20 Care of the Child with Burns
Pearls
• Intentional injury (child abuse) should be considered and ruled out as a cause of burns and scalds in children.
• Burns are painful; therefore analgesia should be provided and supplemented as needed before burn care.
• Formulas developed to estimate burn size in adults should not be used to calculate the burn surface area and fluid resuscitation requirements for children.
• Children with large burns should receive nursing and medical care in units with nurses and physicians who have pediatric expertise. Strong consideration should be given to transfer of a child to a pediatric critical care unit when large burns or complicating factors, such as smoke inhalation, are present.
Introduction
The incidence of pediatric burn injuries has declined as a result of preventive measures and legislation. However, more than 1 million burn injuries still occur each year in the United States. Although most of these burn injuries are minor, each year in the United States approximately 45,000 patients suffer moderate to severe burns that require hospitalization. Of these cases, 67% are young males, and 40% are children younger than 15 years.31a Burns are the second leading cause of unintentional death in children younger than 5 years. It is estimated that the number of serious disabilities from burns is triple the number of deaths. Three fourths of these burns are thought to be preventable.22
Eighty-five percent of thermal injuries in children occur at home, usually in the kitchen or bathroom. Infants and toddlers are injured most frequently by scald burns (Table 20-1),1 whereas contact burns become more common once the infant is crawling or walking. Flame burns are seen in children 2 to 4 years of age and older and are the most common cause of burn injury in children 5 to 18 years of age. Electrical and chemical burns are uncommon in children and can be lethal if they are severe.128
Essential anatomy and physiology
The skin is composed of three layers: epidermis, dermis, and subcutaneous tissues (Fig. 20-1). The epidermis is a superficial layer of stratified epithelial tissue that is composed of five microscopic levels of maturing cells. The epidermis is thinner in infants than in older children, and its thickness also varies over parts of the body. This layer is constantly shed to the environment, so that it regenerates continually. After a superficial burn, the epidermis will regenerate because portions of the epidermal appendages are present.
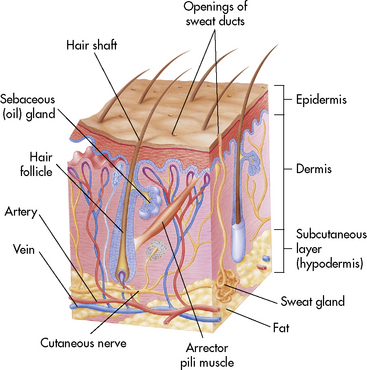
Fig. 20-1 Anatomy of the skin.
(From Thibodeau GA, Patton KT: Anatomy and physiology, ed 5, St Louis, 2003, Mosby.)
Severity and Classification of Injury
Depth of Burn
The severity of the burn injury is determined by estimating the depth and extent of the injury. The degree of tissue destruction is affected by the burning agent, its temperature, and the duration of exposure to the heat source. Healthy skin can tolerate brief exposure to temperatures up to 40° C (104° F) without injury, but higher temperatures will produce burns. Severity of the injury increases as the temperature and duration of contact increase.180
Classically, description of burn injury refers to the three concentric zones of tissue damage.143,180 The central area of the burn wound, called the zone of coagulation, is injured most severely and is characterized by coagulation necrosis. The zone of stasis is an area of direct but milder injury, which can be damaged further if ischemia develops.232 The zone of hyperemia is the area of tissue most peripheral to the initial burn and is injured only minimally.
A second method of burn classification describes the specific depth of injury (Table 20-2). A first-degree burn involves the top portion of the epidermis and does not extend into the dermis layer (Fig. 20-2). The burn area is characterized by erythema, mild edema, pain, and blanching with pressure. There is no vesicle formation. First-degree burns (e.g., sunburn) heal spontaneously without scarring in 7 to 10 days.
Extent of Injury
A rapid method of calculating burn area in adolescents and adults, developed in the 1940s by Pulaski and Tennison,174a is called the rule of nines (Fig. 20-3).1 In the rule of nines, each upper extremity and the head constitute 9% of the TBSA, and the lower extremities and the anterior and posterior trunks are each 18% of TBSA. The perineum, genitalia, and neck comprise the remaining 1% of the TBSA. A quick estimate of burn size can also be obtained by using the patient’s palm to represent 1% of TBSA and transposing that measurement to estimate the wound size.
Use of the rule of nines can be misleading in children because the child’s body proportions differ from those in adolescents and adults. In children, the head and neck constitute a relatively larger portion of the TBSA, and the lower extremities constitute a smaller portion. For example, an infant’s head constitutes 19% of TBSA, compared with 9% in an adult. Thus, a modified rule of nines, based on the anthropomorphic differences of infancy and childhood, is generally used to assess pediatric burn size (see Fig. 20-3). Clinical criteria can also be used to estimate the percentage of TBSA burned, based on the patient’s age and the body part burned (see Classification of Burns).
Another widely used method of determining the extent of pediatric burn injury is the Lund and Browder method (Fig. 20-4). This method allows for changes in body surface area as the average-sized child grows.119
Classification of Burns
After providing first aid and initiating appropriate fluid resuscitation, providers should consider transferring the patient to a tertiary burn center. Burn units with experienced multidisciplinary teams are best prepared to treat patients with major burns. In addition to physicians and nurses, respiratory and rehabilitation therapists play a critical role in managing acute burns. Any patient who sustains a major burn injury, as defined by the American Burn Association (Box 20-1), should be transferred to a nearby burn center for further care.
Box 20-1 American Burn Association Criteria for Major Burn Injury
• Second- and third-degree burns over more than 10% TBSA in patients less than 10 or greater than 50 years
• Second- and third-degree burns over more than 20% TBSA in other age groups (i.e., between 10 and 50 years)
• Third-degree burns over more than 5% TBSA in any age group
• Burns involving the face, hands, feet, genitalia, perineum, and skin overlying major joints
• Significant electrical burns, including lightning injury
• Burns with significant concomitant trauma
• Burns with significant preexisting medical disorders
• Burns in patients requiring special social, emotional, and rehabilitative support (including suspected inflicted injury and neglect)
Pathophysiology of a Burn
Local Circulatory Destruction
The vessels supplying the burned skin are occluded, and there is reduction in or cessation of blood flow through the arteries and veins. Release of vasoactive substances (especially histamine) from injured cells will produce vasoconstriction,143 and peripheral vessel thrombosis ultimately may occur. The reduction in skin perfusion can produce tissue necrosis and increase the depth of the burn.
Capillary Permeability (Third-Spacing) Period
As protein rich fluids, electrolytes, and plasma escape into the interstitial space, peripheral edema develops. Movement of proteins into the interstitial space will increase tissue colloid osmotic pressure, enhancing the intravascular-to-interstitial fluid shift.136
Fluid lost from the vascular space is relatively isotonic; therefore if it is replaced with isotonic or hypertonic fluids, electrolyte balance should be maintained. Dilutional hyponatremia, hypocalcemia, and hypomagnesemia are seen occasionally,213 particularly if antidiuretic hormone secretion is significant (antidiuretic hormone secretion causes water retention in excess of sodium—see Chapter 12). It is rarely necessary to replace these electrolytes if isotonic fluids are administered; however, electrolyte balance should be monitored closely. Hypotonic fluids (e.g., 5% dextrose and water or 5% dextrose and 0.45% sodium chloride) should not be administered during this period.
The concentration of base bicarbonate in the extracellular fluid decreases after a burn, and fixed acids are released from the injured tissues into the extracellular fluid, including the plasma. These acids normally are excreted by the kidney and buffered by respiratory compensation. If fluid resuscitation is inadequate, or respiratory function is compromised, the patient may develop metabolic acidosis. Young infants are less able to compensate for significant metabolic acidosis, because the infant kidneys are unable to excrete large quantities of acids or absorb large quantities of bicarbonate.179
Cardiovascular Dysfunction
Cardiac output falls after a burn as the result of decreased intravascular volume and the development of myocardial dysfunction.123 Myocardial dysfunction after a burn is not explained entirely by intravascular fluid loss. Within 30 minutes after a large burn (i.e., 50% or more of TBSA), cardiac output may decrease to 30% of preburn levels and may remain depressed for 18 to 36 hours. Cardiac output returns to normal levels long before plasma volume has been restored completely.47
The fall in cardiac output after a burn has been attributed to the presence of circulating myocardial depressant factor or the development of a catecholamine (stress induced) increase in systemic and pulmonary vascular resistances and increased ventricular afterload.229 Treatment of low cardiac output requires supportive care; the efficacy of vasoactive (inotropic) drug therapy in the treatment of this cause of myocardial dysfunction has not been determined.
In general, treatment of inadequate cardiovascular function requires support of maximal oxygen delivery (including support of oxygenation, ventilation, and cardiac output) with titration of intravenous volume administration. The effectiveness of vasoactive agents for children with significant burns has not been studied (refer to discussion of shock in Chapter 6).
Pulmonary Injuries
Respiratory insufficiency can result from the inhalation of superheated air, steam, toxic fumes, or smoke, and it is a major cause of morbidity and mortality in burned children.94,97,126,146,197 This respiratory failure may result from airway edema or obstruction or from microcirculatory changes and increased capillary permeability. Pulmonary edema can result from inhalation injuries, excessive volume administration during resuscitation, or sepsis.
Inhalation of smoke, steam or other irritants will produce upper airway edema, erythema, and blistering. Progressive edema can cause upper airway obstruction. Ciliated epithelial cells may be damaged during inhalation, so that foreign particles can enter the bronchi. The damaged mucosal layer may slough 48 to 72 hours after a burn, producing acute airway obstruction.30,94
Damage to the pulmonary parenchyma can result from an inhalation injury and can complicate shock and fluid resuscitation (see Respiratory Failure, later in this chapter and Acute Respiratory Distress Syndrome in Chapter 9). Increased alveolar capillary membrane permeability will produce pulmonary edema with resultant intrapulmonary shunting and hypoxemia, decreased lung compliance, and increased work of breathing.118
Carbon Monoxide Poisoning
Carbon monoxide (CO) is produced in almost every fire, and CO poisoning can cause immediate death during the fire or death during the first 12 hours after a burn. CO has much higher affinity for hemoglobin than does oxygen; as a result, it will bind tightly with hemoglobin, forming carboxyhemoglobin (COHb). Each gram of hemoglobin bound to CO is unable to carry oxygen, so impaired oxygen transport, decreased oxygen delivery, tissue hypoxia, and metabolic acidosis will result if CO levels are high. COHb levels greater than 40% usually produce significant tissue and organ ischemia and dysfunction, and levels greater than 60% are usually fatal.78,216
Gastrointestinal Dysfunction
Gastrointestinal ischemia can increase the permeability of gastrointestinal mucosa to gram-negative bacteria and endotoxins. As a result, translocation of gram-negative bacteria or endotoxin can occur and may precipitate gram-negative sepsis (see Septic Shock in Chapter 6, and Septic Shock: Mediators of the Septic Cascade in the Chapter 6 Supplement on the Evolve Website).
The incidence of Curling’s ulcer is unknown, because it typically is diagnosed at autopsy. Superficial gastric and duodenal mucosal changes are common in children with major burns,67 but ulcer prophylaxis has ensured that clinically significant bleeding and ulceration are still relatively uncommon.
Gastrointestinal ulceration may produce pain, hemorrhage, or perforation. Gastric suction and stool samples should be tested for the presence of blood (heme protein), and the use of antacids or sucralfate (a hydrogen ion diffusion barrier) should be considered.131 Administration of histamine receptor antagonists (e.g., cimetidine or ranitidine) is controversial, because the morbidity of these drugs may be higher than the risk of stress ulceration. Severe pneumonias may result from aspiration of gastric bacteria that can flourish after these drugs are administered. The gastric pH should be maintained at 3.5 to 5.0 (see Chapter 14).
Metabolic Changes
The patient with a burn is in a hypermetabolic state, with high oxygen consumption and caloric requirements. Metabolic rate reaches its peak at double (or more) normal values approximately 4 to 12 days after a burn.5b Catecholamine secretion activates the stress response, and heat production and substrate mobilization will result in protein and fat catabolism, increased urinary nitrogen losses, and rapid utilization of glucose and calories.70 An increased metabolic rate continues until after the burn is healed or covered by graft.
Central thermoregulation is altered at this time, and the hypermetabolic condition often produces a low-grade fever.205 In contrast, heat loss and a fall in body temperature may be observed in the very young child with an extensive burn.
Because a burn is a major body stress, muscle protein catabolism increases to provide amino acids for gluconeogenesis and fuel sources for local tissue needs.69 Insufficient protein administration and nutrition will result in a marked catabolic state (negative nitrogen balance) and major muscle loss. Large amounts of urea in the urine indicate increased nitrogen loss.218
Thermal injury and hypermetabolism result in increased serum free fatty acids. Hydrolysis of stored triglycerides is accelerated, and catecholamine secretion stimulates mobilization of fat stores. Hypoalbuminemia results from increased protein loss at the burn surface and can, in turn, reduce fatty acid transport.75
Compromise in Immune Function
After a burn, several circulating immunosuppressive substances are present. Nonspecific suppressor T cells compromise lymphocyte response for approximately 48 hours.154 Leukocyte phagocytosis is reduced, and the reticuloendothelial system is often depressed.220 Burn toxin, a high-molecular-weight protein, is thought to contribute to postburn immunosuppression. The patient’s immune function may be compromised further by the application of topical antimicrobial agents and the insertion and contamination of intravascular catheters.
Infection or injury can activate the complement system, resulting in a normal inflammatory response.88 Extensive burns result in a decrease in serum complement levels and a potential reduction in the inflammatory response during infection (see Septic Shock in Chapter 6, and Septic Shock: Mediators of the Septic Cascade in the Chapter 6 Supplement on the Evolve Website).
Common clinical conditions
Care of the child with burns requires support of cardiorespiratory function, prevention of infection, and preparation of the burn surface for healing or grafting. In addition, potential complications of the burn and its treatment must be prevented. An overview of this nursing care is provided in the nursing care plan (Box 20-2), and the major potential patient problems are reviewed in the following discussion.
Box 20-2 Nursing Care of the Child with Thermal Injuries
Inadequate Cardiac Output and Tissue Perfusion (Alteration in Tissue Perfusion) Related to: Extravascular Fluid Shift and Relative Hypovolemia, Inadequate or Delayed Fluid Resuscitation, Constriction of Eschar
Expected Patient Outcome
Patient will demonstrate adequate tissue perfusion as evidenced by:
Nursing Activities
• Constantly assess fluid balance, systemic perfusion. Monitor for signs of hypovolemic shock, including: tachycardia, oliguria, cool skin and cool extremities, weak peripheral pulses, altered level of consciousness, negative fluid balance, low central venous or pulmonary artery wedge pressure, development of lactic acidosis, and absence of hepatomegaly (by clinical examination) and of cardiomegaly (on chest radiograph).
• Calculate fluid replacement requirements and discuss inadequate fluid administration or excessive fluid losses with the on-call provider immediately.
• Administer fluid boluses and maintenance fluids as needed to restore adequate intravascular volume and systemic perfusion and to replace ongoing fluid losses.
• Assess perfusion and appearance of and movement and sensation in extremities and digits.


• Assist with escharotomies or fasciotomies on arms, legs, and chest as needed.
• Perform passive and active range-of-motion exercises to extremities as ordered.
• Position patient carefully to prevent compromised blood flow to extremities.
Potential Hypovolemia or Inadequate Fluid Volume Related to: Fluid Loss Through Evaporation from Burn Surface, Increased Capillary Permeability and Extravascular Fluid Shift, Inadequate Fluid Administration, Excessive Fluid Losses Through Fever, Diarrhea
Expected Patient Outcomes
• Patient will demonstrate adequate intravascular volume as evidenced by: effective systemic perfusion, balanced fluid intake and output (with consideration of fluid loss from surface of burn), urine volume of 1 mL/kg per hour, electrolyte balance, central venous pressure or pulmonary artery wedge pressure of approximately 2 to 5 mm Hg (or higher as needed to maintain adequate systemic perfusion), normal arterial pH, and normal serum lactate.
• Patient will demonstrate no signs of hemoconcentration or dehydration, such as dry mucous membranes, poor skin turgor, increase in serum electrolyte concentration and hematocrit, sunken fontanelle (in infants), and oliguria with increased urine specific gravity.
Nursing Activities
• Monitor patient systemic perfusion: signs of hypovolemia include signs of poor systemic perfusion associated with a low central venous pressure or pulmonary artery wedge pressure, small heart size on chest radiograph, and absence of hepatomegaly. Report these findings to the on-call provider immediately.
• Monitor for signs of dehydration, including: sunken fontanelle in infants, dry mucous membranes, poor skin turgor, evidence of weight loss, rise in serum electrolyte concentration, and increase in hematocrit. Report findings to the on-call provider.
• Monitor urine output and fluid balance every hour; report oliguria or negative fluid balance to the on-call provider (consider fluid loss through burn).
• Titrate fluid administration as needed (and with physician or other provider order) to maintain systemic perfusion.
• Monitor heart size and evidence of pulmonary edema on chest radiograph; discuss these findings with on-call provider.
• Monitor electrolyte balance, serum albumin, and hematocrit.
• Obtain daily weights, report them to the on-call provider, and discuss changes in fluid requirements.
Potential Hypervolemia or Fluid Volume Excess Related to Excessive Fluid Administration and Renal Failure
Nursing Activities
• Monitor systemic perfusion and fluid balance; report signs of congestive heart failure (tachycardia, tachypnea, periorbital edema, hepatomegaly, increased respiratory effort, cardiomegaly, and oliguria), excessive weight gain, or positive fluid balance to the on-call provider (note that most patients will demonstrate weight gain following fluid resuscitation after a burn).
• Assess for evidence of pulmonary edema, including crackles and increased respiratory effort; be prepared to support oxygenation and ventilation as needed.
• Palpate liver margin; report the presence or progression of hepatomegaly to the on-call provider.
• Monitor electrolyte balance; report a fall in electrolyte concentration or hematocrit to the on-call provider.
Potential Airway Obstruction Related to: Airway Inflammation, Pulmonary Interstitial Edema, Reduced Ciliary Function Following Inhalation Injury, Altered Level of Consciousness
Expected Patient Outcomes
• Patient will demonstrate patent airway as demonstrated by: normal spontaneous respiratory rate and effort, adequate depth of respirations and air movement, effective oxygenation and carbon dioxide removal (per arterial blood gases, pulse oximetry and exhaled carbon dioxide tension [PETCO2]), and absence of crackles and stridor
• If patient develops airway obstruction, intubation and appropriate support will be provided immediately.
Nursing Activities
• Monitor patient respiratory rate, effort, and air movement. Notify on-call provider of signs of airway obstruction, including tachypnea, retractions, nasal flaring, stridor, or weak cry. Be prepared to assist with emergency intubation as needed. Resuscitation bag and mask with oxygen source should be available at the bedside.
• Note that the diagnosis of respiratory failure from airway obstruction is a clinical diagnosis and can be present despite normal arterial blood gases and pulse oximetry. Hypoxemia and hypercarbia will only be late signs of airway obstruction, and intubation should be accomplished before these develop.
• Monitor for evidence of inhalation injury, including singed nasal hairs, excessive secretions, progressive respiratory distress; report these findings to the on-call provider immediately.
• Provide oxygen therapy as needed and monitor the effect on systemic oxygenation, including pulse oximetry and arterial blood gases.
• Perform tracheal suctioning as needed to maintain a clear upper airway.
• Encourage the alert patient (as age-appropriate) to take deep breaths and cough as needed to clear the airway.
• Insert oral or nasal airway as needed (and ordered by on-call provider).
• Position child to maintain airway patency (particularly important if level of consciousness is impaired).
• Assess patient responsiveness; discuss elective intubation if the patient is obtunded or demonstrates decreased response to stimulation
Hypoxemia, Hypoxia and Impaired Gas Exchange Related to: Airway Obstruction, Inhalation Injury, Pulmonary Edema, Acute Respiratory Distress Syndrome, Carbon Monoxide Poisoning, Impaired Level of Consciousness
Expected Patient Outcomes
• Patient will demonstrate adequate respiratory function as evidenced by: appropriate respiratory rate, depth and effort; patent airway; normal arterial blood gases; COHb level less than 10%; absence of crackles or pulmonary edema on chest radiograph.
• If patient demonstrates hypoxemia despite oxygen therapy, or ineffective ventilation, support of airway, oxygenation, and ventilation will be provided,
• Patient will remain alert and oriented with adequate respiratory rate and effort.
Nursing Activities
• Monitor respiratory rate and effort, and notify a provider of development of respiratory distress.
• Monitor pulse oximetry, exhaled carbon dioxide (PETCO2), and arterial blood gases; notify a provider of any development of hypoxemia or hypercarbia.
• Monitor for evidence of inhalation injury, including headache, dizziness, confusion, flushed appearance (late finding), visual disturbance, seizures, and metabolic acidosis. Report these findings to the on-call provider immediately.


• Administer oxygen as ordered, and monitor the patient’s response.
• Be prepared to assist with non-invasive positive-pressure ventilation, hyperbaric oxygen therapy or intubation and mechanical ventilation as needed. Ensure that emergency equipment is readily available.
Pain Related to Burn, Multiple Invasive or Painful Catheters, and Painful Dressing Changes and Procedures
Expected Patient Outcomes
• Patient will demonstrate no pain, as evidenced by verbal reports of pain (as age-appropriate), facial expression, crying or whining, and increased heart rate and blood pressure.
• Patient will demonstrate relaxed body position, relaxed facial expression, ability to sleep when undisturbed, and appropriate heart rate and blood pressure for age and clinical condition.
• Patient will indicate reduction or elimination of pain as indicated and quantified using consistent pain assessment tools (see Chapter 5).
Nursing Activities
• Assess nature, location, quality, and intensity of pain every hour and as needed using consistent pain assessment tool (see Chapter 5).
• Monitor patient closely for nonverbal evidence of pain, including: restlessness, guarding of burned areas, wrinkled brow, clenched fingers, reluctance to move, facial pallor or flushing, diaphoresis, tachycardia or hypertension, pupil dilation.
• Assess analgesic administration and discuss modification of analgesic dose, schedule, or type with a physician or other on-call provider if pain persists.
• Administer analgesics as ordered and evaluate effectiveness; if continuous infusion medications are ordered, ensure that bolus administration of the drug is used to initiate infusion therapy and achieve therapeutic drug levels. Bolus therapy may also be required if dose of drug is increased (see Chapter 5). Ensure adequate analgesia before painful procedures.
• Identify factors that aggravate or alleviate pain and modify patient support accordingly.
Potential Burn Wound Infection or Septic Shock Related to: Open Wound, Presence of Multiple Invasive Catheters, Compromise in Immune Function
Expected Patient Outcomes
• Patient will demonstrate no signs of infection, including fever or hypothermia, leukocytosis or leukopenia, wound erythema, wound drainage, or positive blood cultures.
• If infection develops, it will be promptly treated with antibiotics and the patient will be closely monitored for evidence of sepsis.
• Burn wound and graft sites will heal within appropriate interval.
Nursing Activities
• Assess wound appearance for evidence of local signs of infection, including: erythema; change in color, appearance, odor, or amount of wound drainage; change in color of wound to black or dark brown, progression of burn to full thickness injury; sloughing of graft or wound breakdown after closure.
• Monitor patient temperature and white blood cell count and differential; notify the on-call provider of changes.
• Obtain blood cultures as ordered.
• Administer antibiotics as ordered (precisely on time) and monitor for side effects.
• Ensure strict aseptic technique during all invasive procedures; ensure clean technique during all noninvasive procedures including wound care.
• Maintain closed delivery system for all intravenous lines.
• Ensure good hand-washing technique by all members of the healthcare team before and after patient contact.
• Monitor the patient closely for signs of sepsis, including fever, hypothermia, tachycardia, tachypnea, alteration in responsiveness, leukocytosis, leukopenia, metabolic acidosis, evidence of organ system dysfunction (e.g., oliguria, diarrhea or vomiting, abdominal distension, elevation in liver transaminases), evidence of relative hypovolemia (and increased intravascular fluid requirements to maintain perfusion), and signs of septic shock (sepsis plus cardiovascular and one other organ system failure). See Boxes 6-2 and 6-3.
Potential Temperature Instability Related to Heat Loss Through Burn Surface
Nursing Activities
• Monitor body temperature hourly initially, then every 2 to 4 hours when the patient’s condition is stable.
• Monitor for signs of cold stress, including shivering or chills, or tachycardia, tachypnea and hypoxemia in young infant.
• Use a heat shield, over bed warmer, or warming blanket as needed to maintain the patient’s body temperature.
• Keep ambient air warm, and prevent any room draft.
• Use warmed (not hot) solution during burn care.
• Perform burn care under a heat shield or overbed warmer for infants or young children and as needed for older children.
• Minimize patient exposure during treatments or wound care (e.g., perform burn care on one extremity at a time, and redress that extremity before the next extremity is unwrapped).
• Consider warming of intravenous fluids and blood products; warming should be performed according to hospital policy for infants and young children.
Potential for Inadequate Nutrition Related to: Excessive Caloric Requirements, Inadequate Caloric Intake, Altered Metabolism
Nursing Activities
• Calculate the patient’s caloric and protein needs and notify the on-call provider if these are not being met. Monitor patient tolerance of tube, oral, or parenteral feedings. Ensure appropriate distribution of calories within protein, fat, and carbohydrates.
• Begin enteral feedings as ordered; notify a provider of feeding intolerance (including abdominal distension, vomiting, diarrhea, increased residual volume after gastric feeding, reflux). For additional information, see Chapter 14.
• Implement measures to reduce nausea and vomiting as needed, including position changes and administering an antiemetic.
• Ensure adequate oral intake when tolerated; calculate caloric intake and ensure provision of multiple opportunities to eat a wide variety of appetizing foods.
• Obtain daily weight; notify a provider of weight loss or failure to gain weight.
• Obtain consultation with dietician as needed. Evaluate oxygen and caloric expenditure using calorimetry as needed.
Inadequate Intravascular Volume and Cardiac Output: Third-Spacing Phase
Etiology and Pathophysiology
The magnitude of the intravascular volume deficit will depend on the depth and surface area of the injury and the time elapsed before adequate fluid resuscitation.28 In general, deeper and larger (15% or more of TBSA) burns are associated with more significant fluid shifts and circulatory complications.
Clinical Signs and Symptoms
After a significant burn, intravascular volume loss will eventually produce signs of hypovolemia (Box 20-3). Children often do not exhibit significant signs of hypovolemia, including hypotension until more than 25% of the circulating volume is depleted and complete cardiovascular collapse is imminent.
Significant hypovolemia will compromise systemic perfusion and may produce shock. Such hypovolemia will produce tachycardia, prolonged capillary refill time, and cold extremities. Anuria is often present. The development of a metabolic acidosis (i.e., fall in arterial pH, rise in serum lactate) indicates critical compromise in tissue perfusion. The young infant in shock often will demonstrate temperature instability and hypoglycemia. Hypotension may develop only as a late sign of shock.47
Management
Intravenous Access and Monitoring
Whenever a major burn is present or systemic perfusion is compromised significantly, insertion of a central venous catheter (into the subclavian, internal jugular, or femoral vein) should be considered to provide reliable, large-bore venous access. Use of a multilumen central venous catheter will provide two or more ports to facilitate fluid administration and central venous pressure (CVP) monitoring. Any central venous catheter must be inserted under strict sterile conditions and cared for using strict aseptic technique (see Chapter 21 and Box 22-6).
Determination of Fluid Requirements
A variety of formulas have been developed to assist in determining fluid losses and requirements in patients with burns (Table 20-3). Many formulas, however, have been designed for use in adult patients and are based solely on body weight and percentage of TBSA burned. Use of these adult formulas will result in inadequate pediatric fluid resuscitation.62,135
The most popular formula for use in adolescent and adult patients with burns is the Parkland (by Baxter), formula.10 Modification of the Parkland formula for children provides for crystalloid administration during the first 24 hours of therapy. The volume administered during this time is based on the burn surface area (4 mL/kg per percent of TBSA burned) plus maintenance fluid requirements (1500 mL/m2 BSA).215 Half of this calculated fluid is administered during the first 8 hours of therapy, and the remaining half is administered during the next 16 hours of therapy.
The child’s fluid resuscitation requirements should be based on body surface area rather than weight. Because children have a greater body surface area in relation to weight, weight-based formulas can underestimate the fluid requirements of children with minor burns and may grossly overestimate the fluid requirements of those with extensive burns.79 TBSA can be rapidly estimated from height and weight using standard nomograms (see inside back cover of this text).
The Galveston formula26 (developed by Carvajal at the Shriners Hospital for Children in Galveston, Texas) provides 5000 mL/m2 BSA burned plus 2000 mL/m2 BSA of lactated Ringer’s solution given over the first 24 hours after the injury, with half the volume administered during the first 8 hours and the remaining half over the next 16 hours. The Carvajal formula26 recommends crystalloid and colloid administration based on the absolute surface area of the child’s burn, plus generous maintenance fluid administration.
Selection of Fluid Content
There is continued debate regarding the relative benefits of crystalloid versus colloid administration during burn resuscitation.27,42,51,176 Proponents of crystalloids advocate the use of isotonic or hypertonic crystalloids because they are physiologic, inexpensive, and readily available.
Critics of crystalloid administration note that immediately after administration, isotonic crystalloids will equilibrate between the intravascular and interstitial spaces, and only a fraction of administered intravenous crystalloids will remain in the vascular space.178 Therefore, large quantities of crystalloids generally are required to restore intravascular volume. In addition, the fluid that moves into the interstitial space may contribute to worsening systemic edema. Pulmonary interstitial water usually does not increase substantially during this time, because pulmonary capillary permeability remains normal unless significant inhalation injury occurs. In addition, lymph flow is usually proportional to the amount of pulmonary interstitial water movement.
Colloid resuscitation may restore intravascular volume and pressure more efficiently than will crystalloid administration. If capillary permeability is normal, administered colloids will remain in the vascular space for several hours, exerting oncotic pressure. This oncotic pressure will increase intravascular volume and maintain intravascular osmolality, so that continued fluid shift from the vascular space is less likely. Because colloids are thought to diffuse more slowly into the interstitial space, colloid resuscitated patients may develop less edema than crystalloid-resuscitated patients.163 Adequate fluid resuscitation should be possible with relatively small volumes of colloids,86,201 so that the patient receives a small volume and salt load.
Critics of colloid administration note that membrane permeability is not normal in patients immediately after burns, and proteins may move from the vascular to the interstitial space during the first 24 hours after a burn.9 Movement of administered colloids into the interstitial space can increase interstitial oncotic pressure, enhancing the fluid shift from the intravascular space into the interstitial space.
Colloid administration during the first day after a burn was avoided in the past, based on the fear that it would increase the severity of third-spacing of fluid.182 However, the validity of this criticism has been challenged during the last decade. Although albumin may leave the vascular space, an equal amount of albumin may be returned to the vascular space by lymphatics approximately 8 hours or more after a burn. Therefore many institutions have successfully added small amounts of colloids to their early burn resuscitation protocols.
In general, adequate resuscitation can be provided if isotonic crystalloids are administered in sufficient quantity.48 Lactated Ringer’s (LR) solution, an isotonic crystalloid, is the most widely used solution for burn resuscitation. The composition of LR’s solution closely mimics extracellular (including intravascular) fluid composition (Table 20-4); therefore LR’s solution is ideal for replenishing intravascular water and electrolytes. In addition, LR’s solution contains lactate, which is metabolized to bicarbonate, so it will buffer mild acidosis. Lactated Ringer’s solution is inexpensive, readily available, and effective in the treatment of nonhemorrhagic hypovolemia.
During fluid resuscitation, the child’s systemic perfusion and urine output must be monitored closely. These parameters should improve if fluid administration is adequate (Table 20-5). The serum hemoglobin concentration, electrolyte balance, and acid-base status (including serum lactate) must also be monitored closely.
Table 20-5 Clinical Responses to Fluid Resuscitation in Burned Patients
Parameter | Desirable Response (Fluid Resuscitation Adequate) | Undesirable Response (Fluid Administration Inadequate) |
Urine output | 1 mL/kg per hour (up to 30 kg, then 25-30 mL/h) | <1 mL/kg per hour (for children above 30 kg, less than 25 mL/h) |
Specific gravity | 1.010-1.025 | >1.025 |
Weight | Preburn level | 10% less than preburn level |
Blood pressure | Normal for age or high* | Low for age* |
Pulse | Normal for age* | Normal or high* |
Level of consciousness | Alert, clear, and lucid | Lethargic and stuporous |
Hematocrit | 35%-45% | 48%-55% |
Serum sodium | 135-145 mEq/L | >150 mEq/L |
Blood urea nitrogen | 5-20 mg/dL | >25 mg/dL |
Creatinine | 0.8-1.4 mg/dL | >2.0 mg/dL |
Osmolality (serum) | 275-295 mOsm/L | >300 mOsm/L |
Urine sodium | 60-100 mEq/L | ≤40 mEq/L |
Blood pH | 7.20-7.50 | <7.20 |
Serum lactate | Venous: 0.5-2.2 mmol/L Arterial: 0.5-1.6 mmol/L |
>4 mmol/L |
Peripheral circulation | Brisk capillary refill; normal color in unburned areas | Cyanosis; prolonged capillary refill |
Central venous pressure (CVP) | 4-8 mmHg | <2-4 mmHg |
Pulmonary artery pressure (PAP) | Systolic, 20-30 mmHg | Systolic, <20 mmHg |
Diastolic, 5-15 mmHg | Diastolic, <5 mmHg | |
Cardiac index | 3.0-4.5 L/min per m2 BSA | <3.0 L/min per m2 BSA |
BSA, Body surface area.
* See normal blood pressure and heart rate ranges for age in Tables 1-1 and 1-3 (and on pages inside front cover).
Hypertonic saline resuscitation can be beneficial in treating burn-induced shock.16,17,64,84 This process maintains intravascular volume more effectively because it induces movement of free water from the interstitial to the intravascular space, thus decreasing generalized tissue edema. However, hypertonic saline is not widely used because of the potential risk of hypernatremia, hyperosmolarity, renal failure, and alkalosis.98,173,222 Some favor the use of a modified hypertonic solution—adding an ampule of sodium bicarbonate to each liter of lactated Ringer’s solution during the first 24 hours of resuscitation.19
Routine Care
Regardless of the type of resuscitation fluid being used, the nurse must closely monitor the patient’s response to volume resuscitation (see Table 20-5). Adequate systemic perfusion, demonstrated by warm extremities, brisk capillary refill, strong peripheral pulses, and adequate (1-2 mL/kg body weight per hour) urine volume should be observed.
The child’s level of consciousness should be appropriate for clinical condition. Irritability may be an early sign of cardiovascular or neurologic deterioration,150 and lethargy or decreased response to painful stimulation is abnormal and requires investigation.
During fluid resuscitation, the nurse should be alert for the development of pulmonary edema, and the team should have a plan for a sequence of appropriate respiratory support. Elective intubation should be performed before decompensation occurs (see Respiratory Failure in this chapter and Chapter 9).
The urine sodium may also be monitored. Normal urinary excretion of sodium is approximately 60 to 100 mEq/L. A low urine sodium (less than 40 mEq/L) usually results from aldosterone secretion in the presence of inadequate intravascular volume123 and indicates the need for further volume administration.
Evaluation of Therapy
Increased fluid administration is probably necessary if inadequate systemic perfusion and continuing acidosis are associated with a low CVP. Vasoactive drug therapy will not improve systemic perfusion produced by hypovolemia. Poor systemic perfusion and extreme acidosis despite adequate fluid administration indicate severe shock and are associated with a high mortality (see Shock in Chapter 6).