12 Cardiac Surgery and Transplantation
After reading this chapter, you should be able to:
• outline cardiac surgical procedures including coronary artery bypass graft surgery and valve repair and replacement
• describe the indications, advantages and disadvantages of using cardiopulmonary bypass
• outline methods of myocardial preservation during cardiac surgery
• outline immediate postoperative management of cardiac surgical patients including haemodynamic, rhythm monitoring, ventilatory support, postoperative bleeding including pericardial tamponade, postoperative pain, fluid and electrolyte and emotional and family support
• outline the principles of counterpulsation in intra-aortic balloon pumping
• outline the benefits and timing of balloon inflation and deflation, conventional and real timing, management and assessment of timing and timing errors
• describe the nursing management of IABP complications, including limb perfusion, bleeding and immobility-related complications
• discuss methods of weaning IABP and management of IABP removal
• discuss the immediate postoperative care of heart transplant recipients
• describe the clinical manifestations of postoperative complications in heart transplant recipients
• identify signs and symptoms of rejection in heart transplant recipients
• evaluate the effectiveness of nursing interventions in the postoperative management of heart transplant recipients.
Introduction
Many critically ill patients experience compromised cardiac function, as either a primary or secondary condition. This chapter follows on from those situations examined in Chapter 10, and reviews patients with conditions that tend to be cared for in specialised critical care units. In this chapter, the management of a patient who requires cardiac surgery for coronary artery disease or valvular disease will be discussed, including the use of cardiopulmonary bypass. In addition, the use and management of intra-aortic balloon pumping in cardiac surgical and medical patients will be outlined. The management of patients following heart transplantation will be identified including the immediate postoperative complications and their prevention and management.
Cardiac Surgery
Structural Abnormalities
Some structural abnormalities result from myocardial infarction, and have been described in Chapter 10. Other structural abnormalities result in valvular disease (mitral, aortic, tricuspid, pulmonic) and ventricular defects.
Valvular Disease
The incidence and types of valvular disease have changed over the past 50 years.1 Valvular disorders, such as mitral stenosis and aortic regurgitation, often arise from infectious diseases like rheumatic fever and syphilis, which are much less common today. Conversely, there has been a rise in the rate of aortic stenosis, which is due to degenerative disease common in ageing. In contrast to these trends, the prevalence of rheumatic fever and rheumatic heart disease among Indigenous Australians is one of the highest in the world.2 Also, Pacific Islanders living in New Zealand have much higher rates of rheumatic fever than the general population.3 As a result, valvular disorders are much more common in these groups. Rheumatic fever is discussed under infective endocarditis in Chapter 10.
Stenotic valves have a tightened, restricted orifice, so that blood must be forced through at higher pressure (Figure 12.1). In regurgitation, also called valvular incompetence or insufficiency, incomplete closure of the valve leaflets results in backflow of blood. Valvular conditions can result from congenital deformities, but also from the degenerative changes associated with ageing, from infection and rheumatic diseases. When a valve is stenosed, higher pressure is required to push blood through the narrow opening and the heart compensates by hypertrophy and dilation. When a valve is incompetent the heart does not empty sufficiently, so again the heart compensates by hypertrophy and dilation. In both these conditions heart failure may result; however, in regurgitation, pressure in the ventricles and atrium grows and this pressure is reflected back into the pulmonary or venous system. Although the heart contains four valves, the majority of disorders affect the mitral and aortic valves in the left side of the heart.
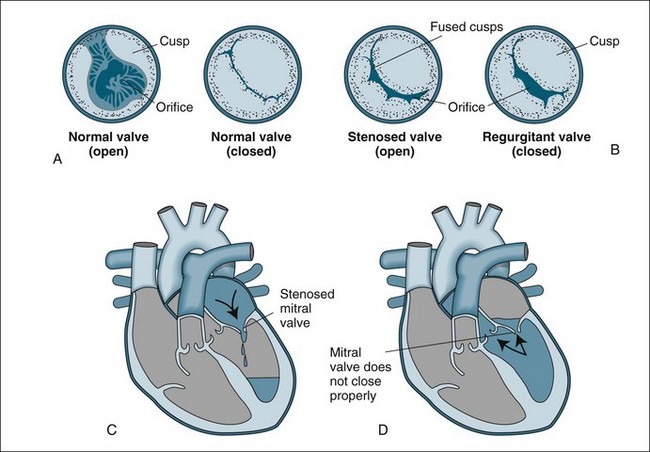
FIGURE 12.1 Valvular stenosis and regurgitation: (A) normal position of valve leaflets (cusps) when the valve is open and closed; (B) open position of a stenosed valve (left) and closed position of a regurgitant valve (right); (C) haemodynamic effect of mitral stenosis shows the mitral valve is unable to open completely during left atrial systole, limiting left ventricular filling; (D) haemodynamic effect of mitral regurgitation shows the mitral valve does not close completely during left ventricular systole, allowing blood to re-enter the left atrium.43
Aortic valve disease
Aortic stenosis is a narrowing of the opening of the valve between the left ventricle and aorta (Figure 12.1). This stenosis often results from degenerative changes that occur with age or as a result of congenital abnormalities such as a bicuspid aortic valve (prevalence of bicuspid aortic valve in the general population is 0.5% and may cause aortic stenosis or regurgitation). Aortic stenosis is usually associated with left ventricular hypertrophy in response to the high pressure needed to push blood into the aorta. Increased myocardial oxygen demands from the hypertrophied muscle also mean that angina is common. Often the first sign of aortic stenosis is left heart failure, which is a culmination of these two effects and adaptive dilation.4 On auscultation, additional heart sounds are heard as a systolic murmur and a loud S4.
Aortic regurgitation may occur acutely when the aortic valve is damaged by endocarditis, trauma or aortic dissection, and presents as a life-threatening emergency. Chronic aortic regurgitation usually results from rheumatic heart disease, syphilis, chronic rheumatic conditions or congenital conditions. Again the left ventricle compensates by hypertrophy and dilation, which ultimately can result in left heart failure. When left heart failure occurs, left atrial pressure rises and may cause pulmonary hypertension. In the acute situation, the patient presents with collapse, severe hypotension and dyspnoea.4 Patients with chronic regurgitation may remain asymptomatic for years, finally presenting with signs of left heart failure. On auscultation, a diastolic murmur can be heard.
Mitral valve disease
Mitral valve stenosis often occurs as a result of rheumatic heart disease and less often from systemic lupus erythromatosus. These diseases cause damage to the leaflets and chordae tendineae, so that during healing the scars contract and seal, restricting the aperture. Left atrial pressure rises with resultant pulmonary hypertension. In chronic conditions, this pressure may also affect the right ventricle.5 Lung compliance is also reduced, causing dyspnoea. On auscultation a low-pitched diastolic murmur and an opening snap can be heard.
Mitral valve regurgitation results when the mitral valve and chordae tendineae are damaged, often due to myocardial infarction, rheumatic disease and infectious endocarditis. Backflow into the left atrium during systole creates elevated atrial and pulmonary pressures, and pulmonary oedema can result.5 On auscultation, a third heart sound and a pansystolic murmur can be heard.
Surgical Procedures
Coronary Artery Bypass Graft Surgery
CABG uses a section of vein or artery to bypass a blockage in the patient’s coronary artery. The vessels used for grafting arise from the internal mammary artery, or are taken from the saphenous vein or radial artery. Saphenous veins are removed from the legs, and the radial artery from the forearm and used as a free graft with anastomoses at the ascending aorta and distally to one or more coronary arteries. When saphenous veins are used as grafts (SVG), they often develop diffuse intimal hyperplasia, which ultimately contributes to restenosis. Patency rates are lowest in saphenous vein grafts attached to small coronary arteries or coronary arteries supplying myocardial scars. Consequently, arterial grafts are used more often, as they are more resistant to intimal hyperplasia. Internal mammary arteries (IMAs) and radial artery grafts may be used.6 The IMA remains attached to the subclavian artery and is mobilised from the chest wall and anastomosed to the coronary artery distal to the occlusion (Figure 12.2). If the radial artery is being harvested for grafting, the collateral circulation in the forearm is assessed. Echo colour Doppler provides best accuracy of forearm circulation, although the clinical Allen test is quite commonly used. The disadvantage of the Allen test is that it has around 5% false patency result.7 A selection of IMA, SVG and radial artery grafts may be necessary over time as repeat procedures are needed or in patients with extensive disease requiring multiple grafts.
Over recent years a new approach to CABG – minimally invasive direct coronary artery bypass grafting (MIDCABG) – has been used. This procedure uses intercostal incisions and a thorascope instead of a sternotomy to access the heart and coronary arteries. MIDCABG is also often performed without cardiopulmonary bypass (off pump coronary artery bypass, OPCAB); instead, the heart is slowed with beta-blockers to allow the surgery to be performed on a beating heart.8 OPCAB procedures may also be performed using full or partial sternotomy to provide access for multiple vessels grafting. Both procedures have been successful responses to the drive to reduce recovery times, patient stays in hospital and costs.8 MIDCABG is currently only used in single-vessel disease, particularly the left anterior descending (LAD) artery. More recently, robotically-assisted cardiac surgery has been performed in America and Europe and has been introduced at a small number of Australian hospitals for CABG and mitral valve surgery. This technique has further reduced the invasiveness of cardiac surgery, as little more than stab wounds are required in the right chest for thoroscopy and the robotic instruments. Avoiding true thoracotomy or sternotomy improves postoperative pain experiences and shortens length of stay.9
Although CABG is the most common cardiac surgical procedure undertaken in Australia, the incidence has declined since 2005/06 to be 61 procedures/100,000 population in 2007–08.10 The decline in surgery rates is due to changes in the treatment of CHD, including the advent of percutaneous coronary intervention (PCI). More procedures are now being performed in older patients, with 73% of current patients aged over 60 years.1 CABG is used to relieve the symptoms of angina by increasing coronary blood flow distal to occlusive coronary lesions. It is a palliative, not curative, treatment as the underlying disease process continues.11 CABG is more effective than PTCA in patients with extensive, multi-vessel disease.9,11 CABG is also used in left main vessel lesions due to the high risk of extensive infarction associated with PTCA in this area. Women do not appear to have the same access to CABG surgery, as men are three times more likely to have surgery, although only twice as likely as women to have CHD.12 CABG surgery is commonplace, and many cardiothoracic centres have highly efficient, effective systems in place with mortality rates as low as 2%.
Valve Repair and Replacement
Valve surgery is usually undertaken to repair the patient’s valve or, more often, to replace the valve with either a mechanical or tissue prosthesis. The clinical decision for valve surgery is primarily based on the clinical state of the patient using the New York Heart Association (NYHA) classification system and echocardiographic findings.5 The type of surgery used will depend on the valves involved, the valvular pathology, the severity of the condition and the patient’s clinical condition. Often valve surgery is not a single procedure, and it may involve multiple valves, CABG and implantable cardioverter defribillator (ICD). Valve surgery is palliative, not curative, and patients will require lifelong health care.
Valve replacement may be necessary, but could be associated with higher risks due to long-term disease process and poor underlying left ventricular function. The most common indication for valve replacement is aortic stenosis, and accounts for 60–70% of valve surgery.13 Prosthetic valves may be mechanical or biological. Mechanical valves are made of metal alloys, pyrolite carbon and Dacron (Figure 12.3). Biological valves are constructed from porcine, bovine or human cardiac tissue. Mechanical valves are more durable but have an increased risk of thromboembolism, so lifelong anticoagulation is required. Biological valves suffer from the same problems as the patient’s valve (i.e. calcification and degeneration). The choice of valve depends on the age of the patient and potential difficulties with taking anticoagulants.
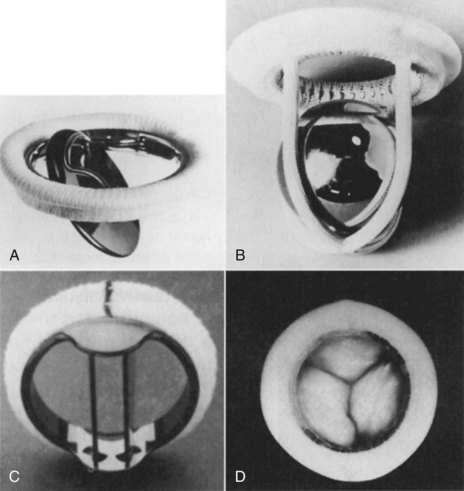
FIGURE 12.3 Prosthetic valves: (A) the Bjork-Shiley valve, with a pyrolyte-carbon disc that opens to 60 degrees; (B) the Starr-Edwards caged-ball valve model 6320, with satellite ball; (C) the St Jude Medical mechanical heart valve, with a mechano-central flow disc; (D) the Hancock II porcine aortic valve, with stent and sewing ring covered in Dacron cloth.5
Mortality for valvular surgery is higher than for CABG, reflecting the underlying loss of ventricular function and additional procedures that are common. Risk stratification models have been developed to help determine the patients that are most likely to have poor recovery and outcomes.14 The major factors that contribute to poor outcomes are worse left ventricular function and age over 70 years old.
Cardiopulmonary Bypass
Adverse effects of CPB are diverse, and include the following:15
• Haematological effects due to exposure of the blood to tubing and gas exchange surfaces, which initiates surface activation of the clotting cycle. Also blood component damage due to shear stress from the roller action of the pump, which reduces haematocrit, leucocyte and platelet counts.
• Pulmonary effects due to activation of systemic inflammatory response syndrome (SIRS) that increases capillary leakage, and lung deflation during surgery leading to post-operative atelectasis.
• Cardiovascular effects due to volume changes, fluid shifts and decreased myocardial contractility, which decreases cardiac output. This is most severe during the first 6 hours, but usually resolves within 48–72 hours.
• Neurological effects due to poor cerebral perfusion and generation of thromboemboli from aortic cannulation, which can lead to cerebrovascular accidents.
• Renal effects due to decreases in cardiac output during initiation of CPB, which decreases renal perfusion.
• Post-pump delirium or psychosis, which occurs in 32% of CPB patients although the cause has not been identified. Symptoms include short-term memory deficit, decreased attention, and inability to respond to and integrate sensory information.
• Activation of a systemic inflammatory response, which may cause vasodilation and increased cardiac output.
Nursing Management
The Immediate Postoperative Period
Patients should be transported to intensive care accompanied by at least an anaesthetist, an appropriately qualified nurse and transport personnel under continuous cardiac monitoring and assisted ventilation. It is prudent to include capnography during patient transport to detect ventilator disconnection, dysfunction, or endotracheal tube migration. Intensive care or theatre nursing staff may be a component of the transport team. The admission to intensive care requires a team approach, with the participation of intensive care nursing and medical staff and/or technician input. The immediate postoperative decision making on patient management is influenced by handover from anaesthetists, settling in procedures and collegial assistance.16 Admission activities are commonly divided between nurses, with one nurse taking responsibility for establishing monitoring and haemodynamic assessment and management, and a second nurse managing ventilation and endotracheal tube security, as well as managing chest drains, gastric tube and urinary catheter. If staffing permits, additional nurses may take responsibility for documentation, performing arterial blood gases, 12-lead ECG and providing assistance as required.
The objectives of immediate post operative management of cardiac surgical patients may include:
Haemodynamic Monitoring and Support
Typical haemodynamic monitoring includes an intra-arterial catheter for continuous blood pressure monitoring and arterial blood sampling. Cardiac output and preload measurement are achieved most commonly with either a pulmonary artery or central venous catheter configured for pulse contour cardiac output (PiCCO) monitoring (see Chapter 9).
Preload measures provided by the pulmonary artery catheter include right atrial pressure (RAP) to approximate right ventricular filling, and pulmonary artery pressure (PAP) to approximate right ventricular systole and provide insight into pulmonary vascular resistance and left heart function. The pulmonary capillary wedge pressure (PCWP) is available to approximate left ventricular filling and left heart function. Alternatively, the PiCCO monitoring system represents preload by intrathoracic blood volume index (ITBVI) and global end-diastolic volume index (GEDVI). In addition, the extravascular lung water index (EVLWI) can demonstrate the accumulation of interstitial lung water.17
Certain common haemodynamic patterns are seen in the early postoperative phase. These must be detected through thorough monitoring and interpretation of variables, and managed according to specific needs. During the initial two hours of recovery period, 95% of patients will experience haemodynamic instability.18
Hypertension
Hypertension is present in up to 30% of patients initially,19 as hypothermia, stress responses, pain and hypovolaemia contribute to vasoconstriction.19–21 When the systemic vascular resistance is excessive, the high afterload may contribute to low cardiac output.19 Rewarming to normothermia with space blankets or heated air blankets, fluid administration, administration of sedation or analgesics, and infusion of IV vasodilators (glyceryl trinitrate or sodium nitroprusside) are all commonly used to overcome vasoconstriction when contribut-ing to hypertension.19–21 Occasionally beta-blockers are used. Hypertension increases myocardial workload and contributes to bleeding.
Hypotension
Transient hypotension requiring treatment is common at some stage during the postoperative period. Contributing factors to hypotension include hypovolaemia and decreased venous return (from polyuria, bleeding, ventilation and positive end-expiratory pressure, and excess vasodilation), contractile impairment (from ischaemia or infarction, hypothermia, and negative inotropic influences), pericardial tamponade, and vasodilation (from excess vasodilator therapy, or as part of an inflammatory response to cardiopulmonary bypass).22
Hypotension may present with reduced or elevated preload, reduced or elevated cardiac output, and reduced or elevated systemic vascular resistance (SVR). When hypovolaemia is present, cardiac output will be low and SVR usually high. Hypovolaemia is diagnosed by measuring preload indicators, as pressure (RAP, PAP, PCWP) or volume (ITBVI, GEDVI).17,19 Colloids (e.g. normal serum albumin) are generally preferred for volume restoration in the postoperative period.18 Blood returned from the cardiopulmonary bypass circuit (‘pump blood’) usually accompanies the patient to ICU, and this should be readministered at a rate suitable to filling indices and blood pressure.
Hypotension accompanied by elevated preload and low cardiac output usually represents cardiac dysfunction or pericardial tamponade, and the distinction should be quickly sought.20,23 When such left ventricular dysfunction is present, there is usually compensatory vasoconstriction and tachycardia, although heart rate responses may be unreliable due to cardioplegia, cold, conduction disease21 and preoperative beta-blocking agents. Inotropic agents, including milrinone hydrochloride, adrenaline, dopamine or dobutamine, may become necessary (these are covered more completely in Table 20.7 and its accompanying text). When the profile of severe left ventricular dysfunction is persistent (either at the time of coming off bypass or later in intensive care), intra-aortic balloon pumping may be instituted. ECG assessment for new ischaemia or infarction should be made, which if of significant size, may warrant surgical re-exploration or angiographic investigation. Pericardial tamponade is also a cause of hypotension (covered later in this chapter).
A fourth common postoperative profile is hypotension with normal or elevated cardiac output in the presence of low SVR. This may occur with excess vasodilator administration, the use of postoperative epidural infusions, and vasodilation from a systemic inflammatory response to cardiopulmonary bypass and other factors such as reinfusion of collected operative site blood.24 The inotrope milrinone hydrochloride is popular in the postoperative phase because of its dilating effect on radial artery grafts,25 but often contributes to hypotension through its systemic vasodilator properties. When hypotension is attributable to vasodilation, metaraminol or noradrenaline may be used.19 Arginine vasopressin, by infusion, has more recently emerged as an effective alternative vasoconstrictor for cardiac surgical patients.26
A mean arterial pressure of 70–80 mmHg is generally targeted in the postoperative period.21 This can sometimes be reduced if there has been ventriculotomy or if there is concern about the status of the aorta.20 The cardiac index should be maintained above 2.2 L/min/m2, as hypoperfusion develops below these values. When at these levels, additional assessments are often undertaken, such as mixed venous oxygen saturation measurement (to assess oxygen delivery deficits) and arterial pH and lactate measurements (to detect metabolic acidosis from anaerobic metabolism).
In addition to assessment of preload, contractility and afterload, heart rate and rhythm should be assessed for their input into cardiac output and blood pressure. Extremes of rate and arrhythmias alter ventricular filling and may need correction. If temporary pacing wires are present, pacing strategies for haemodynamic improvement include rate rises (even if already in the normal range)21 and the provision of dual-chamber or atrial pacing as alternatives to just ventricular pacing. Alternatively, if ventricular pacing is present, reducing the rate to permit expression of a slower sinus rhythm may, with the provision of atrial kick, improve cardiac output and blood pressure (refer to Chapter 11 for more information on pacing).
Rhythm monitoring and postoperative arrhythmias
Continuous rhythm monitoring is necessary while in intensive care, and telemetry monitoring is usually continued until discharge from hospital. Lead selection is often haphazard, but a chest lead in the V1 position (or lead MCL1) generally provides best information on atrial and ventricular activity.27 Unlike many leads, these two leads reliably demonstrate normal rhythms, bundle branch block and ventricular rhythms,27 and may be useful in confirming pulmonary artery catheter irritation as the cause of ventricular arrhythmias.28
A 12-lead ECG is performed on admission to the ICU and should be compared with the preoperative ECG. It should be assessed for signs of new ischaemia or infarction, new bundle branch block and arrhythmias or conduction disturbances. Pericarditis, a frequent complication of surgery, appears as ST segment elevation (often, but not always, in many leads), and may mask or mimic myocardial infarction. The nurse should look for the classic concave upward, or ‘saddle-shaped’ ST segment, to distinguish pericardial changes from the more convex upward ST segment of infarction. Worsening of pain on inspiration and a pericardial rub help to confirm pericarditis.27
Atrial fibrillation is the most common postoperative arrhythmia and contributes significantly to postoperative morbidity and hospital length of stay.29 It occurs in up to 30–50% of patients, most often on days 2 to 3 postoperatively.15,29 Many patients revert without treatment,19 but when treatment becomes necessary beta-blockers and amiodarone appear the most successful agents for correction.29 Digitalis is effective for rate control and IV magnesium is often used, although further evidence for its use is needed. Atrial pacing to prevent atrial fibrillation is being increasingly explored but a clear recommendation on pacing sites and protocols has yet to emerge. By contrast, atrial overdrive pacing can be an effective means to immediately and safely interrupt atrial flutter.29
Ventricular ectopic beats are common and by themselves do not require treatment unless they accompany ischaemia or biochemical disturbance,19 in which case they may progress to more complex arrhythmias. Consideration should always be given to the pulmonary artery catheter as the cause (including both correctly and malpositioned catheters),28 as this is an easily corrected influence. Ventricular tachycardia and fibrillation are uncommon and usually denote myocardial disturbance such as ischaemia or infarction, shock, electrolyte disturbance, hypoxia, or increased excitation by high circulating catecholamine levels.17 Standard approaches to resuscitation according to protocols in Chapter 24 apply, including standard CPR over the recent sternotomy. When ventricular fibrillation cannot be corrected, consideration is often given to re-exploration of the chest to examine graft patency and/or provide internal cardiac massage. The cardiac surgical intensive care unit should be equipped to enable emergency re-exploration for such purposes.
Ventilatory Support
Ventilation should be approached according to the general principles described in Chapter 15. As anaesthesia is not typically reversed at the end of the operation, patients are generally admitted apnoeic, and within 1–3 hours return to wakefulness and spontaneous breathing.
Ensuring a secure airway is an initial priority; the following should be undertaken:
• Confirmation of endotracheal tube position and its security immediately on admission:
There has been a general trend to more rapid ventilatory weaning in recent years, and in some centres ‘fast-track’ cardiac surgical recovery includes extubation at the end of the operation before transfer to a recovery unit for suitable patients. Indices of respiration show no improvement when intubation is maintained for longer compared with early extubation,30 and pooled results from randomised early extubation trials show earlier ICU discharge and shorter lengths of stay (by 1 day) when early extubation is undertaken.31
• intraoperative neurological event
• gas exchange deficit with unresolved hypoxaemia
• significant haemodynamic insufficiency
• patients returning from theatre late in the evening may sometimes continue ventilation overnight to optimise postextubation breathing ability.
For many patients, ventilation is provided purely for initial airway and apnoea protection rather than for treatment of pulmonary deficits. In the absence of pulmonary disease, many centres provide fairly uniform approaches to parameter settings that aim at sustaining ventilation and oxygenation, while limiting traumatic risk to the lungs (see Table 12.1). However, approaches to ventilation will need to be tailored in the presence of operative complications or coexisting lung disease.
Nominal or generally acceptable settings | Alternatives to nominal settings and reasons for variation |
---|---|
SIMV with volume control ventilation | Pressure control suitable. Generally used only if there is significant hypoxaemia or the need to exert greater control on pulmonary pressure. Hybrid modes such as Autoflow, pressure-regulated volume control or volume control plus (VC+) are also suitable, generally for same indications as pressure control. |
Tidal volume 8–10 mL/kg | Lower tidal volumes (6–8 mL/kg) when there is known compliance disorder (atelectasis, pulmonary oedema, fibrosis) or unexplained high plateau pressures. |
Mandatory rate 10 L/min | Faster rates may be necessary if low tidal volume strategies become necessary. Lower rates if gas trapping risk due to airways disease. |
Inspiratory flow 30–50 L/min to provide I : E ratio of 1 : 2 to 1 : 4 acceptable | Slower flows to prolong the inspiratory time may be necessary if there is atelectasis and hypoxaemia, or if there is a desire to lessen inspiratory pressures. Faster flows to enhance expiratory time necessary only if gas-trapping risk. |
PEEP minimum levels of 5 cmH2O | Higher levels of PEEP according to severity of hypoxaemia. |
Pressure support 5–10 cmH2O | Automated pressure support modes such as automatic tube compensation (autoadjusted pressure support according to overcome flow resistance of tracheal tubes) or volume support (autoadjusted pressure support to achieve target tidal volume on spontaneous breaths) exist. There is no pressing indication for their use in uncomplicated cardiac surgical patients. |
Permissive hypercapnoea rarely necessary | Particularly important to avoid if existing pulmonary hypertension, as may worsen acutely with respiratory acidosis. |
FiO2 initially 1.0 then wean down according to PaO2/SaO2 | According to PaO2/SaO2. |
Ventilation challenges specific to the postcardiac surgical setting include:
• atelectasis due to operative access
• pneumothorax (pleural opening for grafts, or ventilation-induced trauma)
• pulmonary hypertension from cardiac failure or valve disease
• cardiogenic shock/post-pump failure
• systemic inflammatory response syndrome due to cardiopulmonary bypass
• early or rapid weaning that is undertaken before complete readiness, leading to failure at weaning attempt
• surgical pain limiting spontaneous effort and potentially leading to atelectasis or sputum retention.
Approaches to weaning
As patients often have no underlying pulmonary pathology, and have been ventilated for brief periods only, rapid weaning phases have become the norm in most centres. In many instances, as soon as the patient wakes and begins spontaneous breathing activity he/she may be suitable for at least a trial of spontaneous breathing in CPAP mode, usually with some modest level of pressure support (e.g. 5–10 cm H2O). If tolerated and the patient maintains an adequate minute volume, SpO2 and PaCO2, then extubation may be considered within as little as another 30 minutes. Normal demonstrations of airway protection capability (e.g. neuromuscular control, gag, swallow, cough and patient strength) should be sought before extubation (see Chapter 15 for details).
Where ventilation has been more prolonged due to postoperative pulmonary problems, weaning may be approached more cautiously, as might be applied to the general longer-term ventilated patient. Gradual mandatory rate reduction or increasing periods of spontaneous ventilation interspersed with periods of greater assistance have been used.31
Assessment and Management of Postoperative Bleeding
Blood transfusions are not aimed at restoring haemoglobin to normal levels, and, despite variations in acceptable levels, relative anaemia is almost universally tolerated. Haemoglobin levels are thus not routinely treated unless below 80 g/L, except in the elderly or when there are significant comorbidities.19,32 From these levels patients return to normal haemoglobin status within 1 month postoperatively.32
Contributors to impaired haemostatic capability
• cardiopulmonary bypass influences:
• preoperative anticoagulant/antiplatelet medications commonly encountered
• increased fibrinolytic activity
• surgical defects such as failure of access site closure, or vascular anastomosis defects.
Bedside assessment of bleeding
The activated clotting time (ACT) is the most commonly used assessment of coagulation and heparin activity during cardiac surgery and subsequently in intensive care. It measures the time to onset of fibrin formation (initial clot development). The ACT has been valuable because it can be inexpensively and efficiently performed at the bedside, providing prompt results and requiring only modest personnel training. Bleeding patients with a prolonged ACT come under consideration for administration of protamine or other agents.32 Treatable levels vary from greater than 120 sec to greater than 150 sec among different centres.
A limitation of ACT measurements is that they provide no information about clotting processes beyond initial fibrin formation, so clotting deficits such as impaired clot strength or the presence of significant fibrinolysis as contributors to bleeding are not revealed by this test.33 By contrast, the thromboelastograph (TEG) measures the clotting process as it proceeds over time.33 TEG monitoring not only reveals abnormalities early in the clot process (time to fibrin formation, as would be demonstrated by the ACT) but also the subsequent development of clot strength, clot retraction, and finally fibrinolytic activity for each of their contributions in the bleeding patient.33 TEG monitoring, although considerably more expensive than the ACT, is now available as a bedside or operating room technology and offers better insight into bleeding causes. In addition, because TEG monitoring identifies deficiencies at the various stages of clot formation, development of clot strength and the presence of undue fibrinolytic activity, it may permit better matching of procoagulant, blood product or antifibrinolytic therapy to needs.33
Heparin reversal
Cardiopulmonary bypass requires full heparinisation (initially 300 IU/kg), which is reversed at end-operation.32 The specific antidote, protamine sulphate, is administered as bypass is ceased, at a dose of 1 mg per 100 units heparin used (i.e. 3 mg/kg).32 If reversal is less than complete, as evidenced by a prolonged ACT, further protamine sulphate (at doses of 25–50 mg over 5–10 minutes) may be necessary.
Management of bleeding
Treatment approaches to bleeding once the patient is in intensive care include further protamine administration if the ACT remains prolonged, blood and blood product administration (platelets, clotting factors, fresh frozen plasma), procoagulants (desmopressin acetate) and antifibrinolytic agents (see Table 12.2 for more details). Other general measures such as rewarming the patient and preventing or treating hypertension should be undertaken.
TABLE 12.2 Management of the bleeding patient post-cardiac surgery21,22,25,33,34,44
Therapy | Dose | Comments/issues |
---|---|---|
Protamine sulphate | 25–50 mg slow IV (<10 mg/min); may be repeated if ACT prolonged | Specific antidote to heparin. May cause hypotension. Contraindicated in patient with seafood allergy. |
Aprotinin (Trasylol) | continuous infusion of 2 million units over 30 min, then 500,000 units per hour | Antifibrinolytic. Proteinaceous. Anaphylaxis risk on re-exposure. Alert should be posted on history. |
Desmopressin acetate (DDAVP) | 0.4 mcg/kg IV | Promotes factor VIII release; limited evidence for use. |
‘Pump blood’ (blood retrieved from bypass circuit at end-operation) | often 400–800 mL | This is the remaining blood in bypass circuit; usually centrifuged before returning to patient; note: this blood contains heparin from CPB. |
Whole blood/packed cells | as necessary to achieve Hb >80 g/L or more according to needs | Autologous blood sometimes available when patients have donated blood preoperatively. |
Fresh frozen plasma | as necessary | ‘Broad-spectrum’ factor replacement; contains most factors. Useful adjunct to massive blood transfusion. |
Platelet concentrates | as necessary | Generally ABO and Rh compatible preferred. |
Epsilon-aminocaproic acid (Amicar) | 100 mg/kg IV followed by 1–2 g/h | Antifibrinolytic. Inhibits plasminogen activation. |
Cryoprecipitate | 10 units IV | Contains factor VIII and fibrinogen (factor I). |
Calcium chloride or gluconate | 10 mL 10% solution | Used to offset citrate binding of calcium in stored blood. |
Prothrombinex | 20–50 IU/kg IV | Contains factors II, IX and X. |
Assessment and Management of Pericardial Tamponade
Described as one of the extra-cardiac obstructive shocks, pericardial tamponade often resembles cardiogenic shock. The low cardiac output and hypotension result in oliguria, altered mentation, peripheral hypoperfusion and development of lactic acidosis. Compensation includes tachycardia and marked vasoconstriction, elevating the systemic vascular resistance. As in cardiogenic shock, there is usually elevation of the filling pressures (right atrial, pulmonary artery and pulmonary capillary wedge pressures), sometimes with a particularly suggestive merging of the pulmonary artery diastolic, right atrial and pulmonary artery wedge pressures.23 Additional features that may be present include muffled heart sounds, decreased QRS voltage, electrical alternans, narrowing pulse pressure and pulsus paradoxus, along with features of increasing anxiety and/or dyspnoea in the awake patient.
Importantly, the ‘classic’ or typical haemodynamic profile described above is not uniformly seen in tamponade, and tamponade should never be excluded because the haemodynamic status does not match this profile. This may be because classic tamponade implies uniform compression of the entire heart, which may not be the case with haemorrhagic tamponade. A clot may develop over just one chamber rather than occupying the entire pericardium, and so there may be compromise to only a single chamber rather than the whole heart.21,23
Management of pericardial tamponade
Steps to control bleeding and blood pressure as described above may limit further losses into the pericardium. All steps should be taken to maintain or re-establish chest tube patency (crushing clots within tubing, ‘milking’ when it is truly necessary) and to ensure free flow of blood from the chest by avoiding dependent tubing loops, or side-to-side rolling of the patient to possibly bring collections into proximity of drain tubes. When tube patency is in doubt, the surgeon may even pass a suction catheter through the chest drain under aseptic conditions in an attempt to remove clots at the drain tip.23 If the above measures do not relieve tamponade, consideration is given to re-exploring the pericardium, either by returning to the operating theatre or, in an emergency, to the intensive care unit, although this is less preferable.
Assessment and Management of Postoperative Pain
As much an art as a science, pain control in the cardiac surgical patient remains a major challenge and continues to provide uncertainty and opportunities for nursing clinicians and researchers. Principles are similar to those outlined in Chapter 7. Surgical pain, often complicated by pericardial inflammation, results in differing pain types, requiring different approaches.34 These different approaches to pain management must be balanced against the promotion of spontaneous breathing, chest physiotherapy, mobilisation, and participation in education and lifestyle modification programs.
Analgesic options include intravenous, oral or rectal analgesics, antiinflammatories and, less commonly, epidural therapies and nerve blocks. Intravenous opiates and codeine/paracetamol preparations provide the mainstay of postoperative analgesia. When insufficient, or when clinical and electrocardiographic features suggest pericarditis, antiinflammatory agents such as rectal indomethacin are appropriate. The place of IV COX-2 inhibitors such as parecoxib appear uncertain, as analgesic efficacy now must be weighed against emerging data suggesting increased thrombotic complications.35
Fluid and Electrolyte Management
Fluid therapy in the postoperative period is aimed at maintaining blood volume, replacing recorded and insensible losses, and providing adequate preload to sustain haemodynamic status. Isotonic dextrose solutions (5%) or dextrose 4% + saline 0.18% are commonly used at approximately 1.5 L/day as maintenance fluids.14
Potassium replenishment is generally necessary according to measured serum potassium. Polyuria is usually evident in the early postoperative period due to deliberate haemodilution while on cardiopulmonary bypass. With polyuria comes potassium losses, which must be treated to avert atrial or ventricular ectopy and tachyarrhythmias. Because of these predictable potassium losses, protocols for potassium replacement may be instituted, with standing orders for potassium replacement (e.g. 10 mmol over 1 hour if the serum potassium is <4.5 mmol/L, or 20 mmol over 2 hours if <4.0 mmol/L). Main line hydration infusions may also have added potassium to avoid hypokalaemia. Hypomagnesaemia may also develop due to polyuria, and is likewise proarrhythmic. Supplementation (magnesium chloride) is often used for arrhythmia management postoperatively, but its effectiveness has been questioned in many trials.36
Emotional Responses and Family Support
The experience of being diagnosed with a cardiac disorder, waiting for surgery, the surgical experience and recovery is an emotional journey for patients and their families. Regardless of low mortality rates, the possibility of death and painful wounds can concern patients. Consequently, patients undergoing cardiac surgery often experience anxiety and depression, which can be distressing for patient and family.37–39 Women appear to be more vulnerable to these emotions in relation to cardiac surgery than men.40 Although it is normal and potentially protective to experience anxiety, higher levels of these emotions can be destructive. Anxiety and depression are predictive of worse postoperative outcomes, including poorer psychosocial adjustment and quality of life, more cardiac symptoms and readmissions.41 Therefore, it is essential to consider and address anxiety and depression when providing care for cardiac surgical patients.