Chapter 12 Cardiac Performance
INTRODUCTION
An assessment of cardiac performance constituted the first nuclear cardiology studies performed in man. As early as 1927, Blumgart and Weiss in a series of elegant papers identified characteristics of hemodynamic performance as measured by circulation times in normals and in patients with significant cardiovascular disease, utilizing injected radon followed by measurements obtained with a modified Wilson cloud chamber over the thorax and contralateral upper extremity.1 These pioneering studies called attention to the potential of utilizing radioisotope technology for answering relevant clinical questions.
Presently, cardiac performance can be evaluated by nuclear cardiology in three general manners. Two involve measurement of radioactivity within the blood pool, while the third applies the principles of electrocardiography (ECG) gating to conventional myocardial perfusion single-photon emission computed tomography (SPECT) studies. This latter technique is discussed in detail in Chapter 13 and consequently will not be developed here. This chapter will concentrate on the initial techniques of equilibrium intravascular labeling, which allows repeated imaging over several hours (equilibrium radionuclide angiocardiography [ERNA]), and the first-pass technique during which analysis of the first transit of a radionuclide bolus through the central circulation is assessed (first-pass radionuclide angiocardiography [FPRNA]). Although the intravascular radionuclide approaches for the evaluation of ventricular function have been challenged by both echocardiography and gated perfusion SPECT, these specific techniques still play a role in the quantitative assessment of cardiac performance. These intravascular approaches and their clinical implications and applications are discussed subsequently.
EQUILIBRIUM RADIONUCLIDE ANGIOCARDIOGRAPHY
The basic concept of utilizing a physiologic input to “gate” or physiologically control radioisotope-labeled equilibrium cardiac blood pool data to measure left ventricular ejection fraction was first introduced in 1971.2,3 The physiologic signal chosen was the electrocardiogram, and the blood pool was initially labeled with technetium 99mTc human serum albumin. Left ventricular ejection fraction was measured and regional wall motion assessed visually from end-systolic and end-diastolic summed data.2 This approach built on earlier studies whereby “gating” had been achieved with intravenous first-pass or intraventricular injections of radioisotope and left ventricular ejection fraction evaluated from respective maxima and minima of the generated curves.4–7 ERNA uses the electrocardiographic signal to establish the temporal relationships between acquired nuclear data and volumetric events of the cardiac cycle. To accomplish this, sampling is performed during the time of radioisotopic equilibrium within the blood pool such that sequential data can be summed over several hundred cardiac cycles. These data are then segregated physiologically according to their time of occurrence within the cardiac cycle as determined by the simultaneously acquired electrocardiogram (Fig. 12-1). Data are accumulated until the radioactive count density over the cardiac region of interest is of sufficient magnitude for generation of statistically meaningful analysis. The data from the individual components of this temporal segregation of nuclear data are displayed in an endless-loop scintigraphy movie and as a ventricular volume curve for qualitative visual assessment as well as for quantification of global left ventricular function (Fig. 12-2).
Technical Issues
The duration of study necessitated by utilizing the equilibrium technique can be both an advantage and a disadvantage.8 Because studies involve summation of several hundred cardiac cycles, a number of time-related specific issues must be considered. First, the patient must be able to remain relatively still beneath the detector during the period of acquisition. Second, most studies continue to be obtained with the planar technique. Consequently, data are acquired in multiple views in order for complete interpretation. Generally, this involves the anterior, left anterior oblique, and either left lateral or left posterior oblique views. The need for multiple views is inherent in the planar technique to account for superimposed radioactivity in multiple cardiac and noncardiac structures that can at times obscure analysis of a given region of interest. Such views are also important for defining specific regional left ventricular abnormalities such as ventricular aneurysm or severe akinesis. Third, intrinsic to this approach is the assumption that cardiac performance will remain relatively stable during the entire period of acquisition. Such stability is not present in instances of rapidly changing ventricular responses to atrial fibrillation or frequent premature beats of either ventricular or atrial origin. Fourth, the radionuclide label must remain stable during the period of analysis. (For conventional clinical imaging, this is usually not a problem). Fifth, the framing acquisition interval also must be of sufficient duration to allow statistically meaningful data as well as adequate temporal resolution for definition of parameters of systolic and diastolic ventricular performance.
Gated Blood Pool Single-Photon Emission Computed Tomography
The equilibrium radionuclide technique can also be readily applied to SPECT rather than planar studies.9–14 SPECT blood pool imaging would have some obvious advantages with respect to three-dimensional visualization and elimination of problems induced by overlapping structures (Fig. 12-3). Recently, new algorithms have been developed to improve calculations of both left ventricular ejection fraction and ventricular volumes from gated SPECT studies. It is likely that algorithms such as those recently proposed will enhance the ability to move gated SPECT technology from the research to the clinical environment for assessment of ventricular function.14 However, at the present time, work has been primarily experimental, and advantages have not been of sufficient magnitude to replace the less time-consuming and complex planar approach.
A recent study by Nichols et al. analyzed automated quantitative analysis of gated blood pool SPECT for assessing regional and global wall motion using magnetic resonance imaging as a standard. They found good agreement with independent magnetic resonance imaging calculations and demonstrated that the automated technique was superior to visual analysis.14
Performance
Labeling of the blood pool at equilibrium is obtained using 99mTc fixed to the patient’s own red blood cells.16 This technique is standard and can be done using either an in vitro or modified in vivo technique employing unlabeled stannous pyrophosphate as a facilitator of the labeling process. The in vitro approach has a labeling efficiency of greater than 97% and is the current method of choice.15 A single labeling procedure will involve sufficient radioactivity present in the blood pool to allow for serial studies over a period of 4 to 6 hours. A dose of 25 to 30 mCi is used.
Conventional Anger scintillation cameras are used for these studies. If planar imaging devices are not available, then the single head of a SPECT instrumentation camera can be employed. A 64 ∞ 64 matrix should be used. Pixel size should be less than 4 mm/pixel. No zoom should be applied to a 10-inch field of view camera, and 1.5 to 22 zoom used in a large field of view camera.16 Data are analyzed by computer, either totally automatically or with operator interaction. There must be sufficient radioactivity within the field to allow for quantitative analysis. Generally, studies are acquired over sufficient time to accumulate greater than 4 million counts. At the present time, most studies are performed in the resting state. This evaluation can be performed in multiple views within 15 minutes. Collimation generally involves low-energy high-resolution collimators. If exercise studies are used, high-sensitivity collimators or low-energy all-purpose or low-energy high-sensitivity collimators should be used. Background subtraction is necessary because there is activity throughout the intravascular space. In some programs, the background region is placed 4 pixels outside the lateral border of the left ventricular region of interest. In other programs, this may be done manually. In either case, it is key to be sure that background is not chosen over a very high count area such as the aorta or spleen, since this will lead to erroneous measurements.16
There are two general modes of data acquisition: frame mode or list mode. At the present time, frame mode is most widely used.16–18 In this approach, a specified duration for each portion of the cardiac cycle is established. For resting studies this usually is between 10 and 40 msec or, generally, 16 frames/cycle, depending on the patient’s intrinsic heart rate and the conditions of the study. This same frame duration will be utilized throughout the study, irrespective of any changes in the heart rate. Beat rejection programs are possible, which allow after the fact elimination of pre-specified duration premature and post-extrasystolic beats. A 10% to 15% window around the R-R peak is standard, and beats not falling within this window are rejected. This window will take into account normal physiologic variability. With list mode, analysis is made after acquisition and depends on cycle length. Consequently, with this technique a more accurate evaluation can be obtained in the presence of changes in cardiac cycle length. However, this approach is a bit more complicated and expensive and is for the most part limited to research studies.
where bc = background corrected. With this technique, the lower count of normal is 0.50 (50%).18 In addition to the conventional measurement of left ventricular ejection fraction, other indices of left ventricular ejection as well as ventricular filling can be made. These include ejection time, ejection rate, peak filling rate, and time to peak filling rate. Filling indices are measured in units of end-diastolic volumes per second (EDV/sec, normal < 2.5). To measure diastolic parameters, data must either be acquired at higher temporal resolution (i.e., 24 frames/R-R cycle) or have Fourier filtering applied to the standard 16 frame/cycle ERNA.19 In the generated volume curve, a smooth diastolic upslope concludes with a visible “atrial kick” (see Fig. 12-2).
Ventricular volumes can also be determined by count-based methods. Because at equilibrium blood pool radioactivity is proportional to volume, by using an appropriate region of interest and accounting for attenuation, it is possible to measure chamber volume.20–23 This may be accomplished by either acquisition and counting of a reference blood sample or by measuring pixel size for calibration. Volumes measured in this manner correlate well with other analyses. Because analysis is count-based, the data are free from errors associated with geometric analysis of volume. Ventricular volume measurements are increasingly important clinically with respect to serial monitoring and assessing ventricular remodeling.
Interpretation of ERNA studies requires both visual and quantitative analysis. Quantitative evaluation of right ventricular function is difficult with this technique because of contamination from anterior overlying right atrial activity. Consequently, right ventricular global function is best evaluated by concomitant first-pass techniques.24,25 The degree of left anterior obliquity chosen for ERNA requires operator interaction to ensure optimal separation of right and left ventricles. This is generally achieved at a 45-degree angle but often requires operator interaction to account for individual anatomic variation. From this view, measurement of global left ventricular function such as LV ejection fraction is obtained. Each of the three views employed provides qualitative information concerning regional contraction. The left anterior oblique view provides views of the septal, inferoapical, and lateral walls. The anterior view provides information concerning contraction of the anterior and apical segments. The left lateral or left posterior oblique views allow insight into contraction of the inferior wall and posterobasal segments. In addition, since the entire intravascular blood pool is labeled, information is derived concerning all cardiac and vascular structures such as the atria, aorta, and pulmonary vasculature. Regional function can also be quantified utilizing regional ejection fraction measurements with this approach; the left ventricular blood pool is divided into four to five segments, and ejection fraction is derived from each segment (Fig. 12-4).26,27 This analysis is also done in the left anterior oblique view.
Phase Imaging
Parametric phase images are derived from the same radionuclide data utilized for standard ERNA measures of right and left ventricular function.28,29 Commercially available computer programs are utilized. A phase angle is assigned to each pixel of the phase image. This is derived from the first Fourier harmonic of time. The phase angle () corresponds to the relative pattern and sequence of contraction in the cardiac chamber of interest throughout a single cardiac cycle. Generally, color coding is used and corresponding histograms are generated in each study. Dyssynchrony of cardiac contraction, whether intraventricular or interventricular, is evaluated from the difference in appropriate mean phase angles (Fig. 12-5). In the case of intraventricular dyssynchrony, right ventricle–left ventricle delays are evaluated. In the case of intraventricular dyssynchrony, this is measured by the standard deviation of the mean phase angle for the left ventricle. The technique has been used to identify sites of bypass tracks, sites of arrhythmogenesis, and altered contraction patterns in cardiomyopathy, and to assess the impact of biventricular pacer resynchronization therapy on the efficiency of cardiac contraction.20–34 The technique has also recently been employed to localize ventricular tachycardia exit sites as well as subsequent contraction sequence and functional effects of arrhythmia. In this very technically challenging study, a total of 26 patients with 32 episodes of ventricular tachycardia were studied. This occurred both in the electrophysiology laboratory and spontaneously. The phase analysis technique was quite accurate when compared to electrophysiologic study for defining exit site of the arrhythmia. In addition, there were good correlations between the imaging findings and the patient’s ability to tolerate the specific arrhythmia.34
Ambulatory Monitoring
An additional application of the ERNA principle involves utilization of miniaturized portable equipment for monitoring patients during routine activities.35 Instrumentation has been developed that allows for monitoring over several hours following equilibrium blood pool labeling. The device, called the VEST, is worn by patients such that they may be fully ambulatory. Radionuclide and electrocardiographic signals are stored on tapes comparable to Holter monitoring for analysis of arrhythmias. Off-line analysis allows trending of data concerning left ventricular ejection fraction and relative volumes (Fig. 12-6). This approach has been standardized in several laboratories and has been utilized for the assessment of silent myocardial ischemia as well as pharmacologic intervention.36–38 Newer-generation equipment has been developed for this assessment.
First-Pass Radionuclide Angiocardiography
The first-pass approach offers an alternative to ERNA.8,39 However, because it is somewhat more technically demanding, it is performed less frequently. Nevertheless, it can be used routinely at the time of any 99mTc-labeled pharmaceutical injection such as myocardial perfusion agents.40–44 This option presents a distinct advantage.
With the first-pass technique, sampling occurs only during the initial seconds of transit of the radioactivity bolus through the central circulation. Analysis is made of the high-frequency components of this signal. The basic assumption of this approach is that there has been sufficient admixture of radioactivity with circulating blood such that the count rate changes recorded represent a true proportionality to volumetric changes within the cardiac chamber of interest. The temporal segregation of the radioactivity bolus allows independent evaluation of right and left ventricular function (Fig. 12-7). Regional function can also be assessed.
Any technetium-labeled radiopharmaceutical can be used for a first-pass technique. For the most part, this had previously either been 99mTc-pertechnetate or 99mTc complexed to other carriers such as DTPA or sulfur colloid. In such a manner, multiple injections can be made for assessment of function at both rest and exercise.39,45,46 Currently, first-pass studies are generally done at the time of tracer injection for either ERNA or perfusion SPECT. Other short-lived generator systems have been employed experimentally; these include generators involving tungsten-178-tantalum-178,47 osmium-191-iridium-191m,48–51 and mercury-195m-gold-195m.52–55 However, none have been used clinically to any substantial extent.
The scintillation camera used for first-pass studies is extremely important. To provide statistically meaningful high count rate data, cameras must be able to provide high sensitivity with system linearity and no major dead-time losses.8,56 Initial approaches utilizing this technique involved multicrystal scintillation cameras. This instrumentation has since been replaced by second- and third-generation digital scintillation cameras suitable for rapid acquisition of high count rate data. Another experimental imaging system is the multiwire proportional chamber gamma camera.57 However, this system provides optimal data only for low energies less than 100 keV, such as those provided by tantalum-178.
Studies are obtained in the anterior or right anterior oblique views. Data from FPRNA are computer processed in frame mode. Regions of interest are selected over the chamber of interest. Generally these are fixed regions. Activity is analyzed only as the initial bolus traverses the chamber of interest, the right or left ventricle. A background correction is necessary. A number of approaches to background have been described, but generally a fixed background is used.16,24 The first-pass approach is the best technique for assessing global right ventricular function. This can also be used in the process of ERNA studies evaluating the left ventricle, with analysis performed at the time of initial radionuclide injection.
A variation called the gated first-pass technique can be used as well. With this technique, there is a merging of first-pass and equilibrium technology. First-pass data are acquired in concert with the ECG signal. These data are stored and summed, and several beats are used to form a representative cardiac cycle assessing the right heart phase of transit. Separate regions of interest are defined for end-systole and end-diastole. No background subtraction is necessary.16 This provides higher count data than that obtainable with a simple bolus injection.
Shunt Detection
The first-pass study also may be utilized to detect and quantify intracardiac shunts.58–63 A region of interest is selected over the lung field. The pulmonary time-activity curve from this region is then analyzed. Under normal circumstances there will be a sharp rise in the time-activity curve as the bolus enters the pulmonary circulation, with a subsequent rapid falloff before recirculation. In the presence of a significant left-to-right shunt, activity will remain in the lung, and pulmonary washout will be relatively slow. The degree of shunting measured by this technique correlates well with other standard techniques. While this technique remains a viable option, it is clear that Doppler echocardiographic techniques are the method of choice and the most widely applied technology for assessing shunts.
CLINICAL APPLICATIONS
Resting Ventricular Performance
Measurement of left and right ventricular performance in the resting state provides important insight with respect to a large number of cardiovascular conditions. This includes prognosis in both coronary and non-coronary cardiovascular disease.8,56,64 Evaluation of global left ventricular function can assist in therapeutic decisions ranging from implantation of automatic internal defibrillators64 to monitoring of cancer chemotherapy (see Chapter 24).66,67 Distinction can be made between systolic and diastolic heart failure (see Chapter 23).68–72 Assessment can be made of relative chamber sizes, thereby providing reasonable inferences concerning the hemodynamic relevance of valvular lesions. Distinction can be made between cardiac and pulmonary etiologies of symptoms.
Coronary Artery Disease
Perhaps the widest clinical application of resting radionuclide ventricular function studies has involved the assessment of patients with both acute and stable coronary artery disease. Numerous reports over several decades have documented the importance of left ventricular function at rest as an important prognostic indicator in patients with coronary artery disease, with or without documented prior myocardial infarction.69–77 This prognostic importance has transcended the therapeutic approaches available in any one clinical era (Fig. 12-8). This evaluation was first established over 3 decades ago in the coronary artery surgery study (CASS), where the importance of prognostic stratification based on ejection fraction in patients with multivessel disease was first noted.78 In the present era, the relevance of ejection fraction in defining the need for placement of internal defibrillators following acute myocardial infarction has also been well established and has led to a major paradigm shift in how such patients are approached.65
Exercise Studies
A large volume of information has been generated concerning the value of exercise ventricular performance utilizing either ERNA or FPRNA techniques.56,64 Exercise may be performed in supine, semisupine, or upright positions. In patients with coronary artery disease, measurements during exercise may be expressed either in absolute terms of the exercise ejection fraction or as the change in ejection fraction from rest to exercise (Δ LVEF). Specific prognostic levels vary among studies.79–91 However, in general, risk of either death or myocardial infarction is at least 5% per year when (Δ) ejection fraction involves a decrease of 5% or more (in absolute numbers; Fig. 12-9).92 Data are parallel using absolute LVEF during exercise, with a cut level being less than 30%.93 However, despite these excellent results, exercise ventricular function studies are currently not widely performed and have been largely replaced by either exercise myocardial perfusion SPECT or stress echocardiography (see Chapters 13, 15, and 17).
Silent Myocardial Ischemia
Prognosis in ischemia appears to be comparable whether symptoms are present or not. This has been noted over decades with exercise studies.94 Using ambulatory ventricular function, silent myocardial ischemia can be readily evaluated. This has been noted during routine activities as well as under conditions of mental stress (see Chapter 25).35,95–98
Valvular Heart Disease
It is recognized that both diagnosis and accurate assessment of hemodynamic severity in valvular heart disease are best accomplished by echocardiography and Doppler techniques. Nevertheless, important prognostic information can be obtained utilizing radionuclide approaches, particularly with exercise.99 In aortic regurgitation, left ventricular ejection fraction at rest is a primary determinant of prognosis. Abnormal ejection fraction, even in the absence of symptoms, is considered an indication for valve surgery.100 Changes in ejection fraction with exercise also have provided important information concerning prognosis in patients with asymptomatic aortic regurgitation and normal ventricular function at rest.101 This parameter is even more accurate when combined with echocardiographically defined wall stress, in the form of a new myocardial contractility index (Fig. 12-10).102 Borer and colleagues demonstrated that this particular measurement is more predictive with respect to outcomes than either radionuclide or echocardiographic measures alone.
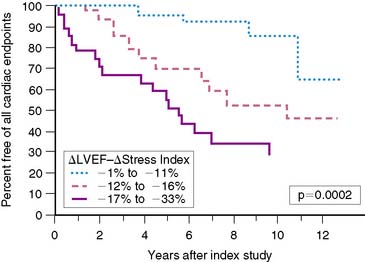
Figure 12-10 Relationship of myocardial contractility index to occurrence of any cardiac endpoint over follow-up.
(From Borer JS, Supino P: Equilibrium imaging. In Iskandrian AE, Verani MS [eds]: Nuclear Cardiac Imaging. Principles and Applications, 3rd ed. London: Oxford University Press, 2003, p 346.)
In mitral regurgitation, measurement of right and left ventricular ejection fraction can also have important applications with respect to assessing outcome. Because in mitral regurgitation there is unloading of the left ventricle by virtue of the pathophysiology of the specific valvular lesion, greater hemodynamic stress is placed on the right ventricle. Consequently, it has been suggested that right ventricular ejection fraction at rest and/or exercise is a more sensitive indicator of prognosis in this condition (Fig. 12-11).103–105 It is also recognized that right ventricular ejection fraction is extremely sensitive to changes in the afterload conditions faced by this chamber.25,106 The interaction of measures of both left and right ventricular ejection fraction can play an important role in assessing prognosis in patients with mitral regurgitation.
Congestive Heart Failure
Analysis of right and left ventricular ejection fraction plays an important role in the assessment of patients with known or presumed heart failure (see Chapter 23). Resting studies allow assessment of systolic versus diastolic dysfunction. Assessment of relative chamber sizes may provide additional insight into both the hemodynamic impact of silent valvular disease and the results of intervention. Serial measures at rest provide important insights into monitoring chemotherapeutic cardiotoxic effects (see Chapter 24). Assessment of global function can play an important role in patient selection for cardiac transplantation or ventricular assist device implantation.
Measurements of ejection fraction currently play a substantial role in defining suitability for implantation of cardioverter-defibrillators. Based on a number of major clinical trials, a clear-cut survival benefit has been demonstrated in individuals who have sustained a myocardial infarction or who have chronic congestive heart failure with substantial depressions in ejection fraction.106 Clearly the ability of the nuclear procedure to define ejection fraction in most precise terms would make this the most suitable modality for evaluating such patients.
Phase analysis has been used in two studies to assess the impact of biventricular pacing on interventricular and intraventricular dyssynchrony in patients with cardiomyopathy. Kerwin and colleagues evaluated a small series of patients with dilated cardiomyopathy and intraventricular conduction delay before and after biventricular pacing.33 The degree of interventricular dyssynchrony as measured by phase analysis present at baseline correlated with left ventricular ejection fraction. During biventricular pacing, interventricular dyssynchrony decreased. Left ventricular ejection fraction increased in all patients with biventricular pacing and the increment in ejection fraction correlated with improvement in interventricular dyssynchrony (Fig. 12-12).
Fauchier and colleagues evaluated 103 patients with idiopathic dilated cardiomyopathy.34 Patients were studied with phase ERNA and followed up for a mean of 27 ± 23 months. Eighteen patients had a major cardiac event. The degree of left and right ventricular dyssynchrony and QRS duration were predictors of cardiac events. However, the degree of interventricular dyssynchrony was not. The degree of left ventricular intraventricular dyssynchrony was an independent predictor of future cardiac events.
Chronic Obstructive Pulmonary Disease
The use of first-pass technology for monitoring right ventricular function can play an important role in assessing the hemodynamic consequences of longstanding lung disease. Since right ventricular performance is extremely afterload dependent, these measurements can provide important insights into associated pulmonary hypertension and its concomitant effects in patients with pulmonary disease.24,25
1. Blumgart H.L., Weiss S. Studies on the velocity of blood flow. VII: The pulmonary circulation time in normal resting individuals. J Clin Invest. 1927;4:399.
2. Strauss W.H., Zaret B.L., Hurley P.J., et al. A scintigraphic method for measuring left ventricular ejection fraction in man without cardiac catheterization. Am J Cardiol. 1971;28:575.
3. Zaret B.L., Strauss H.W., Hurley P.J., et al. A noninvasive scintiphotographic method for detecting regional ventricular dysfunction in man. N Engl J Med. 1971;284:1165.
4. Folse R., Braunwald E. Determination of fraction of left ventricular volume ejected per beat and of ventricular end-diastolic and residual volumes. Circulation. 1962;25:674.
5. Hoffmann G., Kleine N. Die methode der radiokardiographischen funktions analyse. Nuklearmedizin. 1968;7:350.
6. Bacharach S.L., Green M.V., Borer J.S., et al. ECG-gated scintillation probe measurement of left ventricular function. J Nucl Med. 1977;18:1176.
7. Van Dyke D.C., Anger H.O., Sullivan R.W., et al. Cardiac evaluation for radioisotope dynamics. J Nucl Med. 1972;13:585.
8. Wackers F.J.Th, Soufer R., Zaret B.L. Nuclear cardiology. In: Braunwald E., Zipes D.P., Libby P., editors. Heart Disease. 6th ed. Philadelphia: WB Saunders; 2001:273.
9. Corbett J.R., Jansen D.E., Lewis S.E., et al. Tomographic gated blood pool radionuclide ventriculography: Analysis of wall motion and left ventricular volumes in patients with coronary artery disease. J Am Coll Cardiol. 1985;6:349.
10. Corbett J.R. Tomographic radionuclide ventriculography: Opportunity ignored? J Nucl Cardiol. 1994;1:567.
11. Lu P., Liu X., Shi R., et al. Comparison of tomographic and planar radionuclide ventriculography in the assessment of regional left ventricular function in patients with left ventricular aneurysm before and after surgery. J Nucl Cardiol. 1994;1:537.
12. Groch M.W., DePuey E.G., Belzberg A.C., et al. Planar imaging versus gated blood-pool SPECT for assessment of ventricular performance: a multicenter study. J Nucl Med. 2001;42:1773.
13. Harel F., Finnerty V., Nao Q., et al. J Nucl Cardiol. 2007;14:544.
14. Nichols K.J., Van Tosh A., Wang Y., et al. Validation of gated blood-pool SPECT regional left ventricular function measurements. J Nucl Med. 2009;50:53.
15. Callahan R.J., Froelich J.W., McKusick K.A., et al. A modified method for the in vivo labeling of red blood cells with Tc-99m: Concise communication. J Nucl Med. 1982;23:315.
16. Wackers F.J.Th, Bruni W., Zaret B.L. Planar equilibrium radionuclide angiocardiography: Acquisition and processing protocols. In: Nuclear Cardiology: The Basics. Totowa, NJ: Humana; 2003:81.
17. Bacharach S.L., Green M.V., Borer J.S., et al. A real-time system for multi-image gated cardiac studies. J Nucl Med. 1977;18:79.
18. DePuey G.E., Garcia E.V. Updated imaging guidelines for nuclear cardiology procedures, part I. J Nucl Cardiol. 2001;8:G1.
19. Bacharach S.L., Green M.V., Borer J.S., et al. Left ventricular peak ejection rate, filling rate and ejection fraction: Frame rate requirements at rest and exercise. J Nucl Med. 1979;20:189.
20. Starling M.R., Dell’Italia L.J., Walsh R.A., et al. Accurate estimates of absolute ventricular volumes from equilibrium radionuclide angiographic count data using a simple geometric attenuation correction. J Am Coll Cardiol. 1984;3:789.
21. Links J.M., Becker L.C., Shindledecker J.G., et al. Measurement of absolute left ventricular volume from gated blood pool studies. Circulation. 1982;65:82.
22. Massardo T., Gal R.A., Grenier R.P., et al. Left ventricular volume calculation using a count-based ratio method applied to multigated radionuclide angiography. J Nucl Med. 1990;31:450.
23. Levy W.C., Cerqueira M.D., Matsuoka D.T., et al. Four radionuclide methods for left ventricular volume determinations: Comparison of a manual and automated technique. J Nucl Med. 1992;33:763.
24. Zaret B.L., Wackers F.J.Th. Measurement of right ventricular function. In: Gerson M.C., editor. Cardiac Nuclear Medicine. 3rd ed. New York: McGraw-Hill; 1997:387.
25. Shulman D.S. Assessment of the right ventricle with radionuclide techniques. J Nucl Cardiol. 1996;3:253.
26. Maddox D.E., Wynne J., Uren R., et al. Regional ejection fraction: a quantitative radionuclide index of regional left ventricular performance. Circulation. 1979;59:1001.
27. Zaret B.L., Wackers F.J., Terin M., et al. Assessment of global and regional left ventricular performance at rest and during exercise after thrombolysis in Myocardial Infarction (TIMI II) study. Am J Cardiol. 1992;69:1.
28. Botvinick E., Dunn R., Frais M., et al. The phase image: Its relationship to patterns of contraction and conduction. Circulation. 1982;65:551.
29. Botvinick E.H. Scintigraphic blood pool and phase image analysis: the optimal tool for the evaluation of resynchronization therapy. J Nucl Cardiol. 2003;10:424.
30. Botvinick E., Schechtmann N., Dae M., et al. Augmented pre-excitation assessed by scintigraphic phase analysis during atrial pacing. Am Heart J. 1987;114:738.
31. Munoz L., Chin M., Krishnan R., et al. Scintigraphic phase analysis in the study of ventricular tachycardia (abstract). J Clin Res. 1992;40:19A.
32. Botvinick E., Frais M., Shosa D., et al. An accurate means of detecting and characterizing abnormal patterns of ventricular activation by phase image analysis. Am J Cardiol. 1982;50:289.
33. Kerwin W.F., Botvinick E.H., O’Connell J.W., et al. Ventricular contraction abnormalities in dilated cardiomyopathy, effect of biventricular pacing to correct interventricular dyssynchrony. J Am Coll Cardiol. 2000;35:1221.
34. Fauchier L., Marie O., Casset-Senon D., et al. Interventricular and intraventricular dyssynchrony in idiopathic dilated cardiomyopathy. A prognosis study with Fourier phase analysis of radionuclide angioscintigraphy. J Am Coll Cardiol. 2002;40:2022.
35. Zaret B.L., Jain D. Monitoring of left ventricular function with miniaturized non-imaging detectors. In: Zaret B.L., Beller G.A., editors. Nuclear Cardiology: State-of-the-Art and Future Directions. 2nd ed. St. Louis: Mosby; 1999:91.
36. Burg M.M., Jain D., Soufer R., et al. Role of behavioral and psychological factors in mental stress induced silent left ventricular dysfunction in coronary artery disease. J Am Coll Cardiol. 1993;22:440.
37. Jain D., Burg M., Soufer R., et al. Prognostic implications of mental stress induced silent left ventricular dysfunction of patients with stable angina pectoris. Am J Cardiol. 1995;76:31.
38. Ciampi Q., Betocchi S., Violante A., et al. Hemodynamic effects of isometric exercise in hypertrophic cardiomyopathy: Comparison with normal subjects. J Nucl Cardiol. 2003;10:154.
39. Marshall R.C., Berger H.J., Costin J.C., et al. Assessment of cardiac performance with quantitative radionuclide angiocardiography: Sequential left ventricular ejection fraction, normalized left ventricular ejection rate, and regional wall motion. Circulation. 1977;56:820.
40. Baillet G.Y., Mena I.G., Kuperus J.H., et al. Simultaneous technetium-99m MIBI angiography and first-pass radionuclide angiography. J Nucl Med. 1989;30:38.
41. Williams K.A., Taillon L.A. Gated planar technetium-99m-sestamibi myocardial perfusion image inversion for quantitative scintigraphic assessment of left ventricular function. J Nucl Cardiol. 1995;2:285.
42. Elliott A.T., McKillop J.H., Pringle S.D., et al. Simultaneous measurement of left ventricular function and perfusion. Eur J Nucl Med. 1990;17:310.
43. Boucher C.A., Wackers F.J., Zaret B.L., et al. Technetium-99m sestamibi myocardial imaging at rest for assessment of myocardial infarction and first-pass ejection fraction. Multicenter Cardiolite Study Group. Am J Cardiol. 1992;69:22.
44. Borges-Neto S., Curtis M.A., Morris E.I., et al. Combined Tc-99m sestamibi first-pass radionuclide angiography and cardiac SPECT imaging during arbutamine infusion delivered by a computerized closed-loop system. Clin Nucl Med. 1999;24:42.
45. Francis C.K., Cleman M., Berger H.J., et al. Left ventricular systolic performance during upright bicycle exercise in patients with essential hypertension. Am J Med. 1983;75:40.
46. Williams K.A., Taillon L.A., Draho J.M., et al. First-pass radionuclide angiographic studies of left ventricular function with Tc-99m-teboroxime, Tc-99m-sestamibi and Tc-99m-DTPA. J Nucl Med. 1993;34:394.
47. Lacy J.L., Layne W.W., Guidry G.W., et al. Development and clinical performance of an automated, portable tungsten-178/tantalum-178 generator. J Nucl Med. 1991;32:2158.
48. Treves S., Cheng C., Samuel A., et al. Iridium-191 angiocardiography for the detection and quantitation of left-to-right shunting. J Nucl Med. 1980;21:1151.
49. Packard A.B., Day P.J., Treves S.T. An improved 1910s/191mIr generator using a hybrid anion exchanger. Nucl Med Biol. 1995;22:887.
50. Hellman C., Zafrir N., Shimoni A., et al. Evaluation of ventricular function with first-pass iridium-191m radionuclide angiocardiography. J Nucl Med. 1989;30:450.
51. Heller G.V., Treves S.T., Parker J.A., et al. Comparison of ultra-short-lived iridium-191m with technetium-99m for first pass radionuclide angiocardiographic evaluation of right and left ventricular function in adults. J Am Coll Cardiol. 1986;7:1295.
52. Wackers F.J., Giles R.W., Hoffer P.B., et al. Gold-195m, a new generator-produced short-lived radionuclide for sequential assessment of ventricular performance by first pass radionuclide angiocardiography. Am J Cardiol. 1982;50:89.
53. Mena I., Narahara K.A., de Jong R., et al. Gold-195m, an ultra-short-lived generator-produced radionuclide: Clinical application in sequential first pass ventriculography. J Nucl Med. 1983;24:139.
54. Elliott A.T., Dymond D.S., Stone D.L., et al. A 195mHg-195mAu generator for use in first-pass nuclear angiocardiography. Phys Med Biol. 1983;28:139.
55. Fazio F., Gerundini P., Margonato A., et al. Quantitative radionuclide angiocardiography using gold-195m. Am J Cardiol. 1984;53:1442.
56. Williams K.A., Borer J.S., Supino P. Radionuclide angiography. In: Iskandrian A.E., Verani M.S., editors. Nuclear Cardiac Imaging, Principles and Applications. 3rd ed. London: Oxford University Press; 2003:323.
57. Verani M.S., Lacy J.L., Guidry G.W., et al. Quantification of left ventricular performance during transient coronary occlusion at various anatomic sites in humans: A study using tantalum-178 and a multiwire gamma camera. J Am Coll Cardiol. 1992;19:297.
58. Maltz D.L., Treves S. Quantitative radionuclide angiocardiography: Determination of Qp:Qs in children. Circulation. 1973;47:1049.
59. Arheden H., Holqvist C., Thilen U., et al. Left-to-right cardiac shunts: comparison of measurements obtained with MR velocity mapping and with radionuclide angiography. Radiology. 1999;211:453.
60. Tian J.H., Murray I.P., Walker B., et al. First-pass radionuclide angiocardiography in the determination of left-to-right cardiac shunt site in children. Cathet Cardiovasc Diagn. 1982;8:459.
61. Baker E.J., Ellam S.V., Lorber A., et al. Superiority of radionuclide over oximetric measurement of left to right shunts. Br Heart J. 1985;53:535.
62. Eterovic D., Dujic Z., Popovic S., et al. Gated versus first-pass radioangiography in the evaluation of left-to-right shunts. Clin Nucl Med. 1995;20:534.
63. Kelbaek H., Aldershvile J., Svendsen J.H., et al. Evaluation of left-to-right shunts in adults with atrial septal defect using first-pass radionuclide cardiography. Eur Heart J. 1992;13:491.
64. Borer J.S., Supino P. Equilibrium imaging. In: Iskandrian A.E., Verani M.S., editors. Nuclear Cardiac Imaging. Principles and Applications. 3rd ed. London: Oxford University Press; 2003:346.
65. Moss A.J., Zareba W., Hall W.J., et al. Prophylactic implantation of a defibrillator in patients with myocardial infarction and reduced ejection fraction. N Engl J Med. 2002;346:877.
66. Schwartz R.G., Zaret B.L. The diagnosis and treatment of drug-induced myocardial disease. In: Muggia F.M., Green M.D., Speyer J.L., editors. Cancer Treatment and the Heart. Baltimore: Johns Hopkins University Press; 1992:173.
67. Jain D. Cardiotoxicity of doxorubicin and other anthracycline derivatives. J Nucl Cardiol. 2000;7:53.
68. Setaro J.F., Zaret B.L., Schulman D.S., et al. Usefulness of verapamil for congestive heart failure, abnormal diastolic filling and normal left ventricular systolic performance. Am J Cardiol. 1990;66:981.
69. Bonow R.O., Vitale D.F., Bacharach S.L., et al. Asynchronous left ventricular regional function and impaired global diastolic filling in patients with coronary artery disease: reversal after angioplasty. Circulation. 1985;71:297.
70. Bonow R.O., Frederick T.M., Bacharach S.L., et al. Atrial systole and left ventricular filling in hypertrophic cardiomyopathy: Effect of verapamil. Am J Cardiol. 1983;51:1386.
71. Bonow R.O. Radionuclide angiographic evaluation of left ventricular diastolic function. Circulation. 1991;84:1208.
72. Bonow R.O., Bacharach S.L., Green M.V., et al. Impaired left ventricular diastolic filling in patients with coronary artery disease; assessment with radionuclide angiography. Circulation. 1981;64:315.
73. The Multicenter Post Infarction Research Group. Risk stratification and survival after myocardial infarction. N Engl J Med. 1983;309:331.
74. Rogers W.J., Papapietro S.E., Wackers F.J.Th, et al. Variables predictive of good functional outcome following thrombolytic therapy in the Thrombolysis in Myocardial Infarction Phase II (TIMI II) Pilot Study. Am J Cardiol. 1989;63:503.
75. Cerqueira M.D., Maynard C., Ritchie J.L., et al. Long-term survival in 618 patients from the Western Washington Streptokinase in Myocardial Infarction Trials. J Am Coll Cardiol. 1992;20:1452.
76. Candell-Riera J., Permanyer-Miralda G., Castell J., et al. Uncomplicated first myocardial infarction: Strategy for comprehensive prognostic studies. J Am Coll Cardiol. 1991;18:1207.
77. Zaret B.L., Wackers F.J.Th, Terrin M.L., et al. Value of radionuclide rest and exercise left ventricular ejection fractions in assessing survival of patients after thrombolytic therapy for acute myocardial infarction: Results of Thrombolysis in Myocardial Infarction (TIMI) phase II study. J Am Coll Cardiol. 1995;26:73.
78. CASS principal Investigators: Coronary Artery Surgery Study (CASS). A randomized trial of coronary bypass surgery. Survival data. Circulation. 1983;68:939.
79. Bonow R.O., Kent K.M., Rosing D.R., et al. Exercise-induced ischemia in mildly symptomatic patients with coronary artery disease and preserved left ventricular function. N Engl J Med. 1984;311:1339.
80. Clements I.P., Brown M.L., Zinsmeister A.R., et al. Influence of left ventricular diastolic filling on symptoms and survival in patients with decreased left ventricular systolic function. Am J Cardiol. 1991;67:1245.
81. Mazotta G., Bonow R.O., Pace L., et al. Relation between exertional ischemia and prognosis in mildly symptomatic patients with single- or double-vessel coronary artery disease and left ventricular function at rest. J Am Coll Cardiol. 1989;13:567.
82. Miller T.D., Taliercio C.P., Zinsmeister A.R., et al. Risk stratification of single- or double-vessel coronary artery disease and impaired left ventricular function using exercise radionuclide angiography. Am J Cardiol. 1990;65:1317.
83. Supino P.S., Wallis J.B., Chlouoverakis G., et al. Risk Stratification in the elderly patient after coronary artery bypass grafting: The prognostic value of radionuclide cineangiography. J Nucl Cardiol. 1994;1:159.
84. Iqbal A., Gibbons R.J., Zinsmeister A.R., et al. Prognostic value of exercise radionuclide angiography in a population-based cohort of patients with known or suspected coronary artery disease. Am J Cardiol. 1994;74:119.
85. Moriel M., Rozanski A., Klein J., et al. The differing prognostic utility of exercise radionuclide ventriculography in coronary artery disease patients with and without prior myocardial infarction. Int J Card Imaging. 1997;13:403.
86. Shapira I., Heller I., Isakov A., et al. Impact of early exercise radionuclide cineangiography on long-term prognosis after CABG. Ann Thorac Surg. 1997;64:473.
87. Griffin B.P., Shah P.K., Diamond G.A., et al. Incremental prognostic accuracy of clinical, radionuclide and hemodynamic data in acute myocardial infarction. Am J Cardiol. 1991;68:707.
88. Abraham R.D., Harris P.J., Roubin G.S., et al. Usefulness of ejection fraction response to exercise one month after acute myocardial infarction in predicting coronary anatomy and prognosis. Am J Cardiol. 1987;60:225.
89. Zhu W.X., Gibbons R.J., Bailey K.R., et al. Predischarge exercise radionuclide angiography in predicting multivessel coronary artery disease and subsequent cardiac events after thrombolytic therapy for acute myocardial infarction. Am J Cardiol. 1994;74:554.
90. Roig E., Magrina J., Armengol X., et al. Prognostic value of exercise radionuclide angiography in low-risk acute myocardial infarction survivors. Eur Heart J. 1993;14:213.
91. Mazotta G., Camerini A., Scopinaro G., et al. Predicting severe ischemic events after uncomplicated myocardial infarction by exercise testing and rest and exercise radionuclide ventriculography. J Nucl Cardiol. 1994;1:246.
92. Supino P.G., Borer J.S., Herrold E.M., et al. Prognostication in 3-vessel coronary artery disease based on left ventricular ejection fraction during exercise: Influence of coronary artery bypass grafting. Circulation. 1999;100:924.
93. Shaw L.J., Heinle S.K., Borges-Neto S., et al. Prognosis by measurements of left ventricular function during exercise. J Nucl Med. 1998;39:140.
94. Bonow R.O., Bacharach S.L., Green M.V., et al. Prognostic implications of symptomatic vs asymptomatic (silent) myocardial ischemia induced by exercise in mildly symptomatic and in asymptomatic patients with angiographically documented coronary artery disease. Am J Cardiol. 1987;60:778.
95. Burg M.M., Jain D., Soufer R., et al. Role of behavioral and psychological factors in mental stress induced silent left ventricular dysfunction in coronary artery disease. J Am Coll Cardiol. 1993;22:440.
96. Jain D., Burg M., Soufer R., et al. Prognostic implications of mental stress induced silent left ventricular dysfunction of patients with stable angina pectoris. Am J Cardiol. 1995;76:31.
97. Kayden D.S., Wackers F.J., Zaret B.L. Silent left ventricular dysfunction during routine activity following thrombolytic therapy for acute myocardial infarction: A predictor of subsequent cardiac morbidity. J Am Coll Cardiol. 1990;15:1500.
98. Jain D., Joske T., Lee F.A., et al. Day-to-day reproducibility of mental stress-induced left ventricular dysfunction in patients with coronary artery disease and its relationship to autonomic activation. J Nucl Cardiol. 2001;8:347.
99. Borer J.S., Wencker D., Hochreiter C. Management decisions in valvular function and performance. J Nucl Cardiol. 1996;3:72.
100. Bonow R.O., Picone A.L., McIntosh C.L., et al. Survival and functional results after valve replacement for aortic regurgitation from 1976 to 1983: Impact of preoperative left ventricular function. Circulation. 1985;72:1244.
101. Borer J.S., Hochreiter C., Herrold E.M., et al. Prediction of indications for valve replacement among asymptomatic or minimally symptomatic patients with chronic aortic regurgitation and normal left ventricular performance. Circulation. 1998;97:525.
102. Borer J.S., Supino P. Equilibrium imaging. In: Iskandrian A.E., Verani M.S., editors. Nuclear Cardiac Imaging. London: Oxford University Press; 2003:362. Fig. 17-22
103. Niles N., Borer J.S., Kamen M., et al. Pre-operative left and right ventricular performance in combined aortic and mitral regurgitation and comparison with isolated mitral or aortic regurgitation. Am J Cardiol. 1990;65:1372.
104. Wencker D., Borer J.S., Hochreiter C., et al. Preoperative predictors of late post-operative outcome among patients with nonischemic mitral regurgitation with “high risk” descriptors, and comparison with unoperated patients. Cardiology. 2000;93:37.
105. Hochreiter C., Niles N., Devereux R.B., et al. Mitral regurgitation: relationship of non-invasive descriptors of right and left ventricular performance to clinical and hemodynamic findings and to prognosis in medically and surgically treated patients. Circulation. 1986;73:900.
106. Brent B.N., Mahler D., Matthay R.A., et al. Noninvasive diagnosis of pulmonary hypertension in chronic obstructive pulmonary disease: Utility of resting right ventricular ejection fraction. Am J Cardiol. 1984;53:1349.