early Cellular Differentiation and Development
Helper T Lymphocytes
• Helper T type 1 (Th1) cells are responsible for cell-mediated effector mechanisms.
• Helper T type 2 (Th2) cells play a greater role in the regulation of antibody production.
• Regulatory T (Treg) cells are an immunoregulatory type of Th cells.
These divisions are not absolute, with considerable overlap or redundancy in function among the different subsets. This classification is based on the in vitro blends of cytokines that they produce. Th1 and Th2 cells can promote the development of cytotoxic cells and are believed to develop from Th0 cells. Th1 cells interact most effectively with mononuclear phagocytes; Th2 cells release cytokines that are required for B cell differentiation (Fig. 4-9).
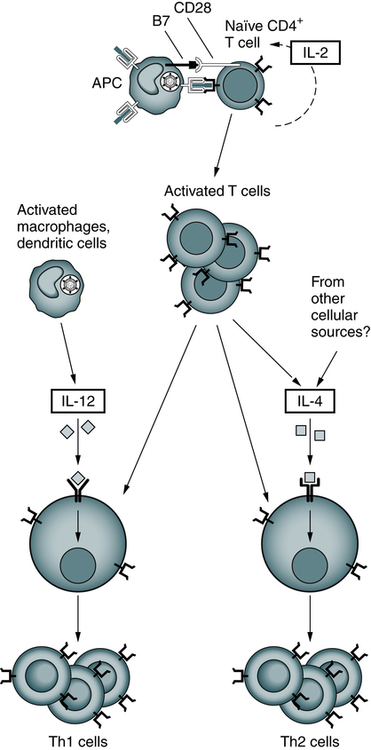
After their activation by antigen and costimulators, naïve helper T cells may differentiate into Th1 and Th2 cells under the influence of cytokines. IL-12 produced by microbe-activated macrophages and dendritic cells stimulates differentiation of CD4+ T cells into Th1 effectors. In the absence of IL-12, the T cells themselves (and perhaps other cells) produce IL-4, which stimulates their differentiation into Th2 effectors. (Adapted from Abbas AK, Lichtman AH: Basic immunology: functions and disorders of the immune system, ed 3, Philadelphia, 2008, Saunders.)
Characterized by high interferon-gamma (IFN-γ) production, Th1 responses promote the elimination of intracellular pathogens (Fig. 4-10, A). Characterized by IL-4 and IL-5, Th2 responses promote a different type of effector response that involves immunoglobulin E (IgE) production and eosinophils capable of eliminating larger extracellular pathogens, such as helminths (see Fig. 4-10, B). In situations of repeated pathogen exposure or persistent infection, the polarization of T cell responses serves to focus the antigen-specific response on a specific effector pathway.
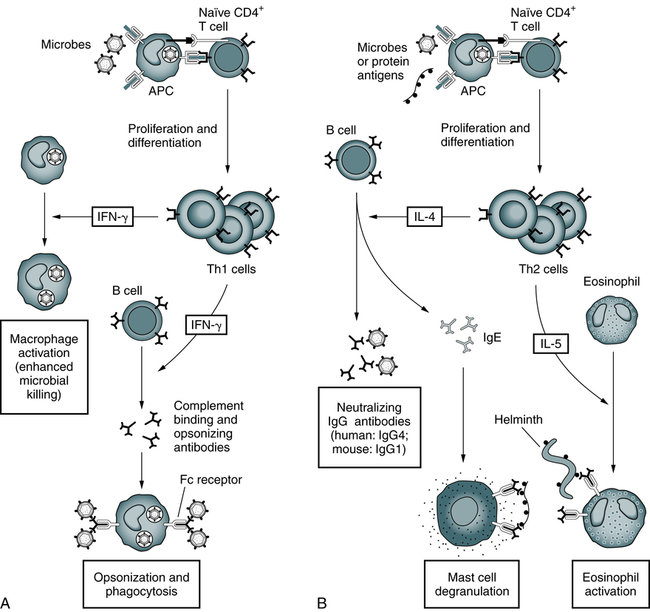
A, Th1 cells produce the cytokine IFN-γ, which activates phagocytes to kill ingested microbes and stimulates the production of antibodies that promote the ingestion of microbes by the phagocytes. B, Th2 cells specific for microbial or nonmicrobial protein antigens produce the cytokines IL-4, which stimulates the production of IgE antibody, and IL-5, which activates eosinophils. IgE participates in the activation of mast cells by protein antigens and coats helminths for destruction by eosinophils. Th2 cells also stimulate the production of other antibodies (IgG4 in humans) that neutralize microbes and toxins but do not bind to Fc receptors or activate complement efficiently. (Adapted from Abbas AK, Lichtman AH: Basic immunology: functions and disorders of the immune system, ed 3, Philadelphia, 2008, Saunders.)
The following factors can influence the terminal differentiation of lymphocytes:
Cytotoxic T Lymphocytes
Cytotoxic T lymphocytes, or T cytotoxic (Tc) cells, are effector cells found in the peripheral blood that are capable of directly destroying virally infected target cells. Most Tc cells are CD8+ and recognize antigen on the target cell surface associated with MHC class I molecules (e.g., human leukocyte antigen [HLA] types A, B, and C) or MHC class I alone. This process is demonstrated by the immune response to virus-infected cells or tumor cells (Fig. 4-11).
In addition to destruction of virally infected, MHC class I–bearing targets, Tc cells are major effectors in allograft organ rejection. Tc cells express CD4 or CD8, depending on the MHC antigen restriction that governs their antigen recognition (i.e., class I or II antigens; Fig. 4-12).
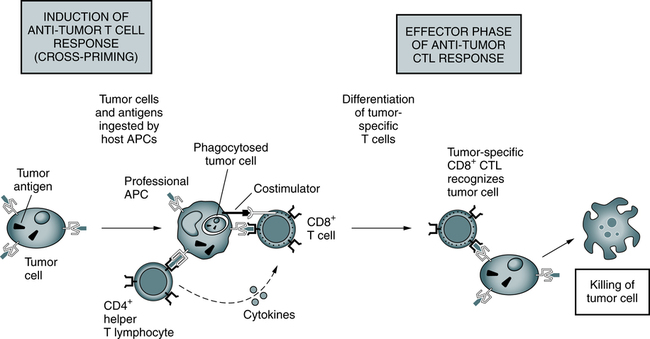
Responses to tumors may be induced by cross-priming (or cross-presentation), in which the tumor cells and tumor antigens are taken up by specialized (so-called professional) APCs, processed, and presented to T cells. In some cases, B7 costimulators expressed by the APCs provide the second signals for the differentiation of the CD8+ T cells. APCs may also stimulate CD4+ helper T cells, which provide the second signals for cytotoxic T lymphocyte (CTL) development. Differentiated CTLs kill tumor cells without a requirement for costimulation or T cell assistance. (From Abbas AK, Lichtman AH: Basic immunology: functions and disorders of the immune system, ed 3, Philadelphia, 2008, Saunders.)
Antigen Recognition by T Cells
The TCR of most T lymphocytes is composed of an alpha and beta polypeptide chain, with constant regions located close to the cell surface and the part that binds to the antigenic peptide of appropriate fit located away from the cell surface. The difference in structure of the distal regions of the alpha and beta chains allows the development of different clones of T cells. The TCR reacts with antigen in the context of MHC class I or II molecules on an APC (Fig. 4-13).
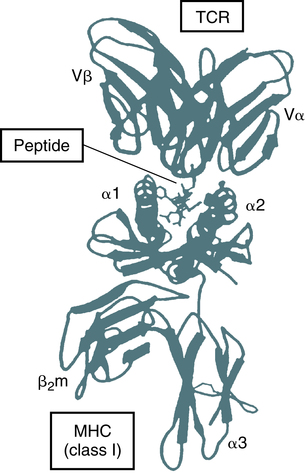