38 Care of the neurosurgical patient
Atony: Decreased or absent muscle tone.
Babinski Reflex: A reflex that is normal in newborns but abnormal in adults; in adults, it indicates a lesion in the pyramidal tract. The reflex is elicited with firm stroking of the lateral aspect of the sole of the foot, which normally elicits dorsiflexion of the big toe with extension and fanning of the other toes.
Baroreceptor: A sensory nerve cell aggregate present in the wall of a blood vessel that is stimulated by changes in blood pressure.
Compliance: The ability of the brain to yield when a pressure or force is applied.
Cranial Surgery: Surgery classified by infratentorial and supratentorial location.
Craniectomy: Removal of a portion of the skull without a replacement.
Cranioplasty: Repair of the skull with replacement of a part of the cranium with a synthetic material.
Craniotomy: A surgical opening of the skull.
Crepitus: A crackling sound produced by the rubbing together of fractured bone fragments or by the presence of subcutaneous emphysema.
Cushing Reflex: An elevated systolic blood pressure, bradycardia, and widening pulse pressure.
Decompensation: The inability of the heart to maintain adequate circulation because of an impairment in brain integrity.
Diabetes Insipidus: A metabolic disorder caused by injury or disease of the posterior lobe of the pituitary gland (the hypophysis).
Focal Deficit: Any sign or symptom that indicates a specific or localized area of pathologic alteration.
Infratentorial: The area below the tentorium that includes the brain stem, cerebellum, and posterior fossa. This approach is used for lesions in the brain stem and cerebellum region.
Laminectomy: Excision of the posterior arch of a vertebra to allow excision of a herniated nucleus pulposus.
Phrenic Nucleus: A group of nerve cells located in the spinal cord between the levels of C3 and C5. Damage to this area abolishes or alters the function of the phrenic nerve.
Pyramidal Signs: Symptoms of dysfunction of the pyramidal tract, including spastic paralysis, Babinski’s reflex, and increased deep tendon reflexes.
Queckenstedt Test: The veins of the neck are compressed on one or both sides. In a healthy person, the cerebrospinal fluid (CSF) pressure rises rapidly and then quickly returns to normal when the pressure is taken off the neck. In a patient with spinal cord obstruction, little or no increase in pressure is found. This test is diagnostically accurate for most cord compressions; however, false-negative results may be obtained if the lesion is located high in the cervical spine area. This test is not performed in patients with known or suspected increased intracranial pressure (ICP).
Rhizotomy: Surgical interruption of the roots of the spinal nerves within the spinal canal.
Spinal Shock: A state that occurs after a spinal cord injury. All sensory, motor, and autonomic activities are lost below the level of the transection, and reflexes are absent. Paralysis is of a flaccid nature and includes the urinary bladder. Autonomic activity gradually resumes as spinal shock subsides. When autonomic activity has returned, bladder and bowel training programs can be started. Flaccid paralysis may develop into varying degrees of spastic paralysis, as evidenced by spasms of flexor or extensor muscle groups. The presence of autonomic activity also allows for episodes of autonomic hyperreflexia.
Subarachnoid Block: The injection of a local anesthetic into the subarachnoid space around the spinal cord.
Subluxation: Partial or incomplete dislocation.
Supratentorial: The area above the tentorium that includes the cerebrum. The supratentorial approach is used for frontal, temporal, parietal, and occipital lobe lesions.
Tonoclonic Movements: Tense muscular contractions that alternate rapidly with muscular relaxation.
Valsalva Maneuver: Contraction of the thorax in forced expiration against the closed glottis; results in increases in intrathoracic and intraabdominal pressures.
As shown in Chapter 10, the physiology of the nervous system is extremely complex. Many neurologic care units have emerged because of the specific type of care needed in the perioperative period. Special education on the physiology, pharmacology, and nursing care is necessary to facilitate appropriate outcomes for the neurosurgical patient. Because nurses in the postanesthesia care unit (PACU) are able to render highly specialized nursing care to these patients, most facilities require that these patients first recover from anesthesia in the PACU before returning to the neurologic care unit or a routine care unit. Neurosurgical patients, or those with underlying neurologic conditions, present a challenge to the perianesthesia nurse. In addition to familiarity with routine perianesthesia care, the nurse must have a basic understanding of the nervous system and pathologic conditions or injuries that may affect this system and must be able to translate this knowledge into the skills necessary to assess, provide care for, and evaluate the neurosurgical patient. This chapter is divided into two sections: cranial surgery and spinal surgery. The division is made solely for this discussion because some aspects of care related to each topic are common to both areas. In addition, disease or injury in any portion of the nervous system may also affect other organs and systems of the body. In caring for the neurosurgical patient, the nurse must consider each structure of the nervous system (see Chapter 10) as it relates to the individual as a whole.
Cranial surgery
Diagnostic tools
Techniques used to ascertain the presence and extent of cranial injury or disease include invasive and noninvasive techniques. A brief discussion of invasive and noninvasive diagnostic procedures is included to familiarize the PACU nurse with the techniques and special considerations necessary in the care of these patients. Many interventional neuroradiology procedures with general anesthesia are now done; these patients go to the PACU after the procedure is completed. The specific PACU care is presented; if information on types of sedation (usually dexmedetomidine) for these patients is needed, please see Chapter 21.
Magnetic resonance imaging
The patient is placed within a cylindric high-powered magnet. Body tissues are then subjected to a magnetic field, which causes some of the hydrogen ions to align themselves with the field. A burst of low-energy radio waves is then applied to knock atomic protons within the tissues out of alignment. When the radio waves are discontinued, these protons release tiny amounts of energy that are “read” by a computer. Next, the MRI generates an image based on this information, thus yielding a detailed picture of the structural content and contours of the internal organs. Contraindications for MRI include claustrophobic, agitated, or obese patients and patients with metallic devices or fragments present in the body.
Positron emission tomography
Recent advances in technology have emerged combining the PET and CT scan together. The PET/CT imaging results in shorter imaging times than the PET scan alone. In addition, research indicates improved lesion localization in addition to more exact tumor staging.1
Cerebral angiography
Arteriography, or angiography, is the diagnostic tool for aneurysms, arteriovenous malformations, and other cerebrovascular abnormalities. A cannulated needle2 is introduced into the femoral or axillary artery and threaded to the level of the common carotid artery. Radiopaque dye is then injected, and radiographs record its path through the cerebral vasculature (Fig. 38-1). Irritation brought on by use of the dye may manifest itself in altered states of consciousness, hemiparesis, or speech difficulties that are usually transient. During and after arteriography, the patient may have an allergic reaction to the dye that can range from mild urticaria to anaphylaxis. Resuscitative equipment must be immediately available until the danger of allergic reaction has passed.
Postprocedure care includes proper hydration to prevent renal complications from the dye.3 In addition, the patient will require close neurologic and cardiovascular monitoring. The proceduralist may have used a closure device at the arterial site. Manufacturer recommendations, institutional policies and proceduralist orders should all be maintained. These orders will consist of bedrest, puncture site checks for bleeding or hematoma and vascular check of the affected limb.4 Intravenous fluids are maintained until the danger of untoward reaction has passed and the patient no longer has the transient nausea that occasionally occurs.
Injuries and pathologic conditions of the brain
Types of injuries
Skull fractures are categorized as linear, comminuted, depressed, or basilar. The linear skull fracture associated with mild brain injury4 and do not require treatment. A comminuted fracture, also known as the eggshell fracture, is a culmination of multiple linear fractures.5 A depressed skull fracture is an inward depression of the skull and is classified as open (compound) or simple (closed).5 Infection is a primary concern, and surgery may be necessary to remove bony fragments, clean the wound, and elevate the depressed bone. Basilar skull fractures occur in the base of the skull and are difficult to diagnose with radiographs. Diagnosis is confirmed with clinical data. Patients often have “raccoon’s eyes” (periorbital ecchymosis), Battle sign (ecchymosis around the mastoid process), or CSF otorrhea.
Consequences of injury
Traumatic head injury can cause hemorrhage beneath a skull fracture or from a shearing of the veins or cortical arteries and results in epidural, subdural, subarachnoid, or intraventricular hemorrhage (Fig. 38-2). The signs and symptoms of brain ischemia and increased intracranial pressure (ICP) vary with the speed at which the functions of vital centers are altered. A small clot that accumulates rapidly may be fatal; however, the patient may survive a slowly developing, much larger hematoma through effective compensatory mechanisms.
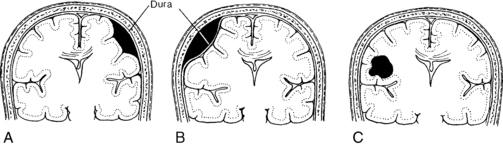
FIG. 38-2 Types of hematomas. A, Subdural hematoma. B, Epidural hematoma. C, Intracerebral hematoma.
(From Black JM, Hokanson Hawks JH: Medical-surgical nursing: clinical management for positive outcomes, ed 8, St. Louis, 2009, Saunders.)
Subacute subdural hematomas fail to show acute signs and symptoms at onset. Brain swelling is not great, but the hematoma may become large enough to produce symptoms. Progressive hemiparesis, obtundation, and aphasia often appear 2 to 14 days after injury. The degree of ultimate recovery depends on the extent of damage produced at the time of injury.
Intraventricular hematoma, which is usually caused by a subarachnoid or intracerebral hemorrhage, is bleeding into the ventricles.6 This can be caused by brain trauma such as penetrating wounds or from an anterior communicating and basilar tip aneurysm.5 An intraventricular hematoma is associated with high mortality, and treatment includes a ventriculostomy with CSF drainage and ICP management.6
Types of pathologic conditions
Cerebral aneurysms are round dilations of the arterial wall that develop as a result of weakness of the wall from defects in the media layer of the artery. Most cerebral aneurysms occur at bifurcations close to the circle of Willis and usually involve the anterior portion. Common bifurcations include those with the internal carotid, the middle cerebral, and the basilar arteries and in relation to the anterior and posterior communicating arteries. The exact cause or precipitating factor is not well defined but may be related to congenital abnormality, arteriosclerosis, embolus, or trauma. Aneurysms are usually asymptomatic and present no clinical problem to the patient unless rupture occurs, which results in neurologic deficits. Ruptured cerebral aneurysm is the major cause of subarachnoid hemorrhage or hemorrhagic stroke. Depending on the severity of the cerebral bleed, the rupture of a cerebral aneurysm can often be fatal.6 If treatable, surgical intervention usually involves clipping or coiling of the aneurysm after identification through angiography. Careful consideration is given to the complications that can occur after aneurismal rupture or bleeding, which are rebleeding, vasospasm, and hydrocephalus.6
Intracranial pressure dynamics
Volume may be added to any of the cerebral compartments and results in increased ICP when the compensatory capacity is exceeded. Brain volume can be increased by a tumor, a hematoma, or edema. Blood volume can be increased through dilation of the vascular bed. CSF volume can be increased through obstruction in the ventricles, resistance to reabsorption, or, in rare instances, increased production of the CSF. Large brain tumors increase pressure by their mass, by blocking the rate of CSF reabsorption, or both. If the tumor is near the surface of the brain, it can cause inflamed meninges that may exude large quantities of fluid and protein into the CSF, thus increasing ICP. Hemorrhage or infection also causes increases in ICP. Large numbers of cells suddenly appear in the CSF and can almost totally block CSF absorption through the arachnoid villi. Regardless of the mechanism, when the volume added exceeds the volume that can be displaced, intracranial compliance is greatly reduced and ICP begins to increase.
Fig. 38-3 illustrates the relationship between intracranial volume and pressure. Phase I shows the success of compensatory mechanisms in maintenance of a constant ICP despite early increases in volume. In phase II, the limited capability of compensatory mechanisms has been exceeded and ICP begins to rise. In phase III, even a slight increase in volume causes a dramatic rise in ICP and thus results in complete decompensation and death. The shape of the curve may be altered by the rate at which the volume increases. Slowly developing increases in volume broaden the curve, whereas rapid increases narrow it.
Perianesthesia care for the patients with the potential for increased ICP requires an understanding of cerebral blood flow (CBF) and the factors that affect it; these factors become defective during increased ICP and are manipulated to reduce ICP. CBF is directly proportional to cerebral perfusion pressure (CPP) and inversely proportional to cerebrovascular resistance. CPP described as the pressure required to perfuse the brain.7 CPP is typically expressed as the difference between the mean arterial pressure (MAP) and the ICP:
Consequently, any increase in ICP or reduction in MAP reduces CPP and resultant CBF. Average CBF is 50 mL per 100 g/min.4 The CBF below which cerebral ischemia occurs has been termed the critical CBF, which is a flow rate of 16 or 17 mL per 100 g/min. Average CPP is 80 mm Hg.4 CBF begins to fail at a CPP of 30 to 40 mm Hg.6 Irreversible hypoxia occurs at a CPP less than 30 mm Hg. When ICP equals MAP, CPP equals zero and CBF ceases.
The lower limit of CBF autoregulation is the blood pressure below which vasodilatation becomes inadequate and CBF decreases. When CPP decreases to less than 60 mm Hg because of increases in ICP, autoregulation ceases to be beneficial or effective in regulation of CBF. Defective autoregulation aggravates pressure increases and creates critical or irreversible levels of ICP by increasing the blood volume within the cranium in an effort to maintain CBF. Defective autoregulation generally occurs when ICP exceeds 30 to 35 mm Hg. Eventually, autoregulation ceases altogether, and blood flow fluctuates passively with changes in arterial pressure, regardless of metabolic activity or regulation.
When ICP is increased, CPP and CBF are reduced, which renders the tissues ischemic. Ischemic cerebral tissue releases acid metabolites that cause a relatively fixed reduction in cerebrovascular tone. Autoregulation ceases and any increase in MAP causes further increase in cerebral blood volume and elicits further increase in ICP. CPP is reduced and thus causes ischemic areas to enlarge, such as those that surround an expanding intracranial mass. As can be seen in Fig. 38-4, a pathologic cycle ensues in which ICP and MAP eventually equilibrate, the CPP drops to zero, CBF stops, and death occurs.
Neurosurgical procedures
Stereotaxis
Stereotaxis enables precise localization of a specified point. A stereotactic frame is applied to the patient’s head, and the target tissue is located with the stereotactic frame’s coordinates and CT scanning. Hemorrhage evacuation, catheter, shunt, or electrode placement and implantation of radioactive seeds are all procedures that may benefit from the use of stereotaxis. Additional uses include destruction of intracranial sensory pathways and the treatment of intractable chronic pain.8
Laser surgery
The benefit of laser surgery is that it enables the neurosurgeon to access areas that were surgically inaccessible with conventional surgery. With laser surgery, the surgeon can dissect a structure without trauma to the surrounding tissue, shrink tumors, and coagulate blood vessels. See Chapter 47 for a complete discussion on laser surgery.
Anesthetic agents and intracranial pressure
Although the brain represents only 2% of the body’s total weight, it receives 12% to 15% of the total cardiac output because of the brain’s high metabolic rate.9 Anesthetic agents alter ICP by increasing or decreasing CBF and cerebral metabolic rate (CMR). In addition to the effects on ICP, some of these agents may also reduce systemic blood pressure and cause cerebral ischemia as a result of inadequate CPP.
Inhalation anesthetics
Isoflurane (Forane) at normocarbia has been shown to increase ICP. The initiation of hyperventilation simultaneously with the introduction of isoflurane prevents the increase in ICP that occurs at normocarbia. Isoflurane does not alter production of CSF and actually decreases resistance to absorption. It does not cause excitation of the central nervous system. Isoflurane produces reductions in CMR, and autoregulation is not impaired to any degree. The greater decrease in CMR may explain why CBF increases are minimal at low concentrations.
Intravenous anesthetics
Opioids, such as fentanyl, morphine, and meperidine, are typically classified as cerebral vasoconstrictors, with resultant reductions in CBF. This effect is readily abolished by vasodilation that can accompany opioid-induced ventilatory depression and the resultant increase in PaCO2. Inconsistencies do exist throughout the literature related to the actual effect CBF and CMR has on the unstimulated nervous system.9 During normocarbia, the combination of nitrous oxide and morphine does not significantly alter CBF or autoregulation. Fentanyl causes a reduction in CBF and ICP in patients with normal CSF pathways. Alfentanil and sufentanil may in fact cause increased ICP in patients with compromised cerebral compliance.
Propofol (Diprivan) reduces CMR, CBF, and ICP and increases cerebrovascular resistance in a dose-dependent manner. The use of propofol in patients with elevated ICP might not be appropriate because of the substantial decrease in MAP and resultant decrease in CPP. Although often used in rapid sequence intubation and continuous infusion during surgery, propofol has a short half-life and is therefore also used for sedation purposes in the neurosurgical patient; this facilitates the neurologic evaluation when needed.10
Dexmedetomidine (Precedex) is a sedative that allows for the patient to be easily aroused and often calm and cooperative while sedated; this enables the completion of the neurologic assessment. Although some precautions remain with this somewhat new drug, respiratory and hemodynamic stability are typically maintained with dexmedetomidine.6,11
Ketamine (Ketalar, Ketaject) can rapidly increase ICP and often reduce CPP, despite mild increases in blood pressure. Ketamine is generally contraindicated for use in neurosurgical patients unless the fontanels are open, CSF aspiration is instituted, or ventilatory control is maintained.
The use of controlled ventilation to produce PCO2 levels in the range of 30 to 38 mm Hg and administration of nitrous oxide and oxygen and possibly low concentrations of isoflurane, together with opioids and muscle relaxants, is a generally accepted anesthetic technique for the neurosurgical patient.12
Adjunctive drugs and interventions used to reduce intracranial pressure
Diuretics
Mannitol and furosemide (Lasix) are diuretics often used to control increased ICP. Mannitol, an osmotic diuretic, is the agent of choice for intracranial hypertension because it decreases intracellular edema.6 Appropriate infusion of crystalloid and colloid solutions is often necessary to prevent adverse changes in plasma concentrations of electrolytes and intravascular fluid volume because of the rapidity of diuresis. Potential complications of the use of mannitol include hyperosmolarity, electrolyte loss, changes in blood viscosity and coagulation, transient intravascular hypervolemia, and rebound or secondary elevation of ICP.
Whereas mannitol reverses edema, furosemide is a loop diuretic and is more effective in decreasing circulating blood volume, thus reducing edema.5 Furosemide is more effective than mannitol in reducing ICP and is the drug of choice in patients with congestive heart failure.
Corticosteroids
Barbiturate therapy
Initiation of barbiturate therapy is the last medication adjunct used in treatment of intracranial hypertension. The patient must have an intracranial pressure–monitoring device, usually started when ICP is more than 30 mm Hg for 30 minutes and CPP is less than 70 mm Hg. The most common barbiturate is pentobarbital, which lowers the ICP by inhibiting free radical–mediated lipid peroxidation, altering vascular tone, suppressing metabolism, and hopefully maintaining an ICP of 15 to 20 mm Hg.5
Temperature management
Hyperthermia is a common crisis in neurocritical care, occurring in an estimated 70% of head-injured patients.4,13 As the patient’s temperature increases, so does CBF and CMR, which could therefore also increase ICP.6 Temperature should be monitored closely, and the patient’s body temperature should be maintained at normothermia or even permissive hypothermia, which is becoming the current trend.12 Other advanced cooling methods include cool saline intravenous infusion and surface-cooling pads or wraps.4 Cooling measures such as antipyretics, hypothermia blankets, and other traditional cooling methods are used as needed.4,5 Methods such as intravascular temperature management may be effective as well.13
Positioning
Body positioning, among many other factors described previously is yet another aspect in proper care of the neurology patient. Positioning can affect ICP, CPP, and MAP.14 Although elevating the head of the bed to 30 degrees is preferred by most physicians, this is a topic of recent discussion.6
Intracranial pressure monitoring
The most precise indicator of the pressure state within the cranium is the CSF pressure, which is obtained through an ICP monitoring device. Trends in ICP, CPP measurement, and intracranial compliance are all functions of ICP monitoring.8 Monitoring of ICP is the standard of care for patients at risk for intracranial hypertension.
The ICP monitoring devices are categorized into two primary categories. The first category includes devices that use fluid or hydrostatic coupling to transmit to an external transducer. Ventricular catheters, subarachnoid bolts or screws, and subdural catheters fall into this group. The second group uses a transducer to directly monitor ICP. These intracranial devices use fiberoptics to transmit ICP pressures and can be placed in the intraventricular, subarachnoid, intraparenchymal, epidural, or subdural spaces (Fig. 38-5).6
The intraventricular catheter (IVC) is the most common and least expensive ICP monitoring device, and it allows for drainage of CSF.6 The tip is introduced into a CSF-containing ventricle via a twist-drill burr hole and is connected to an external transducer that converts the hydrostatic pressure force into a graph and numeric readout. The advantages of the IVC are that it provides a direct ICP reading and is easily kept patent. Another advantage is that CSF can be drained through the catheter for treatment of ICP elevations. In this way, the IVC may serve as a temporary artificial extension of the CSF-shunting compensatory mechanism. Intracranial compliance can also be tested with injecting fluid into the cranium and reading the responding pressure. If an abrupt and steep rise in ICP occurs, one can assume that compliance no longer exists and that the volume-pressure curve is a steep one. When the patient’s arterial pressure is monitored simultaneously, exact CPP can be calculated at any time. The ventricular catheter also has the advantage of allowing instillation of contrast media or air for study of the size and patency of the ventricle. The principal disadvantage of the IVC is the risk of infection and hemorrhage.
Pressure waves in increased intracranial pressure
Pressures waves are discussed in two separate categories: the ICP waveform and the ICP waveform trends (Fig. 38-6).
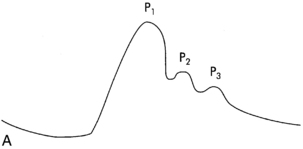
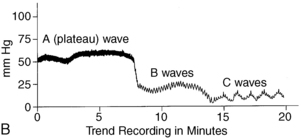
FIG. 38-6 A, ICP waveform. B, ICP waveform trends
(From Barker E: Neuroscience nursing, ed 3, St. Louis, 2008, Mosby.)
The ICP waveform is valuable at the bedside as a means for the nurse to determine the adaptability of the patient.5 The waves correlate with the patient’s heart rate. Ideally there are three or more peaks in the wave, with the P1 (percussion) wave as the first and most prominent. P1 is thought to be arterial in origin and consistent in its amplitude. P2, the tidal wave, is also arterial in origin and is related to the state of compliance. The last wave is the dicrotic wave or dicrotic notch and is venous in origin.3–5 Decreased intracranial adaptive capability and impaired autoregulation are thought to be associated with an elevation in the P2 wave.4
Three patterns have been identified with the ICP waveform trends. The first and most significant type is the A wave, which is more commonly called a plateau wave. These waves are associated with increases in ICP between 50 and 100 mm Hg that last for 5 to 20 minutes. They are seen only in advanced stages of increased ICP (the last phase of the volume-pressure curve) and superimpose themselves when the baseline ICP is elevated and exceeds 20 mm Hg. Early increases in mean systolic arterial pressure do not accompany plateau waves, and autoregulation is impaired. Thus, plateau waves signal hypoxia of brain cells and a decrease in CPP. They may cause both transient and irreversible damage to the brain and may be premonitory signs of acute incidents.
The second type of pressure wave pattern is called the B wave. These waves are sharp rhythmic oscillations with a sawtooth pattern that occurs every 30 seconds to 2 minutes. These waves can indicate increases in ICP as much as 50 mm Hg and are more commonly seen in patients with unstable increases in ICP usually from accelerations producing apneic episodes.5
The third type of pattern is the C wave. These waves are smaller rhythmic oscillations in ICP that occur 4 to 8 times per minute4,6 and indicate increases in ICP by as much as 20 mm Hg. These waves are associated with respiratory influence on blood pressure, but their significance is questionable.
Intracranial pressure assessment
Early signs of increased ICP are change in LOC (restlessness, confusion, agitation, irritability, lethargy), abnormal pupillary function, deterioration of motor function, and severe headache.4,8 Nausea, vomiting, paralysis, visual field deficits, conjugate deviation of the eyes, sensory loss, and nuchal rigidity are also early signs. The presence of these signs may or may not confirm a diagnosis of increased ICP, but they can still inform treatment while treatment is effective.
Most hospitals with the capacity for cranial surgery also have the capacity for continuous ICP monitoring. It is still important to be able to recognize signs and symptoms of intracranial hypertension without the assistance of an ICP monitor. Thus, the traditional signs and symptoms of increased ICP are discussed here, although they are not precise or infallible as indicators of increased ICP. At the least, they indicate that “something is not right” and that constant vigilance and further investigation are necessary. Even the transient appearances of these pressure signs are important; they indicate the development of a highly delicate and unstable intracranial situation, a sign that the patient may be having plateau waves.
Perianesthesia nursing management
Reports should be taken from both the anesthesia provider and the surgeon. Of particular importance are surgical procedure, pathologic findings, bone flap presence, allergies, preexisting medical problems, anesthetics, and any problems that occurred during surgery. Special positioning orders or restrictions, the presence of drains, and known CSF leaks must be noted. The Glasgow Coma Scale (GCS; Table 38-1) is a widely used neurologic assessment tool because of its simplicity, consistency, and reliability between raters who use it. However, the GCS cannot be used to assess subtle changes in the patient’s neurologic status. When the GCS is used, the patient’s responses are scored on a scale of 3 to 15. A score of 3 indicates coma, and a score of 15 indicates a fully alert oriented person with all neurologic functions intact.
CATEGORY | RESPONSE | SCORE |
---|---|---|
Eye opening | Spontaneous | 4 |
To speech | 3 | |
To pain | 2 | |
None | 1 | |
Best verbal response | Oriented to person, place, and time | 5 |
Confused | 4 | |
Inappropriate words | 3 | |
Incomprehensible sounds | 2 | |
No response | 1 | |
Best motor response | Obeys commands | 6 |
Localizes to pain | 5 | |
Withdrawal from pain | 4 | |
Abnormal flexion | 3 | |
Abnormal extension | 2 | |
Flaccid | 1 |
Vital signs
Airway patency is ensured, and the rate, depth, and rhythm are noted. If the rhythm is irregular, its pattern should be determined. Changes in the respiratory pattern may indicate injury to the respiratory center of the brain and the severity of the neurologic injury (Fig. 38-7). If the patient requires a ventilator, the machine should be checked for proper functioning and settings; however, mechanical ventilation can mask changes in the respiratory pattern.
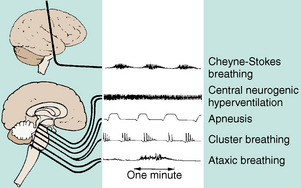
FIG. 38-7 Abnormal respiratory patterns with corresponding levels of central nervous system activity.
(From Urden LD et al: Critical care nursing, ed 6, St. Louis, 2010, Mosby.)
For many years, the nursing literature has documented a relation between changes in blood pressure and pulse and increases in ICP. These changes are often referred to as Cushing reflex, Cushing triad, or Cushing response. Cushing reflex is described as elevated systolic blood pressure, bradycardia, and widening pulse pressure. Additional increases in ICP can lead to Cushing triad, which is described as bradycardia, hypertension, and bradypnea. Cushing reflex and triad are late clinical signs of increased ICP and may indicate brain stem herniation. Patients with head injury often have a higher than normal blood pressure and heart rate that may be the result of pain, hypoxia, and agitation or the release of endogenous catecholamines.
Level of consciousness
The most important indicator of brain function is the LOC, but it is not necessarily indicative of altered ICP. A decreased LOC in the PACU can be caused by the lingering effects of the anesthesia or by neuromuscular-blocking agents sometimes used with patients on positive-pressure ventilation. A change in LOC may also be the result of hypoxia, hypoglycemia, vitamin deficiency, or fluid and electrolyte imbalances. Other underlying pathologic changes can cause alterations in LOC. For assessment of LOC, a description of the patient’s response is best instead of vague terms such as stuporous, semiconscious, or unconscious. A standard assessment form such as the GCS (see Table 38-1) should be available for assessment of the LOC. A change in LOC may also be indicative of deterioration or improvement in the patient’s condition.
Pupillary activity
Pupillary reactions are controlled by the third cranial nerve. In assessment of the pupils, the perianesthesia nurse should examine both simultaneously for shape, size, and equality. Normal pupils are round and, at a midpoint diameter, within the range of 1 to 9 mm. Instead of using terms such as constricted or dilated, measurement of the diameter directly with a pocket millimeter ruler is more precise. Test the direct light reflex of each pupil with a small bright flashlight. Normally the pupil constricts briskly. If it reacts sluggishly or not at all, the reaction is abnormal. To test the consensual light reflex, hold both eyelids open, shine the light in one eye, and observe the other pupil. The opposite pupil should constrict simultaneously with the lighted one, although perhaps not to the same degree.
Nursing care in the postanesthesia care unit
The PACU nurse has three primary responsibilities in the care of the neurosurgical patient: (1) to institute measures of care to sustain optimal physiologic function in the perianesthesia patient, (2) to recognize and prevent conditions that increase ICP beyond normal limits, and (3) to detect and communicate signs and symptoms of the patient’s condition to the physician (Box 38-1).
BOX 38-1 General Postoperative Considerations
Airway, oxygenation status
Neurologic assessment
• Differential diagnosis for decreased level of consciousness
• Differential diagnosis for respiratory depression
• ICP, CPP, and acceptable hemodynamic parameters
• Appropriate patient positioning
• Assess dressing and other sites of intervention (e.g., pin sites if the patient was placed in rigid skull fixation for posterior cervical spine surgery)
Documentation
Adapted from Bitters L: Perioperative surgical considerations. In Bader MK, Littlejohns LR, editors: AANN core curriculum for neuroscience nursing, Glenview, Ill, 2010, American Association of Neuroscience Nursing.
Spinal surgery
In the United States there are approximately 200,000 people living with SCI, and 12,000-20,000 new cases are estimated to occur annually.15 The goal of surgical intervention is minimization of complications related to SCIs, spinal cord tumors, or developmental abnormalities. Complete or incomplete SCI, bony fragments in the canal, unstable dislocation, and evidence of cord compression are some indications for immediate surgical intervention.
Diagnostic tools
Several methods are used in the diagnosis of injury or disease involving the spine or spinal canal.
Conventional radiography and fluoroscopy are used to identify fractures and fracture-dislocations. Narrowing of an intervertebral space is sometimes evident as a result of a herniated nucleus pulposus (slipped disk). Fluoroscopy is used to show instability of the injured part on manipulation. Splintered or displaced bone fragments and radiopaque foreign bodies, such as bullets or other metal fragments, are also seen on radiographs. Radiographs also show abnormalities such as scoliosis and osteoporotic and arthritic changes. Tumors may be evidenced by erosion, calcium deposits within the mass, increased interpediculate distance, enlargement of an intervertebral foramen, or collapse of a vertebra.
Injuries of the spine
The spine protects the spinal cord and the terminal nerve roots. Injuries to the spine and spinal cord occur as a result of flexion, hyperextension, rotation with both flexion and extension, compression, and penetrating wounds (Fig. 38-8). Head injuries often accompany injuries to the spine and vice versa. The cervical spine is extremely mobile and therefore particularly susceptible to injuries that hyperflex or hyperextend the neck. Propulsion can occur anteroposteriorly or laterally. The spinal cord is relatively large in the cervical area and sustains damage fairly easily after injury. This area is unique in that the superior portion of C2 lacks a vertebral body. Instead, the neck has a dens, or projection, called the odontoid. Many injuries to the odontoid extend into C1, or atlas, which has no vertebral body at all.
The thoracic spine is fixed by the ribs, but the lumbar spine is not; therefore an increased incidence rate is seen of injury to the thoracic, lumbar, or sacral regions of the spine. Motor vehicle crashes are the leading cause of spinal cord injuries.15
Complete spinal cord lesion
1. There is immediate loss of all sensory, motor, autonomic, and reflex functions below the level of the injury from spinal shock. Spinal shock can persist for days or weeks, depending on the injury and the patient’s general state of health. It usually lasts 4 to 8 weeks.
2. Urinary retention as a result of bladder sphincter paralysis is present.
3. Paralytic ileus with progressive abdominal distention is present.
4. There is respiratory insult or cessation. Injury to the lower cervical or upper thoracic spine results in cessation of intercostal function. In this event, respiration is under the sole stimulus of the phrenic nerve, and breathing is diaphragmatic. Injury to the cord at the levels of C3 to C5 affects the phrenic nucleus and thus paralyzes the diaphragm and causes respiratory failure.
5. There is a loss of sweating below the level of the lesion.
6. Point tenderness over the injured part is present. Crepitus may or may not be present.
Initial therapeutic efforts are directed at the preservation of life. Mechanical stability, protection of nervous tissue, and freedom from pain are long-term therapeutic goals.
Surgical intervention for spinal cord injuries
General contraindications to surgery are the existence of associated life-threatening injuries, depressed respiratory function caused by high cervical injuries, lack of skilled personnel and necessary equipment, improved neurologic status, and need for stabilization.6
Perianesthesia nursing care for the patient with spinal cord injury
Complications associated with spinal cord injury
Spinal shock
Spinal shock is a form of neurogenic shock characterized by a loss of motor, sensory, autonomic, and reflex activity below the level of the lesion and resulting in flaccid paralysis and paralytic ileus. The classic signs and symptoms of spinal shock include systemic hypotension, bradycardia, and hypothermia. Spinal shock is a condition that occurs immediately after injury or up to hours after an SCI that progressively worsens and may last hours to months, depending on severity of the injury.6
Bradycardia
Bradycardia is associated with spinal shock and nursing interventions such as manipulation of an endotracheal tube, suctioning, turning, and insertion of a nasogastric tube, which may elicit a vasovagal response that leads to bradycardia. Nursing interventions should closely monitor for and provide prevention from a vasovagal response.
Autonomic dysreflexia
Autonomic dysreflexia is a syndrome of massive imbalanced reflex sympathetic discharge.16 It occurs in patients with SCI greater than the splanchnic sympathetic outflow (T5-T6)16 and after the resolution of spinal shock and the return of reflex activity. Autonomic dysreflexia results from excessive reflex stimulation of the sympathetic nerves below the level of injury. Causes of the reflex stimulation include bladder distension, fecal impaction, and noxious stimuli. Symptoms of autonomic dysreflexia are pounding headache, excessively high hypertension, decreased heart rate, profuse sweating, and flushed skin above the level of injury, pallor and goose bumps below the level of the injury, anxiety, and visual disturbances. Treatment is aimed at removing the noxious stimulus and preventing complications from hypertension.
Skin breakdown
As with the patient for cranial surgery, skin care for the patient with SCI is an important aspect of PACU nursing care. Skin breakdown is one of the most obvious, costly, and detrimental complications that a patient with SCI can have. The patient must be turned and repositioned at least every 2 hours to prevent skin breakdown and damage to underlying body tissues. A specialty surface such as an air mattress or alternating pressure mattress may be necessary to prevent skin breakdown.
Urologic complications
Long-term catheterization, osteoporosis, decreased muscle tone, fluid and electrolyte abnormalities, alterations in cardiovascular dynamics, anemia, and catabolism contribute to urologic complications. Stasis, calculi and fistula formation, and chronic urinary tract complications can lead to septicemia. Pneumonia and septicemia have the greatest effect on reduced life expectancy in patients with SCI.17
Herniated intervertebral disk
Herniated intervertebral disk is also known as herniated nucleus pulposus (Fig. 38-9).8 This condition can occur in any of the intervertebral disks, but is most commonly found in one of the last two lumbar interspaces. Pain and some degree of compromise in sensory or motor function along the distribution of the involved nerve are common preoperative findings. Before surgical intervention is undertaken, diagnostic confirmation is sought and the suspected herniated nucleus pulposus is differentiated from tumor, subluxation of the facets, or rheumatoid spondylitis.
Surgery consists of partial hemilaminectomy and removal of the diseased disk. If fusion is necessary to prevent recurrence of pain or deformity, a bone graft is removed from the iliac crest or tibia and placed as a bridge over the defective space. Spinal fusion lengthens the operative procedure and requires a second operative wound site; therefore a greater potential for postoperative complications exists, and the recuperative phase may be lengthened. The threat of shock is also greater because of increased blood loss and pain.
Intraspinal neoplasms
• Intramedullary tumors: those that arise solely from the substance of the cord
• Extradural-extramedullary tumors: those that arise outside the dura, either in the epidural space, vertebrae, or surrounding tissues
• Intradural-extramedullary tumors: those that arise within or under the dura but do not invade the cord
Postoperative care for spinal cord injury surgical interventions
Perianesthesia care of patients for lumbar surgery should include keeping the head of the bed flat and log-rolling the patient to help maintain proper body alignment, promote skin integrity, and minimize discomfort. The surgical site should be inspected for drainage and hematoma, and if spinal fusion was performed, the donor site should also be inspected. Assessment of the patient’s comfort should be performed frequently because of muscle spasms that are often associated with lumbar surgery. The neurologic examination should include assessment of sensation in the lower extremities and notation of the presence of tingling, numbness, or paralysis. The pedal pulses, color, temperature, and capillary refill of the lower extremities should also be assessed.
Summary
Perianesthesia care of the neurosurgical patient can be complex. However, modern pharmacology and technology in addition to our improved understanding of patient’s physiology should facilitate the removal of many of the challenges in the care of these patients. Consequently, for a neurosurgical procedure or central nervous system trauma that necessitates radiologic studies with anesthesia, the anatomy and physiology (see Chapter 10) and pathophysiology have been described to include the monitoring equipment that can be used, all in an effort to ensure that outcomes are as favorable as possible. Besides the basic principles of PACU nursing management, which include airway management, ventilatory assistance, and hemodynamic support, the neurosurgical case entails unique preparation and management. This chapter has provided an in-depth discussion of the perianesthesia care of the neurosurgical patient in an effort to facilitate favorable outcomes.
1. Czernin J, Schelbert H. PET/CT imaging: facts, opinions, hopes, and questions. The Journal of Nuclear Medicine.2004;45:1S–3S.
2. Phillips N. Berry & Kohn’s operating room technique, ed 11. St. Louis: Mosby; 2007.
3. Urden LD, et al. Critical care nursing, ed 6. St. Louis: Mosby; 2010.
4. Bader MK, Littlejohns LR. AANN core curriculum for neuroscience nursing, ed 5. Chicago, Ill: AANN; 2010.
5. Barker E. Neuroscience nursing, ed 3. St. Louis: Mosby; 2008.
6. Hickey J. The clinical practice of neurological and neurosurgical nursing, ed 6. Philadelphia: Lippincott Williams & Wilkins; 2009.
7. Noble KA. Traumatic brain injury and increased intracranial pressure. J Perianesth Nurs. 2010;25:242–247.
8. Schick L, Windle PE. Perianesthesia nursing core curriculum: preprocedure, phase I and phase II PACU nursing. ed 2. St. Louis: Saunders; 2010.
9. Miller RD, et al. Miller’s anesthesia, ed 7. Philadelphia: Churchill Livingstone; 2005.
10. Souba WW, et al. ACS surgery: principles and practice. ed 6. New York: BC Decker; 2007.
11. Kemp KM. Precedex: Is it the future of cooperative sedation. Nursing.2008;38:7–8. Suppl Critical
12. Yentis SM, et al. Anaesthesia and intensive care A-Z, ed 4. Edinburgh: Churchill Livingstone; 2009.
13. Fisher M, et al. Keep the brain cool – endovascular cooling in patients with severe traumatic brain injury: a case series study. Neurosurgery.2010;68:867–873.
14. Ledwith MB, et al. Effect of body position on cerebral oxygenation and physiologic parameters in patients with acute neurological conditions. J Neurosc Nurs. 2010;42:280–286.
15. Centers for Disease Control and Prevention: Spinal cord injury (SCI): fact sheet. available at www.cdc.gov/TraumaticBrainInjury/scifacts.html
16. Campagnolo DI. Autonomic dysreflexia in spinal cord injury. available at emedicine.medscape.com/article/322809-overview
17. Spinal cord injury facts and figures at a glance. National Spinal Cord Injury Statistical Center; February 2011.
Aitkenhead A, et al. Textbook of anaesthesia, ed 5. Edinburgh: Churchill Livingstone; 2007.
Alspach J. Core curriculum for critical care nursing, ed 6. Philadelphia: Saunders; 2005.
Atlee J. Complications in anesthesia, ed 2. Philadelphia: Saunders; 2007.
Baird MS, Bethel S. Manual of critical care nursing, ed 6. St. Louis: Mosby; 2011.
Barash P, et al. Clinical anesthesia, ed 6. Philadelphia: Lippincott Williams & Wilkins; 2009.
Barrett KE, et al. Ganong’s review of medical physiology, ed 23. New York: McGraw-Hill Medical; 2009.
Brain Trauma Foundation, et al. Guidelines for the management of severe traumatic brain injury. ed 3. Guideline XV: Steroids; 2007:S-91–S-95.
Brunton L, et al. Goodman and Gilman’s the pharmacological basis of therapeutics, ed 11. New York: McGraw-Hill Professional; 2005.
Chulay M, Burns S. AACN essentials of critical care. St. Louis: AACN; 2005.
Fleisher LA. Anesthesia and uncommon diseases, ed 5. Philadelphia: Saunders; 2007.
Gallager C, Issenberg B. Simulation in anesthesia. Philadelphia: Saunders; 2007.
Hall J. Guyton & Hall’s textbook of medical physiology, ed 12. Philadelphia: Saunders; 2010.
Lynn-McHale Wiegand DJ. AACN Procedure manual for critical care, ed 6. St. Louis: Saunders; 2011.
McCance K, Huether S. Pathophysiology: the biologic basis for disease in adults and children. ed 5. St. Louis: Mosby; 2006.
Nagelhout J, Plaus K. Nurse anesthesia, ed 4. St. Louis: Saunders; 2009.
Saunders, Stoelting R, Miller R. Basics of anesthesia, ed 6. Churchill Livingstone.: Philadelphia; 2011.
White P. Perioperative drug manual, ed 2. Philadelphia: Saunders; 2005.