34 Burn Injuries
EVERY YEAR IN THE UNITED STATES millions of people are treated for burns; of these, hundreds of thousands are hospitalized, with a significant mortality rate.1–3 The National Burn Repository Report for 2006 reviewed their 10-year experience; overall mortality for 140,318 records was 5.3%. Inhalation injury was reported in 5.7% of cases; approximately 30% of burns were in children or adolescents age 20 years or younger, and the majority were scald burns or contacts with hot objects. Unfortunately, approximately 1166 cases were suspected child abuse. It is estimated that around 120,000 pediatric burn injuries are treated in the emergency department each year in the United States and the majority of patients are younger than 6 years of age.4 Children with burn injuries are well managed only when their care providers thoroughly understand the pathophysiologic and pharmacologic abnormalities associated with burn injury.5,6 These abnormalities include metabolic derangements, neurohumoral responses, massive fluid shifts, sepsis, and the systemic effects of massive tissue destruction. In this chapter we address the pathophysiology, the initial evaluation and resuscitation, and the anesthetic and pain management of children with burn injury. Some of the principles presented are the result of more than 30 years of experience in caring for children with burn injuries, and others are derived from experiences with adults and applied to children.
Each year, in the United States, approximately 15,000 children are hospitalized with burn injuries.1 The mortality rate has declined steadily over the past decades, owing to the advent of dedicated hospital burn centers,7,8 improved surgical techniques, and safer anesthetic management. However, almost 1100 children still die each year from fire and burn injuries. Safety prevention efforts such as smoke detectors do not seem to have reduced pediatric flame injuries because many flame injuries are related to children playing with matches,9,10 although there has been a small overall decrease in total burn injuries to children.11
Pathophysiology
Thermal injury to the skin disrupts the vital surface barrier that is responsible for thermal regulation, bacterial defenses, and fluid and electrolyte balance.12 It is essential to appreciate, however, that even minor, localized burn injuries may be associated with diffuse and dramatic systemic responses. These injuries may have an impact on all of the systems of the body.5 Several mediators released from the burned areas activate the inflammatory response and cause local and remote edema. Complement, arachidonic acid metabolites, and oxygen radicals are involved in this response.6 Cytokines are the key mediators of the systemic effects.13 Abnormal cytokine values reflect the severity of injury, and these abnormalities may persist for years after injury.14–18 Endotoxins are frequently detected in the period immediately after the burn, usually correlate with the burn size, and are predictive of the development of multiple organ failure and the subsequent demise of the patient.19 The clinical symptoms and pathologic changes are relatively more severe in children, and, unfortunately, the gravity of the injury is often underestimated because of their greater ratio of body surface area to weight (Fig. 34-1).
Soon after the injury, massive volumes of fluid shift from the vascular compartment to the burned tissue, resulting in sequestration of fluid, even in nonburned areas of the body, resulting in significant hemoconcentration.5 Despite the massive fluid loss, systemic blood pressure is usually maintained through an outpouring of catecholamines and antidiuretic hormone, both of which are potent vasoconstrictors.20 In the first 4 days after a burn of moderate size or larger (approximately 40% of the body surface area), an amount of albumin equal to about twice the total body plasma content is lost through the wound. In addition to the direct effects of the burn (thrombosis, increased capillary permeability), changes in vascular integrity occur in areas remote from the injury, resulting in tissue edema.21 In the pulmonary capillary network, these changes may be life-threatening; severe pulmonary edema and vascular congestion may result.
Cardiac
Immediately after an injury, cardiac output is dramatically reduced.22,23 This decrease is often related to the rapid reduction in circulating blood volume and the severe compressive effects of circumferential burns on the abdomen and chest that impair venous return.24 Despite adequate cardiac filling pressures, cardiac output often remains reduced. This may result from other factors, such as direct myocardial depression from the burn injury. Some investigators have described circulating myocardial depressant factors such as interleukins, tumor necrosis factors, or oxygen free radicals existing in subjects with extensive third-degree burns.25–28 Transesophageal echocardiography may be helpful in guiding supportive care in the early phase.29 At our institution, acutely burned children frequently require inotropic support during the acute period of depressed cardiac function. Carefully titrated inotropic support improves cardiac output, without the need for volume overload–mediated improvement in cardiac function.
Children develop a hypermetabolic state 3 to 5 days after a burn injury. This state is associated with a twofold to threefold increase in cardiac output, which persists for weeks to months, depending on the extent of the injury and the time needed for wound closure; heart rate, cardiac output, cardiac index, and rate-pressure-product are increased for at least 2 years after burns that involve 40% or greater of body surface area.30 Some children develop a reversible cardiomyopathy.31 Hypertension has been reported to occur during this hypermetabolic period. The most common cause of the hypertension in this period is inadequate pain control, and this should be ruled out. However, other circulating mediators such as increased catecholamines, atrial natriuretic factor, renin-angiotensin, endothelin-1, and vasopressin and other circulating vasoactive mediators can cause intermittent or persistent hypertension in patients with burn injuries.32–36 If decreased cardiac output is observed during this hypermetabolic state, gram-negative sepsis or hypovolemia should be suspected. Closure of the burn wound usually decreases metabolic demand, resulting in a concomitant reduction in cardiac output.37,38 Some children may benefit from treatment with propranolol, which reduces cardiac work and decreases the systemic inflammatory response.39,40
Pulmonary
Pulmonary function may be adversely affected from the upper airway to terminal alveoli. The upper airway is an excellent heat exchanger; just as it warms cold air, it cools hot air. The cooling of hot inspired air may cause a thermal injury to the tissues of the larynx; the air in a closed space (e.g., house or automobile fire) may reach 538° C (1000° F) 2 feet above floor level. Inspiration of superheated air damages the upper airway. Airway obstruction occurs as a result of massive edema formation involving laryngeal and tracheal structures above the carina (see later). The thermal insult also may injure the ciliated epithelium and mucosa in the proximal bronchi. The inhalation of toxic fumes, such as nitrogen dioxide and sulfur dioxide released from burning plastic, which combine with water in the tracheobronchial tree to form nitric and sulfuric acids, may damage the distal bronchi and alveoli. Thus upper airway injury is usually a thermal insult, whereas lower airway injury is a chemical or toxic insult. Acid gases such as hydrochloric acid, sulfuric acid, and phosgene form small aerosolized particles that penetrate deep into the tracheobronchial tree, damaging the alveolar membranes and surfactants.41 Wool and cotton combustion forms aldehydes, which may cause pulmonary edema in concentrations as small as 10 ppm.42 Combustion of synthetic materials (insulation, wall paneling) releases hydrogen cyanide. Although cyanide is a rare toxin in fires,43 cyanide poisoning can lead to histotoxic hypoxia and death,44 mimicking carbon monoxide (CO) poisoning. Inhalation of hydrogen cyanide is an often unrecognized cause of immediate death.
The overall effect of a pulmonary inhalation injury is necrotizing bronchitis, bronchial swelling, alveolar destruction, exudation of protein, loss of surfactant, loss of the protective bronchial lining, and bronchospasm, all of which contribute to the development of bronchopneumonia (Figs. 34-2 and 34-3). Inhalation of particulate matter (smoke, soot) and lower airway edema also obstruct the airway mechanically. Edema of the bronchi, combined with loss of integrity of the pulmonary capillary endothelium, decrease pulmonary compliance. Circumferential chest burns may have a tourniquet-like effect that decreases chest wall compliance.24 All of these injuries lead to clinically important ventilation-perfusion abnormalities and right-to-left intrapulmonary shunting, with hypoxemia and hypercarbia. In adults, the Pao2/Fio2 ratio and baseline carboxyhemoglobin concentrations are predictive of mortality.45 One pediatric trial of inhaled heparin and acetylcysteine suggested a benefit with decreased airway cast formation and mucus plugging.46 However, subsequent studies have yielded contradictory results.47,48 CO inhalation can further compromise both hemoglobin’s oxygen (O2)-carrying capacity and its ability to deliver O2 to tissues. CO also impairs O2 usage at the cellular level (cellular respiration). Severe smoke inhalation alone may occur without externally visible injuries.49 One clue that smoke inhalation has occurred is the presence of singed nasal hairs or nasal passages. Extrapulmonary factors such as changes in cardiac output also can contribute to hypoxemia. Blood gas analysis alone may not indicate these factors. Rational therapy of arterial O2 desaturation requires evaluation of both extrapulmonary and intrapulmonary factors,21,50 including cardiac output, mixed venous O2 content or saturation, and shunt fraction.22 In general, the prognosis of cutaneous burn is compounded by the presence of an inhalation injury; the presence of an inhalational injury doubles the mortality rate from cutaneous burns,41 with pediatric mortality reported to be approximately 16%.51
Renal
Renal function may be adversely affected soon after injury, primarily as a result of myoglobinuria and hemoglobinuria.52 The former is most common after electrical injury,53,54 whereas the latter is common after severe cutaneous burns of 40% or greater of body surface area. Hypovolemia, hypotension, and hypoxemia may further aggravate renal dysfunction, leading to acute tubular necrosis. Catecholamines, angiotensin, and vasopressin production increase, leading to systemic vasoconstriction, compounding the renal insufficiency.55,56 Release of vasoactive peptides such as endothelin-1 may cause acute vasoconstriction, which also may adversely affect renal function.57,58 Fluid retention is common during the first 3 to 5 days after injury and is followed by diuresis thereafter. Thus renal function may be impaired soon after the injury, delaying the excretion of some drugs or their active metabolites. At 3 to 7 days after the burn injury, glomerular filtration rate increases pari passu with an increased cardiac output and metabolic rate.59 The serum half-life of many antibiotics and other medications that depend on renal excretion may be altered as a result of changes (increased or decreased) in the glomerular filtration rate.60–64 Children who sustain a burn that covers greater than 40% of their body surface area demonstrate renal tubular dysfunction, in the form of an inability to concentrate the urine.20 Even during hyperosmolar states, antidiuresis is not observed, suggesting an inadequate renal response to antidiuretic hormone and aldosterone. Thus an adequate urine output may be observed even in the presence of hypovolemia.65 Episodic or persistent hypertension is frequent in children, in part mediated by an increase in renin and catecholamine production.66,67 If hypertension persists, treatment that decreases stress on the cardiovascular system or reduces the potential for hypertensive encephalopathy should be instituted.
Hepatic
The liver may be damaged by hypoxemia or hypoperfusion during the early postburn phase as a result of inhaled or absorbed chemical toxins, hypovolemia, or hypotension.68,69 Reperfusion injury may harm the liver when adequate circulation is reestablished. Delayed hepatic dysfunction may result from drug toxicity, sepsis, the hypermetabolic response to burns, or blood transfusions.70 Studies in adults have found increased hepatic blood flow, increased protein synthesis and breakdown, and increased hepatic gluconeogenesis during the hypermetabolic phase of burn injury. With the onset of sepsis, hepatic glucose output and alanine uptake may decrease sharply but hepatic blood flow and O2 usage can remain increased.68,71 Fatty infiltration of the liver also has been reported.72 Sustained increases in hepatic blood flow deliver more drug to the liver; this effect, combined with drug-induced enzyme induction, may decrease the half-life of drugs that are perfusion limited.73 Although all studies of animals suggest decreased clearance of drugs after burn injury, clinical studies of the capacity of the liver to metabolize drugs are conflicting, even for the same class of drugs.74–79 The magnitude of the burn, the time after injury, and the effects of co-administered drugs, alone or in combination, as well as alterations in protein binding and volume of distribution, may have a role in these conflicting reports.
Central Nervous System
The central nervous system (CNS) may be adversely affected by inhalation of neurotoxic chemicals or by hypoxic encephalopathy; other contributing factors include sepsis, hyponatremia, and hypovolemia.80 CNS dysfunction includes hallucinations, personality changes, delirium, seizures, abnormal neurologic symptoms, and coma.81 These effects may be due to the burn injury or to the administration of drugs necessary for sedation, anxiolysis, and analgesia.82 Such effects usually clear after several weeks. Abnormalities of CNS neurotransmitters have been postulated to mediate the anorexia associated with extensive burn injury.83 The possibility of cerebral edema and increased intracranial pressure also must be considered during the initial phases of burn injury. Under such circumstances, the usual measures for treating increased intracranial pressure would be instituted (see Chapter 24). Data suggest that rapid overcorrection of hyponatremia also may be associated with cerebral injury.84
Hematologic
Blood viscosity may increase as a result of hemoconcentration secondary to fluid shifts and because of alterations in plasma protein content.85 The hematopoietic system is also adversely affected. Ongoing microangiopathic hemolytic anemia secondary to the burn injury is common.86 An inhibitor of erythroid stem cells has been found in the sera of burn patients, which may in part contribute to the anemia of burn injury. Another study has demonstrated a normal erythropoietin response to anemia in patients with burn injury.87 The half-life of red blood cells is diminished in burn patients, and multiple blood draws may contribute to the development of anemia.88,89 The possible role of recombinant erythropoietin in the care of children with burns has yet to be defined.90,91 One study in adults reported no reduction in either mortality or blood transfusion requirements in patients who received recombinant erythropoietin compared with controls.92
In the early stage, thrombocytopenia secondary to increased platelet aggregation and trapping of platelets in the lungs is followed by an increase in platelet count 10 to 14 days after the burn injury. A prolonged period of thrombocytopenia and reduced nadir in platelet count compared to survivors are both associated with increased mortality.93 This thrombocytopenia may persist for several months.89,93 An increase in fibrin split products (disseminated intravascular coagulopathy), which lasts for 3 to 5 days, may occur.62 Factors V, VII, and VIII and fibrinogen are also increased several-fold over baseline for the first 3 months after severe injury uncomplicated by sepsis.94,95 Children with increased platelet counts (>1 million/mm3) who then developed sepsis in our unit experienced a marked decrease in the platelet count. The sudden onset of thrombocytopenia should prompt an evaluation of the child for sepsis.96 Likewise, large swings in the fibrinogen concentration can occur (up to 2 g/dL),97 although these do not appear to herald an increase in the incidence of thrombotic events.
Gastrointestinal
Gastrointestinal function is diminished immediately after thermal injury secondary to the onset of gastric stasis and intestinal ileus.98 Because of the risk of pulmonary aspiration of gastric contents during this time, the stomach should be adequately vented and appropriate gastric acid ulcer prophylaxis instituted. At 48 to 72 hours after a burn injury, when generalized edema is resolving, gastrointestinal function usually resumes. Enteral feeding should be established at this time to provide calories, to blunt the hypermetabolic response, and to attenuate gluconeogenesis and stress ulceration.85,99,100 Early enteral feeding has the added advantages of diminishing muscle catabolism, reducing bacterial translocation through the intestinal mucosa and is associated with reduced mortality.101–104
In children who do not tolerate enteral feeding, parenteral nutrition must be initiated.99,105–107 Stress ulcers (Curling ulcers) are associated with any burn injury and may be life-threatening, although the incidence has decreased in critically ill patients, in part because of improved pharmacologic control of gastric acidity.108 Prospective studies of pediatric and adult burn patients and patients in intensive care indicate that cimetidine or ranitidine in the usual doses does not adequately protect critically ill patients from increases in gastric acidity.60,61 The increased requirement for drugs is due to differences in their pharmacokinetics.60 Therefore frequent feedings when tolerated and the liberal use of antacids, combined with larger or more frequent doses of H2-receptor antagonists (or proton pump inhibitors), may be required to prevent development of stress ulcers.60,85,98
Endocrine
The endocrinologic response to acute thermal injury is complex, involving most organ systems. Stimuli that trigger endocrine responses include the thermal injury itself and subsequent fluid shifts, as well as the stress responses that are associated with critical illness.109 These may include decreased circulating hormone concentrations (e.g., triiodothyronine, dehydroepiandrosterone, and testosterone), as well as increased concentrations of other hormones (antidiuretic hormone, catecholamines, renin, angiotensin II, and cortisol).110 Replacement therapy with synthetic androgenic steroids (e.g., oxandrolone) shortens acute hospital stay and improves body composition (lean body mass) and hepatic protein synthesis.111,112 Glucose control may be poor, owing to the increased levels of cortisol and insulin resistance.113 Tight control of hyperglycemia may improve mitochondrial oxidative capacity,114 decrease the incidence of urinary tract infection, and improves the survival of critically ill burn patients, although the last finding resulted from a single study.115,116 Avoiding hyperglycemia may attenuate the risk of cerebral injury from hypoperfusion states (see Chapters 24 and 39); one group recommends a target blood glucose level of 130 mg/dL.116 Blocking the renin-angiotensin system may improve the insulin response after burn injury.117 It should be noted that insulin resistance might persist for 6 to 9 months after discharge.114
Skin
Extensive skin destruction results in the inability to regulate body heat, conserve fluids and electrolytes, and protect against bacterial invasion. Permeability of burned tissues is markedly increased and proportional to the number of layers of tissue damaged.118 Because children have a much greater ratio of body surface area to weight compared with adults, they are even more likely to become hypothermic (see Fig. 34-1). Thus it is important to keep these children covered as much as possible, to increase the environmental temperature, and to use radiant warmers, plastic wrap around extremities, reflective insulated blankets, artificial “noses” (in-line moisture and heat exchangers), and hot-air heating blankets. Late complications affecting the skin include progressive scar formation, which results in movement-restricting contractures.24,119 Topical antibiotic and antibacterial therapy is necessary to prevent burn wound sepsis.120–125
Metabolic
Many metabolic alterations follow extensive burn injury. Increased usage of glucose, fat, and protein results in greater O2 demand and increased carbon dioxide (CO2) production.* Mediators that have been implicated in these metabolic changes include interleukin-1, tumor necrosis factor, catecholamines, prostanoids, and other stress hormones.2,133 Centrally mediated or sepsis-induced hyperthermia also increases O2 consumption and CO2 production. Some of these abnormalities may persist even after complete closure of the burn wounds, when metabolic demand is already reduced.† Intravenous alimentation, particularly with increased glucose concentrations, may also increase CO2 production and therefore increase ventilatory requirements.105 Increase in O2 demand135 and CO2 production must be compensated for during controlled mechanical ventilation. Treatment of fever reduces metabolic demand.136
Calcium Homeostasis
The ionized calcium concentrations in many acutely burned patients are dramatically decreased. Marked abnormalities in the indices of calcium and magnesium metabolism, including hypoparathyroidism in both acute and recovery phases, may persist for weeks after injury (E-Fig. 34-1).137,138 One study suggested that short-term therapy with pamidronate, a drug that inhibits bone resorption, conserves bone mass after burn injury in children.139 Hypophosphatemia and hypermagnesemia revert toward normal during the latter phase of recovery from the acute injury. The usual reciprocal relationship between calcium and inorganic phosphate is not evident in those with major burns. Therefore supplemental calcium therapy is extremely important, particularly when rapid colloid infusions are required intraoperatively during burn surgeries, because ionized hypocalcemia dramatically impairs cardiovascular homeostasis. In general, frequent small boluses of calcium are safer and more effective than intermittent large boluses (see also Figs. 10-8 and 10-9).140 Doses of 2.5 mg/kg calcium chloride or 7.5 mg/kg calcium gluconate ionize at equivalent rates and produce equivalent increases in calcium concentration. After recovery from burn injury, vitamin D supplements are strongly recommended to offset the decreased conversion of 7-dehydrocholesterol to previtamin D3.141
Psychiatric
It is imperative to recognize that physical trauma is not the only trauma sustained by the pediatric burn patient; psychological trauma and its associated long-term sequelae is also common.142,143 A large percentage of acutely burned children present with acute stress or develop posttraumatic stress disorders.142–145 Risk factors for developing acute stress disorders include the size of burn, the degree of pain, the pulse rate, and parental issues.146 Treatment with fluoxetine or imipramine may ameliorate these stress disorders,147–149 although one randomized controlled study found no difference from placebo.150 Another study found risperidone to be of value in reducing stress symptoms.151 There is an increased incidence of attention-deficit disorders in pediatric burn patients, likely owing to impulsivity.152,153 Finally, the normal psychosocial support network may be impaired in the families of the burn patient, even before the burn injury.154–156
Pharmacology
Subsequent to any major thermal injury, many physiologic changes occur that have an impact on the disposition of drugs in the body. During the hypovolemic period, uptake and clearance of drugs may decrease because of impaired organ perfusion.2,73–76,157 During the hypermetabolic phase, the activity of organs that clear drugs from the circulation (e.g., the liver and kidneys) may be enhanced because of enzyme induction and increased blood flow to those organs.* The massive volume of edema present and the loss of drug through burn wounds can increase the central or total volume of drug distribution.162
Many drugs are highly bound by plasma proteins. The activity of such drugs depends primarily on the unbound rather than total drug concentrations, and small changes in the unbound fraction may have a dramatic effect on the response to the drug. The two major binding proteins, α1-acid glycoprotein and albumin, increase and decrease, respectively, after burn injury, resulting in either decreased or increased free fractions of those drugs to which they bind.73,161 For example, the clearance of morphine and meperidine is enhanced or impaired, depending on the size of the burn, with a trend to reduced morphine or meperidine clearance after large burns compared with moderate burns. In general, burned children clear drugs more readily than those without burn injuries.75–77,162–164 Similarly, pharmacokinetic studies of lorazepam and diazepam indicate that the clearance of the former is increased whereas that of the latter decreases.78,79 In the case of oral ketamine, the pharmacokinetics were unaffected in children with small burns.165
Evidence indicates that burn injury, with its complications and hormonal responses, may affect the number of receptors in tissues.78,83,157,161,166–173 Therefore reports of aberrant responses to drugs acting on adrenergic and cholinergic receptors are not surprising. These include altered sensitivity to succinylcholine at the neuromuscular junction, increased sensitivity to dopamine in the pulmonary circulation, and decreased sensitivity to nondepolarizing neuromuscular blocking drugs.73,166,168–170,174–176 Other examples of drugs affected by burn-induced kinetic and dynamic changes include aminoglycoside antibiotics, diazepam, and cimetidine. Burn-induced alterations in kinetics and dynamics make the clinical response to any medication unpredictable. Therefore clinical effects should always be closely monitored and plasma concentrations, protein binding, and clearance evaluated whenever possible.60,63,73,177–181 Dexmedetomidine (see later) also may have altered pharmacodynamics in the burned child. Because of the known hypotensive effects of the α2a-adrenoceptor agonists, particular attention to limit the dose and to ensure euvolemia may minimize the hemodynamic consequences.
Resuscitation and Initial Evaluation
Airway and Oxygenation
Every burn patient, especially those with inhalation injuries, must be considered hypoxemic and exposed to carbon monoxide (CO). Therefore during transport to the hospital and on admission, administration of high inspired concentrations of O2 is mandatory, pending evaluation of the severity of CO poisoning and pulmonary injury (see later).181 Direct injury to the airway and alveoli occurs in children who have sustained pulmonary injury from the inhalation of smoke, flames, noxious gases, heated air, or steam.42,51,133,182–211 When a child is burned in an enclosed space (house, automobile) or if thermal burns or carbonaceous materials are evident about the mouth and nose, inhalational injury is probable.192,212 Upper airway obstruction caused by edema of the lips, nose, tongue, pharynx, glottis, and subglottis is very common. The resultant airway obstruction can be compared with the combined effects of acute macroglossia, epiglottitis, macro uvula, and laryngotracheobronchitis. The decreasing patency of the airway resulting from rapidly increasing edema, beginning in the first hours after the injury and lasting several days, makes delayed intubation hazardous if not impossible (Fig. 34-4). Prophylactic intubation should be performed in any case of severe facial burns or when pulmonary burn and upper airway inhalation injury are suspected. The severity of adverse outcomes is related to the presence or absence of inhalation injury.51,200–211,213
Control of the airway in children is usually accomplished under general anesthesia. In the case of pure inhalational injury without facial or upper airway burns, the need for intubation should be considered on a case-by-case basis. Early clinical experience at our hospital showed that tracheal tubes could be left in place in these children for weeks with fewer risks than the alternative, tracheostomy.214,215 Tracheostomy in thermally injured children was associated with high mortality rates; in one pediatric series the death rate approached 100%.216 However, in recent years there has been a move back to performing tracheostomy for children who are expected to require long-term ventilatory management. Although one review of burn centers in North America found that the practice of performing a tracheostomy in burned children was variable, there has been increasing tendency to perform a tracheostomy in children over age 7. 217 Some report that an early tracheostomy secures the airway and reduces the risk of subglottic stenosis.218,219 When early airway instrumentation is indicated, a cuffed tracheal tube is preferred to reduce the need for changing the tube to deliver high peak inspiratory pressures should they be required.220 Traditionally, cuffed tracheal tubes have not been used in children younger than 8 years. This practice may not be necessary with proper attention to leak pressures (pressure at which gas can be heard “leaking” around the tracheal cuff by auscultating over the trachea while administering positive pressure) or the use of the MICROCUFF (Kimberly-Clark, Roswell, Ga.) tube (see later), although long-term studies with these tubes are needed (see Chapter 12).221,222 We routinely use cuffed tracheal tubes, appreciating the added flexibility they offer in not requiring replacement because of decreasing edema (with associated increased leak if uncuffed tubes were used). Another important means of minimizing barotrauma is the use of permissive hypercarbia.223 New tracheal tubes that are designed to have the cuff located more distally and made of thinner material (MICROCUFF) may also reduce the potential for airway injury (see also Figs. 12-15 and 12-17), although in the hot environment of a burn unit they may have a greater tendency toward kinking.224–228
Carbon Monoxide Poisoning and Cyanide Poisoning
The majority of smoke inhalation victims have CO poisoning. Direct measurement of carboxyhemoglobin (COHb) is an important guide to the adequacy of treatment. Estimates of the COHb concentration may be derived by measuring (not calculating) O2 saturation or arterial O2 content. The half-life of COHb is approximately 5 hours when the patient is breathing room air but decreases to 90 minutes when 100% O2 is administered.229,230 Immediate administration of O2 is therefore essential to achieve the maximum possible level of O2 in the blood; positive-pressure ventilation may be indicated in severe cases.231–233 Hyperbaric oxygen may be a useful adjunct for this purpose (see later).
Standard pulse oximeters do not differentiate between oxyhemoglobin and COHb; in contrast, transcutaneous O2 analyzers and cooximeters are useful.234 Thus pulse oximetry cannot be used to accurately monitor the oxygenation of patients with CO poisoning because COHb produces an overestimation of O2 saturation; the photo detector is “fooled” into interpreting COHb as oxyhemoglobin.234–236 A more recent eight-wavelength pulse oximeter capable of measuring COHb and methemoglobin may prove useful in the management of burn patients237,238; however, the sensors are quite expensive and likely should be reserved for use in those with an established diagnosis, unless arterial blood gas analysis is not immediately available.239
COHb is produced by the combination of CO with the iron of the heme radical at the O2-binding site. CO combines more slowly with hemoglobin than O2 but is bound 200 times more firmly.240,241 Inhalation of 1% CO for 2 minutes can result in COHb values of 30% (E-Fig. 34-2).242 The toxic effects of CO poisoning are due to tissue, organ, and cellular hypoxia from decreased O2 delivery. Decreased delivery occurs because CO reduces O2 binding capacity both to the hemoglobin molecule at the tissue level and to cytochromes in the respiratory chain at the cellular level; even in small amounts, COHb shifts the O2-dissociation curve to the left (E-Fig. 34-3), thus reducing release of O2 from hemoglobin.42,181,231,241–245 For example, if an individual had 40% carboxyhemoglobin, this would reduce O2 carrying capacity from 20 mL/100 g of hemoglobin to 12 mL/100 g and with the leftward shift further compromise O2 delivery.
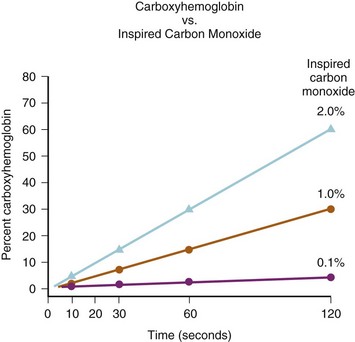
E-FIGURE 34-2 Note the rapid rise in carboxyhemoglobin values when just 1% carbon monoxide is inhaled.
The evidence supporting the use of hyperbaric oxygenation (HBO) therapy as an adjunct therapy for burns remains controversial.85,246–250 A Cochrane review of six randomized controlled trials with 1361 patients concluded that at present insufficient data exist to demonstrate reduced adverse neurologic outcomes with HBO therapy and that additional research is needed to “better define the role, if any, of HBO in the treatment of patients with CO poisoning.”251 The most common indication for hyperbaric therapy in burned children is concomitant CO poisoning.42,252,253 As previously mentioned, CO binds avidly to hemoglobin254 and other iron-containing enzymes, such as intramitochondrial cytochromes, interfering with the delivery and usage of O2, respectively.255,256 Children suffering significant CO exposure are at risk of developing both acute and delayed neurologic sequelae. The pathophysiology of neurologic sequelae is not known, although imaging studies suggest a potentially reversible demyelinating process.257,258 Data supporting the use of hyperbaric O2 to prevent and treat these complications may be weak259–266 but cannot be discounted, given the seriousness of these sequelae.248,249,267,268
The important practical question is whether hyperbaric treatment will decrease the frequency and severity of delayed neurologic sequelae in burned children with concomitant CO poisoning. This is a difficult question because the incidence of delayed sequelae is unknown and determining the severity of an individual exposure is often not possible. Delayed sequelae include headaches, irritability, personality changes, confusion, memory loss, and gross motor deficits. The frequency with which those exposed develop symptoms is unknown, although they are reported to occur in approximately 10% of patients with serious exposures.243 A symptom-free interval of several days is commonly reported. Delayed hyperbaric treatment may relieve symptoms, and spontaneous resolution of delayed sequelae may be expected in up to 75% of patients within 1 year.269–275 The severity of the CO poisoning is often difficult to pinpoint because there is a poor correlation between serum COHb and degree of CO exposure.276,277 Neuropsychiatric testing has been proposed as a more accurate way to determine this,229 but such detailed examinations are difficult in burned children secondary to pain medications and hemodynamic instability. Some clinicians believe that a history of unconsciousness indicates that an exposure has been severe enough to warrant treatment.271,278–280 However, the relatively few randomized prospective studies evaluating this have returned conflicting results.262,266 Hyperbaric O2 treatment is not without expense, inconvenience, and risk, and the indications for treatment of burned children with concomitant CO poisoning are debated.256,281 One study described complications during treatment of a heterogeneous groups of patients: emesis (6%), seizures (5%), agitation requiring restraints or sedation (2%), cardiac dysrhythmias or cardiac arrests (2%), arterial hypotension (2%), and tension pneumothorax (1%).256 Complications may be expected more frequently in the critically ill.282
Cyanide toxicity may occur in inhalational burn injuries as well.283 If cyanide poisoning is confirmed in the child’s blood, administration of hydroxycobalamin or sodium thiosulfate, alone or in combination, is warranted (see Chapter 10).284 HBO therapy has been shown to facilitate movement of cyanide out of tissues and into blood, thereby potentially facilitating treatment,283 although the use of HBO for treatment remains investigational.
Adequacy of Circulation
The various formulas for determining fluid replacement are estimates and often need modification, depending on clinical and laboratory findings.* The most widely accepted fluid protocols in current use are the Parkland (Baxter) and Brooke formulas. All formulas and guidelines for fluid therapy require modification according to the individual child, depending on the child’s response (Table 34-1).6 The most important metric of fluid homeostasis remains a good urine output (0.5 to 1 mL/kg/hr).
Both formulas provide estimates of the fluid volume required for resuscitation, in addition to the calculated normal maintenance fluid requirement for each day. These formulas are of great value in guiding the fluid resuscitation of older children; however, serious underestimation of the fluid volume may occur if applied to infants weighing less than 10 kg. In such infants, it is reasonable to estimate the normal hourly maintenance fluid requirements and then add to this the fluid volume of the Parkland or Brooke formula.2 Alternatively, the crystalloid fluid regimen for resuscitation can be increased to 6 mL/kg × the percent surface area burn per 24 hours.297,298
The degree of edema depends on the volume and composition of the resuscitation fluid administered. Consequently, colloids or hypertonic saline (with or without albumin) are used in some burn centers during early burn wound resuscitation.299 These modified regimens have been shown to be particularly effective in the very young and the elderly.† The purported advantage is less tissue edema. It is of interest that a Cochrane review of 15 studies using hypertonic saline in 614 patients found that less intravenous fluid was needed for resuscitation and higher sodium values occurred, although the overall morbidity and mortality was unchanged.301
A growing practice in burn programs has been to begin the fluid resuscitation with colloid, usually 5% albumin, early during resuscitation of seriously burned children.302 No consensus has been reached on such a colloid protocol.303 In seriously burned children in our unit, we begin with a 5% albumin solution at a maintenance rate immediately on admission. We administer an amount equal to that of their calculated crystalloid requirements, tapering the crystalloid first, and continuing albumin fusion for 48 hours. Further work is required before advocating hypertonic fluid regimens in burned children routinely.304
The syndrome of hyperosmolar hyperglycemic nonketotic coma (severe dehydration, marked hyperglycemia, serum hyperosmolality, and coma in the absence of ketoacidosis) may be associated with burns. Avoiding this syndrome is critically important because it carries a high mortality.285 Glucose-containing solutions should be restricted at all times, particularly during the initial volume resuscitation. Serum glucose concentrations should be measured frequently during this period. Blood glucose concentrations should be managed with insulin with a target blood glucose of 130 mg/dL.116
The general appearance of the child and his or her sensorium provide important guides to the effectiveness of the resuscitative therapy. In addition, urine output is a useful metric to determine the need for additional fluid administration, recognizing that antidiuretic hormone secretion may be increased and renal tubular dysfunction may be present.20,158 Every effort must be made to protect kidney function by providing adequate fluid replacement.2,304 Renal failure in the presence of a major burn is usually lethal. However, overly aggressive fluid administration may induce pulmonary and tissue edema. Therefore, when a burned child is being volume resuscitated, all fluids to replace the circulating blood volume must be carefully titrated. Commonly used end points of satisfactory fluid resuscitation include heart rate, systemic arterial blood pressure, urinary output, central venous pressure, arterial oxygenation, and pH. Cardiovascular function estimated by transthoracic or transesophageal echocardiography29 and technetium-99m ventriculography may be of value in critically ill patients.305,306 These advanced cardiac evaluations are not required in most children; they are mostly useful in the presence of cardiovascular compromise and a need to consider the use of a vasopressor or additional volume loading.
In a child, the evaporative fluid losses exceed 4000 mL/m2 of burn surface each day, compared with only 2500 mL/m2 in an adult.290 Concomitantly, for each square meter of burn surface, 2500 to 4000 kcal of heat is lost each day. Minimizing caloric expenditure and providing caloric supplementation simultaneously are the only ways to minimize catabolism of body tissues. The tendency for children to be poikilothermic, particularly in the absence of protective skin as a result of the burn injury, causes profound temperature derangements. Efforts to maintain a normal body temperature are essential in both the operating room and the intensive care unit. These measures are especially important during the initial volume resuscitation and in the operating room when dressings are removed for examination and excision (Fig. 34-5).
Circumferential Burns
Adverse cardiovascular and respiratory responses are immediate to circumferential burns of the chest, abdomen, and extremities.2,24,196,197 Circumferential burns of the thorax can restrict respiratory effort, resulting in respiratory failure as a result of decreased chest wall compliance. Functional residual capacity is reduced with airway closure and atelectasis, resulting in profound hypoxemia.42,183–187,190–198,307 Deep circumferential burns of the chest and abdomen may generate excessive intrathoracic and intraabdominal pressure, which, in addition to restricting diaphragmatic movement, may further reduce the already decreased cardiac output by impairing venous return (Fig. 34-6).24,196,197 When this occurs, both extrapulmonary and intrapulmonary factors can contribute to arterial desaturation.50
The edema of damaged tissues also can generate severe compressive forces, restricting or occluding the blood flow to burned extremities. The net result may be ischemia of the limb, which if left untreated, may lead to partial or total amputation. Escharotomies of circumferential burns of the chest, abdomen, and extremities must be performed urgently because impaired hemodynamics and respiratory mechanics can cause irreversible damage within hours of the burn injury. Escharotomy is often undertaken without the need for general anesthesia because a full-thickness burn usually destroys skin innervation. Abdominal compartment syndrome may develop in children who require large volume resuscitation. To detect any evolving compartment syndrome, some burn centers advocate routine monitoring of bladder pressure.307,308
Electrical Burns
Electrical burns occur with household voltage (electric cords and sockets) and nonhousehold high-voltage current (power line or lightning). Children often disconnect extension cords by stabilizing one end in their mouths and pulling the other end with a hand, resulting in circumoral and lingual burns.4,53,309,310 High-voltage injuries are often associated with loss of limbs and other injuries that are not immediately obvious.311–314 The extent of this injury is unpredictable. The surface injury is often small, but the extent of underlying tissue damage and necrosis is massive. Such an injury is a combination of electrical and thermal damage.314,315 Victims often have concurrent injuries such as fractures of vertebrae or long bones, ruptured organs, myocardial injury, or numerous contusions. Even children with low-voltage injuries may have abnormalities of cardiac conduction.312 Children with electrical burns may be comatose or have sustained seizures at the time of admission to the hospital. Muscle tissue adjacent to bone is usually more damaged than superficial muscles because bone is a poor conductor of electrical current, and therefore heats up when high currents are passed through it, resulting in damage to the muscles surrounding bone. Early fasciotomy may be needed to preserve the blood flow to extremities (Fig. 34-7). Myonecrosis necessitates general anesthesia during the first day of injury at the time when fluid shifts, hyperkalemia, and myoglobinuria are maximal. Massive myonecrosis and hemolysis may result in hyperkalemia, as well as myoglobinuria and hemoglobinuria. In the presence of hemoglobinuria or myoglobinuria, increased fluids and mannitol will ensure a continuous urine output (>1 mL/kg/hr).316,317 Alkalization of the urine may prevent these proteins from precipitating in the renal tubules. Follow-up of patients with electrical injuries often reveals unpredictable sequelae, which may manifest months to years later. These injuries may occur in organs or areas that do not appear abnormal during the acute course of illness. These late complications most frequently include neurologic dysfunction, ocular damage, damage to the gastrointestinal tract, circumoral strictures, changes in the electrocardiogram, and delayed hemorrhage from large vessels.315,317
Guidelines to Anesthetic Management
Anesthetic management of children with severe thermal injury begins with the initial resuscitation and continues for many years through reconstructive surgery. Knowledge and understanding of the pathophysiology of burn injury enable anesthesiologists to plan appropriate anesthetic management and recognize and treat complications arising as a result of burn injury or its therapy (Table 34-2).2,12,318
System | Early Effects | Late Effects |
---|---|---|
Cardiovascular | ↓ CO as a result of decreased circulating blood volume, myocardial depressant factor | ↑ CO as a result of sepsis ↑ CO 2 to 3 times > baseline for months (hypermetabolism) Hypertension secondary to vasoactive substances such as renin |
Pulmonary | Upper airway obstruction as a result of edema | |
Lower airway obstruction as a result of edema, bronchospasm, particulate matter, sloughing of airway mucosa | ||
↓ FRC | Bronchopneumonia | |
↓ Pulmonary compliance | Tracheal stenosis, vocal cord granuloma | |
↓ Chest wall compliance | ↓ Chest wall compliance | |
Renal | ↓ GFR secondary to | ↑ GFR secondary to ↑ CO |
↓ circulating blood volume | ||
Myoglobinuria | Tubular dysfunction | |
Hemoglobinuria | ||
Tubular dysfunction | ||
Hepatic | ↓ Function as a result of ↓ circulating blood volume, hypoxia, hepatotoxins | Hepatitis |
↑ Function as a result of hypermetabolism, enzyme induction, ↑ CO | ||
↓ Function as a result of sepsis, drug interactions | ||
Hematopoietic | ↓ Platelets | ↑ Platelets |
↑ Fibrin split products, consumptive coagulopathy, anemia | ↑ Clotting factors | |
Neurologic | Encephalopathy | Encephalopathy |
Seizures | Seizures | |
↑ ICP | ICU psychosis | |
Skin | ↑ Heat, fluid, electrolyte loss | Contractures, scar formation, difficult IV access, difficult intubation |
Metabolic | ↓ Ionized calcium | ↑ Oxygen consumption |
↑ Carbon dioxide production | ||
↓ Ionized calcium | ||
Pharmacokinetics | Altered volume of distribution | Tolerance to opioids, sedatives |
Altered protein binding | Enzyme induction, altered receptors | |
Altered pharmacokinetics | Drug interaction | |
Altered pharmacodynamics |
↓, Decrease in; ↑, increase in; AIDS, acquired immunodeficiency syndrome; CO, cardiac output; FRC, functional residual capacity; GFR, glomerular filtration rate; ICP, intracranial pressure; ICU, intensive care unit.
Keeping children with severe burn injuries on nothing by mouth (NPO) status for 8 hours or longer before sedation for a dressing change or anesthesia for a surgical procedure severely compromises caloric intake; therefore we advocate the use of continuous orojejunal or nasojejunal alimentation. Generally children can receive calories up to about 4 hours before sedation or induction without fear of significant gastric residual volumes. Feeding can be resumed almost immediately after the procedure. In children with large injuries who will quickly develop a negative nitrogen balance with cessation of enteral feedings, short-term use of parenteral protein-sparing support is justified and safe.319
Adequate sedation and pain control are necessary before moving children to the operating room. This move is painful, both physically and emotionally, for the child. Intravenous opioids, such as fentanyl, which has minimal histamine release, are particularly helpful. Intravenous midazolam is also helpful for its sedative and amnestic properties. Drug doses should not be based on standard doses used in children without thermal injury. Burned children develop tolerance to most opioids and sedatives, thus requiring increasing doses over time to achieve a satisfactory clinical response.320,321 The dose of sedative or opioid should be titrated to effect while the child is carefully observed and monitored. Children with burn injuries rapidly develop tolerance. It is not unusual for children with burns over greater than 25% of the body surface to require 1 mg/kg/hr of both morphine and midazolam to provide adequate analgesia and sedation.
It is critically important to minimize heat loss and maintain normothermia. This is may be difficult to achieve because of the massive evaporative heat loss that occurs through open wounds. Operating room temperatures during extensive excisions are commonly maintained near 98.6° (37° C).133 Attention must be paid to minimize heat loss both during transport and in the operating room. Multiple blankets or thermal reflective covers are helpful. Special equipment is used to maintain body temperature, including a warming blanket, radiant warmer, blood warmer, and heat/moisture exchangers and forced hot air warmers. Simply wrapping the extremities in sterile plastic bags and covering the head with plastic or thermal insulation material markedly reduces heat and fluid losses (see Fig. 34-5). Although a hot operating room is uncomfortable for staff, maintaining the child’s temperature may be helpful in maintaining normal blood clotting. Each calorie that does not have to be spent to maintain body temperature is one more that can be used in the healing process.
Adequate monitoring for major blood loss and fluid shifts includes arterial and central venous cannulas, a urinary catheter, an electrocardiograph, a pulse oximeter, a capnograph, and an esophageal stethoscope. A secure intravenous route for volume infusion is essential. If the potential for rapid blood loss exists, multilumen catheters may not be adequate because of their high-flow resistance. Rapid infusion devices may be particularly helpful (see Chapter 51).322–324 The femoral vein is an alternative cannulation site, in addition to the internal jugular and subclavian veins (see Chapter 48).
Invasive arterial and central venous pressure monitoring may be established after induction of anesthesia in most children. Propofol, thiopental (if available), or ketamine in incremental doses is usually well tolerated, provided the children are not hypovolemic (onset of effect is noted by lateral nystagmus. (See Video 6-1: Nystagmus after Ketamine Administration.) Studies in children long recovered from acute burn injury found a 40% increase in the thiopental dose needed to ablate the lid reflex, compared with children without burn injury (Fig. 34-8).325 Our experience suggests that the clinical response to propofol appears to be equally shifted to the right; however, clinical studies are lacking. Ketamine may, on occasion, be preferred if the adequacy of intravascular volume is in question or if invasive monitoring lines must be inserted before anesthetic induction; tolerance to ketamine with repeated administration has been reported.326 High-dose fentanyl or morphine combined with nitrous oxide (N2O) for those children who will undergo ventilation postoperatively is also an acceptable anesthetic technique. In general, an inhalation agent is titrated to clinical effect to supplement the opioid-based anesthetic. When using large bolus doses of fentanyl, chest wall rigidity is a possibility. A slow inhalation induction is preferable for children with a compromised airway, bearing in mind the potential for cardiovascular depression.327
Succinylcholine is contraindicated in burned children because of a potentially lethal efflux of potassium ions from muscle.166,169,172 However, within the first 24 hours after a burn, succinylcholine can be used without apparent risk of triggering a hyperkalemic response. This abnormal response first appears 24 to 48 hours after the burn and continues for an indeterminate, but prolonged, period. Some have suggested that the hyperkalemic response continues until all areas of burn have been covered by scar tissue, although this is not evidence-based. Because the end point for succinylcholine-induced hyperkalemia is unknown, we advise avoiding succinylcholine in children with large burns (≥40%) for at least 1.5 years after the burn. The smallest burn reported to trigger a hyperkalemic response was a 9% burn. This abnormal response occurs because the entire muscle membrane, rather than just the myoneural junction, is occupied by acetylcholine receptors. The muscle tissue of burn victims also demonstrates resistance to nondepolarizing muscle relaxants.169,328 We observed a child who demonstrated marked resistance to nondepolarizing neuromuscular blocking drugs (NMBDs) 463 days after burn injury. This response indirectly suggests that the hyperkalemic response may persist long after the acute injury phase of the burn.329 The nondepolarizing NMBDs are therefore the relaxants of choice in children with a burn injury. It has been found that after burns over greater than 25% of body surface area, both the total dose of d-tubocurarine administered and the serum concentration necessary to attain a given degree of muscle twitch depression are three to five times greater than in children without burn injury.* Although d-tubocurarine is no longer used, similar observations have been made with the more modern nondepolarizing NMBDs (Fig. 34-9). If rapid intubation is needed and it is clear that the child’s lungs can be ventilated, high doses of rocuronium (1.2 mg/kg) can be used. However, even 1.2 mg/kg of rocuronium may not provide adequate conditions for rapid intubation and larger doses may be indicated (Fig. 34-10).330 Recovery from neuromuscular blockade has been observed at serum concentrations that would cause 100% twitch depression in children without burn injury. Studies with nondepolarizing NMBDs indicate that the hyposensitivity correlates well with the magnitude of burn (r = 0.88).180,331–333 Protein binding and pharmacokinetic studies with d-tubocurarine indicate that these two factors contribute little to the enhanced requirements.73,157,160,169 An increase in the number of acetylcholine receptors at junctional and extrajunctional areas and an altered affinity for the NMBD by those receptors have a major role in the elevated demand for nondepolarizing NMBDs.168,174,328 Even with high doses of rocuronium, the onset time is prolonged compared with that in nonburned children.334 Pharmacologic reversal of neuromuscular blockade, however, poses no special problem in burned children. Studies with intermediate- and short-acting nondepolarizing relaxants have shown resistance, but it is not as pronounced as has been observed with the long-acting relaxants.
Maintenance of anesthesia is usually accomplished with N2O, O2, an NMBD, and an opioid or inhalation agent. All of the commonly used anesthetic agents can be administered to burned children. Sevoflurane offers an advantage of smooth inhalation induction; isoflurane, desflurane, or halothane can be used for maintenance. No data show that repeated halothane anesthetics in burned children cause hepatotoxicity. All anesthetics cause concentration-dependent depression of cardiac output. In very ill children, anesthetic doses, but not NMBD requirements, are drastically reduced. In this injury, high-dose fentanyl-O2 anesthesia is well tolerated. Ketamine may be the anesthetic agent of choice in specific circumstances, including those in which we wish to avoid airway manipulation after application of fresh facial grafts, for very brief procedures, or children who are unable to open their mouth for a standard laryngoscopy. Ketamine sedation may also be used along with midazolam for sedation before a trial of extubation. E-Figure 34-4 illustrates extubation of a child with a severe facial burn with the use of an airway exchange catheter to provide a ready means for possible reintubation should the trial fail. Some burn centers use ketamine as the sole anesthetic and find it quite satisfactory.335 Ketamine can be used to potentiate the analgesic effects of opioids.336 The postoperative analgesia and somnolence for prolonged periods produced by high-dose ketamine may be considered an advantage in some instances in which postoperative agitation might dislodge fresh skin grafts; one report describes long-term ketamine for sedation and analgesia.337 Conversely, prolonged somnolence will delay reinstitution of critical enteral nutrition. Low-dose ketamine may be used postoperatively for its opioid-sparing effects.338 Ketamine either alone or with propofol is commonly used for burn dressing changes.339,340 An emerging experience with dexmedetomidine in children appears to demonstrate safety and efficacy in reducing otherwise common opioid tolerance and dose escalation.341
The inspired O2 concentration is regulated according to the arterial blood gases and O2 saturation. A pulse oximeter may not function properly on tissue discolored with silver nitrate; scraping the fingernail and cleaning the skin allow proper transmission and reception of the pulse oximeter light.342 Pulse oximetry has a vital role in identifying evolving hypoxemia before the desaturation becomes life-threatening.343 A pulse oximeter probe generally can function even on burned digits. If a child’s digits are swollen or vasoconstriction prevents normal pulse oximeter function, alternative sites must be sought, such as the earlobe, nasal septum, or tongue. We have found the tongue to be particularly valuable. An additional benefit of using the tongue is that the tissues of the mouth apparently direct the interfering electrons of the electrocautery unit away from the pulse oximeter probe placed on the tongue (i.e., minimal electrocautery artifact occurs even when multiple electrocautery devices are used simultaneously).243 A sealed oximeter probe that prevents electrical current leakage and leaching of adhesive can be easily modified (E-Fig. 34-5).344,345 Newer reflectance oximeters may also have a role in the care of burned children.346
The most important consideration of the intraoperative course is monitoring and correcting a child’s blood losses. For this reason, invasive intravascular monitoring is essential. Children may lose as much as 1 to 3 blood volumes during each burn excision. It is therefore necessary to be familiar with the surgical approach to burn excision. During a tangential excision (Fig. 34-11 and Video 34-1), a child might lose 3 to 5 times more blood than during excisions down to fascia (Video 34-2
). The liberal use of very dilute concentrations of epinephrine (500 µg/L in normal saline) injected subcutaneously in both donor and excision sites markedly reduces surgical blood loss (Fig. 34-12 and Video 34-3
)347; our institution uses a 1 : 2,000,000 epinephrine-containing solution (0.5 µg/mL). As much as, or even more than, 10 µg/kg epinephrine may be injected every 20 minutes because of the desensitization to catecholamines.173 It should be noted that significant fluid overload may occur several hours after surgery if excessive clysis or tumescent fluid is injected by the surgeons to facilitate harvesting of skin (Video 34-4
). We have observed a number of infants (~≤10 kg) who developed pulmonary edema several hours after their surgical procedure. Blood loss also depends on the expertise of the surgical team,348 and relatively “blood-free” excision and grafting has been described. It is difficult to estimate blood and fluid loss despite accurate weighing of surgical sponges because of significant losses hidden by the surgical drapes and evaporation. Other indicators of circulating blood volume, such as urine output, central venous pressure, arterial pressure, and shape of the arterial waveform (see Fig. 10-10), must be closely monitored.
Early excision of full-thickness burns has improved survival and shortened hospital stays.349–351 In the past, we routinely observed that 5% of the blood volume was lost for every 1% of the body surface excised and grafted.352,353 This extensive blood loss was a major source of morbidity and expense.354,355 During the last several years, effective blood-conserving techniques for excision have been developed that have drastically reduced intraoperative blood loss. These techniques include (1) clearly planning the excision to be performed before its initiation; (2) performing all extremity excisions under pneumatic tourniquet, exsanguinating the extremity before tourniquet inflation, and wrapping the extremity in a hemostatic dressing before tourniquet deflation; (3) conducting all fascial excisions with coagulating electrocautery; (4) performing major layered excisions as soon as possible after injury, before significant wound hyperemia develops; (5) executing all layered torso excisions after subeschar epinephrine clysis; (6) maintaining normothermia, primarily through maintaining a hot operating room (near 98.6° F [37° C]); and (7) subcutaneous injection of diluted epinephrine (with and without dilute bupivacaine) in donor areas.356
Large doses of epinephrine are well tolerated and markedly diminish bleeding. In a series of 25 consecutive children undergoing extensive layered excision, we used a total dose of epinephrine averaging 25 ± 3 µg/kg without complication.347 Based on preoperative and postoperative hematocrit and known volume of transfusion, the percent of the total blood volume lost per percent of total wound excised generated an average 0.98 ± 0.19% of the blood volume per percent of the body surface excised. This was about one fifth of our earlier experience with this type of excision.357,358 Operating with epinephrine clysis or tourniquets requires the surgeon to accurately determine wound bed viability in the absence of free bleeding. This is an important acquired skill that may be difficult to develop if the surgeon is not performing these procedures frequently.
Chronic ionized hypocalcemia is commonly observed with major thermal injury.137 Prophylactic intermittent administration of calcium chloride or calcium gluconate is strongly recommended during the rapid infusion of citrated blood products.359–362 We observed children experience electromechanical dissociation or cardiac arrest during the rapid administration of fresh frozen plasma (FFP). This observation prompted a controlled prospective study in which highly significant reductions in calcium were found when FFP was administered at a rate of 1 mL/kg/min or greater (see Fig. 10-9).362 Of interest, no relation was seen between adverse cardiovascular responses, rate of FFP infusion, or calcium concentration. A careful review of the previous cases of cardiac arrest revealed that all children were anesthetized with halothane, whereas in our prospective study most were anesthetized with “balanced” techniques. Because all inhalation agents depress cardiac function in part through their calcium channel–blocking activity, a sudden citrate-induced decrease in ionized calcium would be expected to cause additional cardiac dysfunction. Studies in our laboratory have, in fact, documented this interaction.21,362,363 Additional exogenous calcium is administered during rapid infusion of FFP or citrated whole blood, especially in infants (see Chapter 10).362 It is our clinical impression that the rapid administration of (cold) FFP or citrated whole blood through a central line, without additional exogenous calcium, may be more likely to induce severe hypotension, bradycardia, and electrical mechanical dissociation. Our experience has been that rapid administration of citrated blood products is safer through peripheral lines. Rapid administration of washed packed or citrated packed red blood cells does not cause ionized hypocalcemia. It would also seem advantageous to administer exogenous calcium through a peripheral line to avoid excessive concentrations in coronary vessels. However, calcium administered simultaneously in the same intravenous line with FFP or citrated blood products may cause clot formation unless the calcium is rapidly flushed through the intravenous system.
Special Considerations
Pharmacologic Responses
As a general rule, children with burn injuries require larger than normal doses of all medications, including antibiotics, NMBDs, opioids, and benzodiazepines.* Cardiovascular response to catecholamines may be attenuated because of a reduced affinity of β-adrenergic receptors for ligands and diminished second messenger production,173 thus the need for greater than standard doses to achieve the desired clinical response. Pharmacokinetic studies in acutely burned children indicate that the increased requirement for antibiotics is due in part to leakage through the burn wound, rapid urinary excretion, and altered volume of distribution.43 Thermal injuries of more than 30% of body surface area cause an upregulation of acetylcholine receptors and consequent resistance to NMBDs.167–169,171–174,364 In addition, there appears to be increased tolerance to sedatives and opioids. In adult burn patients, the free fraction (pharmacologically active component) of diazepam was greater than in nonburned patients, whereas the clearance of free diazepam was reduced. An increased tolerance to diazepam despite a greater fraction of the pharmacologically active compounds combined with a decreased clearance suggests resistance at tissue receptors similar to that observed for NMBDs at the neuromuscular junction.78 A similar tolerance has been observed with opioids. The persistence of such pharmacodynamic changes for both NMBDs and anesthetic drugs long after recovery from burn injury must be kept in mind and doses titrated according to patient responses.73,177,325,329
The pharmacology of many medications commonly used in burned children remains to be investigated.177 In general, it is necessary to be aware of both pharmacokinetic and pharmacodynamic changes. Furthermore, these children are frequently taking multiple medications, and therefore drug interactions, potentiations, and incompatibilities must be considered. Of particular importance in this context are the H2-receptor antagonists, which are commonly used in burned children and are known to inhibit the clearance of many other medications (see Chapter 6).
Methemoglobinemia
A less common, but important source of intraoperative cyanosis and hypoxemia is the development of methemoglobinemia. When silver nitrate dressings are used on the burn sites, there are some strains of gram-negative bacteria capable of reducing nitrates to nitrites, which diffuse into the bloodstream and convert hemoglobin into methemoglobin.120,121,285 The methemoglobin decreases the available O2-carrying capacity and increases the affinity of the unaltered hemoglobin for O2, thereby further impairing the delivery of O2. As a consequence, the O2-hemoglobin P50 curve is shifted to the left. Therefore methemoglobinemia should be considered in the differential diagnosis of cyanosis. Approximately 5 g of deoxyhemoglobin for each deciliter of blood is necessary to produce visible cyanosis, but a comparable skin color is produced by 1.5 to 2 g of methemoglobin for each deciliter of blood. Blood that contains more than approximately 10% methemoglobin usually appears dark red or even brown, despite a high measured Pao2, and does not change color even with vigorous agitation in room air. Measured O2 saturation or content is low. However, pulse oximetry, although demonstrating a decrease in saturation, provides a falsely increased value.235,236 Treatment consists of removing the toxic agent and administering methylene blue (2 mg/kg) and high inspired O2 concentrations.
Tracheal Tube Size
Because burned children frequently undergo multiple anesthetic procedures, special considerations must be given to the tracheal tube type and size. As mentioned previously, cuffed tracheal tubes are preferable. The size of the tracheal tube, the volume of air inflated into the cuff, and the pressure at which leakage occurs around the cuff should be recorded at each anesthetic. It is common to note that the requirement for a smaller diameter tracheal tube as weeks go by suggests the development of a subglottic lesion (stenosis, granuloma, polyps), which should be investigated with bronchoscopy. When N2O is used, the intraoperative cuff pressure should be checked to avoid excessive pressure on the tracheal mucosa, although MICROCUFF tubes provide a greater margin of safety than conventional tracheal tubes (see Chapter 12). We generally inflate the cuff to the minimum pressure that allows controlled ventilation and check the cuff pressure regularly.
Airway Control
The pediatric burn patient may present an especially difficult airway challenge to the anesthesiologist. This may be due to external airway factors such as temporomandibular joint limitation, macroglossia from thermal injury, and neck contractures.12,327 It also may be due to direct thermal or inhalational injuries to the glottis and respiratory tree. A detailed history and physical examination focusing on airway injury is vital. History details such as victim of fire in a closed space (e.g., house or automobile fire [very commonly associated with inhalational injuries]), vocal changes, stridor, and hoarseness may be important predictors of difficulty in establishing an airway.
Fiberoptic intubation is often aided in these children with manual distraction of the tongue (especially if macroglossia is present) and a jaw lift (Fig. 34-13). If the tongue is difficult to grasp, moderately high suction applied to the tip of the tongue or a gauze wrapped around the tongue and then gently pulling the tongue forward facilitates visualization of glottic structures (see Fig. 12-29).365 Fiberoptic intubation sometimes is more easily performed if the bronchoscope is guided through a laryngeal mask airway (LMA) that has already been seated and used to ventilate the lungs. This can be especially advantageous if there is a lot of perioral edema from inhalational burn injury.
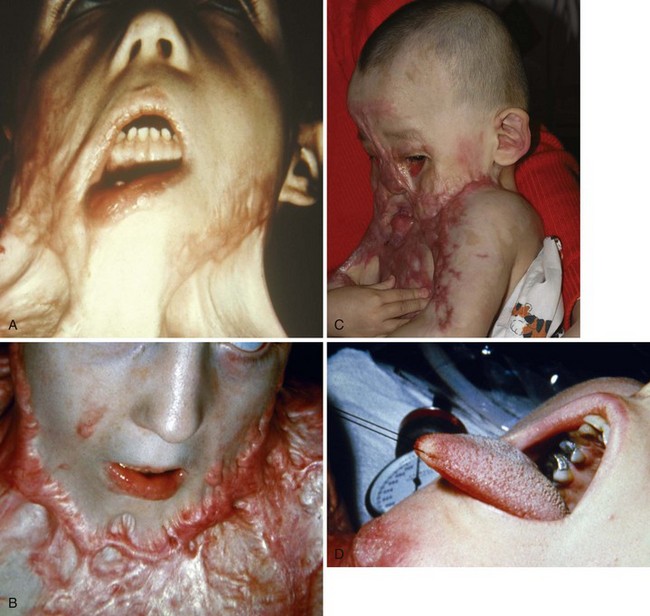
FIGURE 34-13 A, Child with inadequately treated facial burn. Note that skin contracture has resulted in complete distortion of the face with inability to close the right eye. B, Child with another example of an inadequately treated neck burn; note that her chin is fused with the sternum, resulting in very difficult airway management. C, An acute burn injury with an even more extreme example of inability to access the airway. To manage this child safely for the initial neck release, extracorporeal membrane oxygenation was used. This child is also unable to close her eyes. D, Some children with severe neck burns may have their airway visualized only by pulling back on the tongue (zero silk suture, suction applied to the tip of the tongue, or grasping forceps may be used [see also Fig. 12-29]) so as to pull the tongue and larynx cephalad.
In addition to direct laryngoscopy, fiberoptic intubations, and LMA-assisted intubations, other techniques have been described, including retrograde wires and light-wand intubations. These techniques may be difficult to use in a child with a severely burned neck and contractures. In children with severe neck or oral contractures, the surgeon may release the contracture during ketamine sedation and spontaneous ventilation to facilitate access to the airway (Video 34-5). The airway may then be instrumented either directly or indirectly (see Chapter 12).
Hyperalimentation
Hyperalimentation fluids are frequently administered to burned children.99,366 These fluids should be continued intraoperatively; however, we generally reduce the rate of infusion to half to two thirds of the initial infusion rate because metabolic rate is usually decreased during anesthesia. These fluids should be administered with a constant-infusion pump to avoid accidental overinfusion or underinfusion. If the hyperalimentation fluids must be terminated (e.g., to permit blood transfusion), monitoring of blood glucose levels is recommended. Dangerous rebound hypoglycemia may occur if infusion of these solutions is abruptly interrupted and no compensation is made with other glucose-containing solutions. It should be noted that most blood products, particularly whole blood and FFP, provide a significant glucose load. Compatibility of hyperalimentation solutions with drugs, blood, and other infusions must be addressed.
Ultrasound-Guided Vascular Access, Regional Analgesia, and Cardiovascular Assessment
Vascular access for the pediatric burn patient can be extremely challenging. However, placing central venous and arterial catheters in the operating room under carefully controlled conditions is associated with a rate of acute mechanical complications and deep vein thrombosis of less than 1%.367 This applies to both central and peripheral access as a result of thermal damage from the burn, surgical grafting over peripheral vessels, and increased clotting and thrombi from multiple factors such as multiple prior cannulations, hypercoagulable state from the burn, and long periods of being bedbound. Ultrasound is useful not only to more rapidly and safely access arteries and veins but also to diagnose clotted vessels, saving the child many futile attempts at obtaining vascular access.368 Ultrasound also helps establish the location of cannulae. For peripherally inserted central catheters (PICCs), we first use ultrasound to assist in cannulating a vein, then place the probe over the internal jugular to verify that the PICC is not traveling cephalad, and scan the subclavian vein to verify placement.
Ultrasound-guided regional anesthesia is also a valuable tool in the care of the burned child undergoing reconstructive surgery. Typically, the complaints children have after reconstructive procedures involve the pain of the graft donor site. For the past 5 years we have improved the postoperative experience by placing ultrasound-guided blocks of donor sites, sometimes with catheters for further postoperative analgesia. Specific blocks we have found very useful include the lateral femoral cutaneous nerve and fascia iliaca to cover the most common donor site—the lateral thigh. Using ultrasound, reliability of these blocks is significantly improved over typical blind techniques.369,370 We find that block of the lateral femoral cutaneous nerve provides better pain control for the donor site than infiltrating the donor site with local anesthetics. Longer analgesia can be provided by leaving a catheter in place. Usually we place fascia iliaca catheters; lateral femoral cutaneous nerve catheters also work, but we have found the learning curve for successful placement to be a bit steeper.371
Ultrasound is one of several strategies to rapidly assess intraoperative cardiac function during burn surgery. We have used real-time ultrasound while decompressing a spontaneous pneumothorax in a burn patient as a rapid and reliable indicator of when the lung has reexpanded as indicated by return of the pleural “sliding” sign.372–374 Hemodynamic measurements also can be estimated intraoperatively; we use the Bedside Assessment for Trauma/Critical Care (BEAT) examination as a quick assessment of volume status.375,376
Awakening
In the immediate postoperative period, O2 consumption increases even in the absence of shivering.37 If O2 debt develops (metabolic acidosis), appropriate measures must be taken to correct it. Special consideration also must be given to the likelihood of severe pain. Analgesic drugs should be administered in increasingly liberal doses because of increased drug tolerance. Adequacy of air exchange and patency of the airway, however, must be given first priority. It is important to assess the leak pressure at the end of the surgical procedure. The airway patency of the burned child is highly dynamic, and the child whose airway had minimal edema at the beginning of a procedure may have a very edematous airway at the end and be a poor candidate for extubation.
Pain Management and Postoperative Care
Part of the challenge in managing burn pain is due to the overlay of physiologic and psychosocial responses to thermal injury. Besides physical stimulation of nociceptors and other direct pain mechanisms, there is also the very real anticipation, anxiety, and fear associated with these procedures. Evidence suggests that skillful communication and explanations of why certain treatments are necessary, despite the pain caused, decreases analgesic requirements.320,377–379
Opioid administration for pain control has been an evolving science for the child with a burn. Twenty years ago there was significant fear that treatment of pain with opioids would create addictions. However, no reports of children developing opioid addiction after therapeutic uses of opioids have been published and studies in adults revealed a very low addiction rate.380–384 This has led to liberalization of opioid dosing. It is not unusual for children to receive more than 1 mg/kg/hr of morphine intravenously while recovering from burn injuries in our intensive care unit. Once the thermal wounds are closed, opioid requirements rapidly decrease (Fig. 34-14).
Although the fear of post–burn care addiction to opioids has not been realized, there are other reasons why this class of drugs may be detrimental to the child with a burn. Recent evidence from rats suggests that thermal injury itself may lead to a hyperalgesic state with both reduced efficacy of morphine (presumably from downregulation of spinal µ receptors) and increases in N-methyl-d-aspartate (NMDA) receptors. The increases in NMDA receptors induced by burns provide the rationale for the widespread use of ketamine to treat pain in these children. Opioids may also increase sensitivity to pain. Morphine has been shown in a mouse model to downregulate µ-opioid receptors within the spinal cord and cause injury to spinal inhibitory interneurons.383,384 Opioids in mice have also been shown to cause postburn immunosuppression in the mouse model.385
The fact that opioids may have disadvantages when used for pain management has led to a search for alternative analgesics. Among these are potentially dexmedetomidine,386–392 gabapentin,393 and, until their recent withdrawal from the market, cyclooxygenase-2 inhibitors.394–396
Dexmedetomidine is a parenterally administered α2-adrenoceptor agonist with good sedative and anxiolytic properties. In adults, it has been demonstrated to decrease opioid requirements postoperatively.387–391397 In children with burns, it has been used successfully for sedation,386 although larger dexmedetomidine doses may be needed than those required in nonburned adults or nonburned children.386 Dexmedetomidine does not appear to be a remarkable analgesic for children with burns. In a prospective study of dexmedetomidine in acutely burned children,398 a bolus dose of intravenous dexmedetomidine (1 µg/kg over 10 minutes) was followed by an ascending infusion protocol (0.7 µg/kg/hr to 2.2 µg/kg/hr).399 We found no instances of heart block or other arrhythmias, but noted a consistent and significant decrease in mean arterial blood pressure (MAP) after the bolus (approaching 30% change in mean pressure) (Fig. 34-15). This occurred in all ages studied (2 to 18 years), although the decrease in the MAP did not correlate with size of burn, time out from burn, or central venous pressure, before the study. Given these observations, we avoid a bolus dose of dexmedetomidine. However, should a bolus dose be indicated, we recommend 10 mL/kg or more of balanced salt solution or mild pressor support before commencing dexmedetomidine. Other investigators have retrospectively examined cardiovascular stability of pediatric burn patients sedated with dexmedetomidine and concluded that in the absence of a loading dose, blood pressure was maintained in the dexmedetomidine treatment group.400 Dexmedetomidine pharmacokinetics in nonburned children appear similar to those in adults, but they have not been reported in pediatric burn patients.401–403 When dexmedetomidine was compared with midazolam as an adjunct to ketamine in sedation and analgesia for burn dressing changes, the authors determined that both were effective adjuncts but that the dexmedetomidine ketamine group had better sedation with less hemodynamic instability.404 Dexmedetomidine has also been used in anxious children with burns as an intranasal premedication.405 The authors concluded that dexmedetomidine (2 µg/kg) intranasally as a premedication compared with oral midazolam (0.5 mg/kg), induced preoperative sleep faster and was equally effective for induction conditions and rapidity of emergence. In our experience, the majority of our children prefer oral midazolam despite its bitter aftertaste, to intranasal dexmedetomidine. A substantial detractor from dexmedetomidine use is its cost (more than $44 for a 100-µg vial at our institutions). One study in adult volunteers compared intravenous with intranasal dexmedetomidine; pharmacodynamic responses were similar, but the peak blood concentration occurred sooner in the intravenous group.406 Bioavailability after nasal administration was 65% (range 35% to 93%).
Dressing changes sometimes present one of the greatest analgesic challenges. This is because they are very painful, cause a rapid rise above baseline pain, and are associated by the child with anticipation of impending pain. Various methods to manage this pain have been used, including additional opioids and benzodiazepines, ketamine,335 intranasal fentanyl,407 immersive virtual reality,408,409 and music therapy.410 Management of the child’s pain depends on physiologic and pharmacologic factors, as well as the psychological state of the child. Unfortunately, some children become so tolerant that one author observed that doses of intravenous fentanyl as large as 100 µg/kg administered as a bolus did not cause respiratory depression nor did it control the pain (CJC).
When poorly controlled, pain and anxiety have adverse psychologic146,411,412 and physiologic effects.19 Posttraumatic stress disorder has been reported to occur in up to 30% of those with serious burns413,414 and may be related to both the accident and the treatment, particularly in the setting of inadequate control of pain and anxiety. An inconsistent approach to pain and anxiety will be associated with inappropriate degrees of child discomfort, nonuniform drug selection with inconsistent dosing of unfamiliar drugs, varying tolerance of child discomfort among staff members, and bedside disagreements over management of the child’s distress.
To address this issue, a pain and anxiety guideline should be developed by all facilities routinely treating burned children.415–418 We developed one such guideline that we have followed for several years, which is briefly described in Table 34-3.321 The ideal characteristics of such a guideline include (1) safety and efficacy over the broad range of ages and injury acuities seen in the particular unit; (2) explicit recommendations for drug selection, dosing, and escalation of dosing; (3) a limited formulary that generates staff familiarity with agents used; and (4) regular assessment of pain and anxiety levels and guidance for intervention as needed through dose ranging. We have found this structured approach to manage this predictable problem to be very effective over the broad range of injury severity and child ages seen in our unit. Substantial escalation of drug doses, particularly in children with large injuries, is commonly required; doses should be titrated to the child’s needs. When the child is being weaned toward extubation, background medications should be tapered toward a sensorium consistent with airway protection; many children’s tracheas are safely extubated while they are still receiving opioid and benzodiazepine infusions. Finally, it is essential to emphasize that the most effective of all analgesics and anxiolytics is prompt, definitive wound closure.
Tolerance to opioids occurs over time and must be considered so that adequate analgesia is provided throughout the recovery period. It is common to observe children who receive 1 mg/kg of morphine at the beginning of a 2-hour operative procedure not only to be ready for extubation but also to require additional opioids for continued pain relief postoperatively. Similar trends have been observed for fentanyl.162 Thus an increased rate of excretion and degradation may influence the effect of some opioids. As the child recovers, the painful stimuli diminish and the opioid requirements are gradually reduced. This is generally such a prolonged process that withdrawal is not an issue. Anesthesiologists can have a central role in the treatment of thermal injury pain and, with an understanding of pharmacology, pharmacokinetics, and pharmacodynamics, are a vital resource for the care of these children (see Chapters 43 and 44).
Han T, Kim H, Bae J, et al. Neuromuscular pharmacodynamics of rocuronium in patients with major burns. Anesth Analg. 2004;99:386–392.
Scheinkestel CD, Bailey M, Myles PS, et al. Hyperbaric or normobaric oxygen for acute carbon monoxide poisoning: a randomised controlled clinical trial. Med J Aust. 1999;170:203–210.
Sheridan RL, Szyfelbein SK. Staged high-dose epinephrine clysis is safe and effective in extensive tangential burn excisions in children. Burns. 1999;25:745–748.
Weaver LK, Hopkins RO, Chan KJ, et al. Hyperbaric oxygen for acute carbon monoxide poisoning. N Engl J Med. 2002;347:1057–1067.
1 Brigham PA, McLoughlin E. Burn incidence and medical care use in the United States: estimates, trends, and data sources. J Burn Care Rehabil. 1996;17:95–107.
2 MacLennan N, Heimbach DM, Cullen BF. Anesthesia for major thermal injury. Anesthesiology. 1998;89:749–770.
3 Latenser BA, Miller SF, Bessey PQ, et al. National Burn Repository 2006: a ten-year review. J Burn Care Res. 2007;28:635–658.
4 D’Souza AL, Nelson NG, McKenzie LB. Pediatric burn injuries treated in US emergency departments between 1990 and 2006. Pediatrics. 2009;124:1424–1430.
5 Monafo WW. Initial management of burns. N Engl J Med. 1996;335:1581–1586.
6 Alvarado R, Chung KK, Cancio LC, Wolf SE. Burn resuscitation. Burns. 2009;35:4–14.
7 Dimick AR, Brigham PA, Sheehy EM. The development of burn centers in North America. J Burn Care Rehabil. 1993;14:284–299.
8 Sharma RK, Parashar A. Special considerations in paediatric burn patients. Indian J Plast Surg. 2010;43:S43–S50.
9 Taira BR, Cassara G, Meng H, et al. Predictors of sustaining burn injury: does the use of common prevention strategies matter? J Burn Care Res. 2011;32:20–25.
10 Istre GR, McCoy M, Carlin DK, McClain J. Residential fire related deaths and injuries among children: fireplay, smoke alarms, and prevention. Inj Prev. 2002;8:128–132.
11 Duke J, Wood F, Semmens J, et al. A study of burn hospitalizations for children younger than 5 years of age: 1983-2008. Pediatrics. 2011;127:e971–e977.
12 Fuzaylov G, Fidkowski CW. Anesthetic considerations for major burn injury in pediatric patients. Paediatr Anaesth. 2009;19:202–211.
13 Arturson G. Pathophysiology of the burn wound and pharmacological treatment. The Rudi Hermans Lecture, 1995. Burns. 1996;22:255–274.
14 Jeschke MG, Gauglitz GG, Kulp GA, et al. Long-term persistance of the pathophysiologic response to severe burn injury. PLoS One. 2011;6:e21245.
15 Myrianthefs PM, Baltopoulos GJ. Circulating cytokines and outcome prediction of burned children with concomitant inhalation injury. Crit Care. 2008;12:155.
16 Gauglitz GG, Finnerty CC, Herndon DN, Mlcak RP, Jeschke MG. Are serum cytokines early predictors for the outcome of burn patients with inhalation injuries who do not survive? Crit Care. 2008;12:R81.
17 Gauglitz GG, Song J, Herndon DN, et al. Characterization of the inflammatory response during acute and post-acute phases after severe burn. Shock. 2008;30:503–507.
18 Jeschke MG, Barrow RE, Herndon DN. Extended hypermetabolic response of the liver in severely burned pediatric patients. Arch Surg. 2004;139:641–647.
19 Youn YK, Lalonde C, Demling R. The role of mediators in the response to thermal injury. World J Surg. 1992;16:30–36.
20 Morgan RJ, Martyn JA, Philbin DM, Coggins CH, Burke JF. Water metabolism and antidiuretic hormone (ADH) response following thermal injury. J Trauma. 1980;20:468–472.
21 Martyn JA, Burke JF. Is there a selective increase in pulmonary capillary permeability following cutaneous burns? Chest. 1979;76:374–375.
22 Martyn JA, Snider MT, Farago LF, Burke JF. Thermodilution right ventricular volume: a novel and better predictor of volume replacement in acute thermal injury. J Trauma. 1981;21:619–626.
23 Martyn JA, Snider MT, Szyfelbein SK, Burke JF, Laver MB. Right ventricular dysfunction in acute thermal injury. Ann Surg. 1980;191:330–335.
24 Turbow ME. Abdominal compression following circumferential burn: cardiovascular responses. J Trauma. 1973;13:535–541.
25 Sambol JT, White J, Horton JW, Deitch EA. Burn-induced impairment of cardiac contractile function is due to gut-derived factors transported in mesenteric lymph. Shock. 2002;18:272–276.
26 Barret JP, Herndon DN. Modulation of inflammatory and catabolic responses in severely burned children by early burn wound excision in the first 24 hours. Arch Surg. 2003;138:127–132.
27 Andrzejewska E, Niewiadomska H. Evaluation of selected parameters of the cytokine system in burn wounds in children. Pediatr Surg Int. 2000;16:80–84.
28 Horton JW, White DJ. Cardiac responses to burn injury in young and adult guinea pigs. Shock. 1999;12:280–287.
29 Etherington L, Saffle J, Cochran A. Use of transesophageal echocardiography in burns: a retrospective review. J Burn Care Res. 2010;31:36–39.
30 Williams FN, Herndon DN, Suman OE, et al. Changes in cardiac physiology after severe burn injury. J Burn Care Res. 2011;32:269–274.
31 Mak GZ, Hardy AR, Meyer RA, Kagan RJ. Reversible cardiomyopathy after severe burn injury. J Burn Care Res. 2006;27:482–486.
32 Sakurai H, Nozaki M, Traber KS, Traber DL. Atrial natriuretic peptide release associated with smoke inhalation and physiological responses to thermal injury in sheep. Burns. 2005;31:737–743.
33 Smith A, Barclay C, Quaba A, et al. The bigger the burn, the greater the stress. Burns. 1997;23:291–294.
34 Onuoha GN, Alpar EK, Gowar J. Plasma levels of atrial natriuretic peptide in severe burn injury. Burns. 2000;26:449–453.
35 Murton SA, Tan ST, Prickett TC, Frampton C, Donald RA. Hormone responses to stress in patients with major burns. Br J Plast Surg. 1998;51:388–392.
36 Kulp GA, Herndon DN, Lee JO, Suman OE, Jeschke MG. Extent and magnitude of catecholamine surge in pediatric burned patients. Shock. 2010;33:369–374.
37 Demling RH, Lalonde C. Oxygen consumption is increased in the postanesthesia period after burn excision. J Burn Care Rehabil. 1989;10:381–387.
38 Lalonde C, Demling RH. The effect of complete burn wound excision and closure on postburn oxygen consumption. Surgery. 1987;102:862–868.
39 Williams FN, Herndon DN, Kulp GA, Jeschke MG. Propranolol decreases cardiac work in a dose-dependent manner in severely burned children. Surgery. 2011;149:231–239.
40 Norbury WB, Jeschke MG, Herndon DN. Metabolism modulators in sepsis: propranolol. Crit Care Med. 2007;35:S616–S620.
41 Tredget EE, Shankowsky HA, Taerum TV, Moysa GL, Alton JD. The role of inhalation injury in burn trauma: a Canadian experience. Ann Surg. 1990;212:720–727.
42 Fein A, Leff A, Hopewell PC. Pathophysiology and management of the complications resulting from fire and the inhaled products of combustion: review of the literature. Crit Care Med. 1980;8:94–98.
43 Barillo DJ, Goode R, Esch V. Cyanide poisoning in victims of fire: analysis of 364 cases and review of the literature. J Burn Care Rehabil. 1994;15:46–57.
44 McCall JE, Cahill TJ. Respiratory care of the burn patient. J Burn Care Rehabil. 2005;26:200–206.
45 Hassan Z, Wong JK, Bush J, Bayat A, Dunn KW. Assessing the severity of inhalation injuries in adults. Burns. 2010;36:212–216.
46 Desai MH, Mlcak R, Richardson J, Nichols R, Herndon DN. Reduction in mortality in pediatric patients with inhalation injury with aerosolized heparin/N-acetylcystine [correction of acetylcystine] therapy. J Burn Care Rehabil. 1998;19:210–212.
47 Oremus M, Hanson MD, Whitlock R, et al. A systematic review of heparin to treat burn injury. J Burn Care Res. 2007;28:794–804.
48 Holt J, Saffle JR, Morris SE, Cochran A. Use of inhaled heparin/N-acetylcystine in inhalation injury: does it help? J Burn Care Res. 2008;29:192–195.
49 Hantson P, Butera R, Clemessy JL, Michel A, Baud FJ. Early complications and value of initial clinical and paraclinical observations in victims of smoke inhalation without burns. Chest. 1997;111:671–675.
50 Martyn JA, Aikawa N, Wilson RS, Szyfelbein SK, Burke JF. Extrapulmonary factors influencing the ratio of arterial oxygen tension to inspired oxygen concentration in burn patients. Crit Care Med. 1979;7:492–496.
51 Palmieri TL, Warner P, Mlcak RP, et al. Inhalation injury in children: a 10 year experience at Shriners Hospitals for Children. J Burn Care Res. 2009;30:206–208.
52 Gupta KL, Kumar R, Sekhar MS, Sakhuja V, Chugh KS. Myoglobinuric acute renal failure following electrical injury. Ren Fail. 1991;13:23–25.
53 Tomkins KL, Holland AJ. Electrical burn injuries in children. J Paediatr Child Health. 2008;44:727–730.
54 Celik A, Ergun O, Ozok G. Pediatric electrical injuries: a review of 38 consecutive patients. J Pediatr Surg. 2004;39:1233–1237.
55 Aikawa N, Wakabayashi G, Ueda M, Shinozawa Y. Regulation of renal function in thermal injury. J Trauma. 1990;30:S174–S178.
56 Popp MB, Silberstein EB, Srivastava LS, et al. A pathophysiologic study of the hypertension associated with burn injury in children. Ann Surg. 1981;193:817–824.
57 Huribal M, Cunningham ME, D’Aiuto ML, Pleban WE, McMillen MA. Endothelin-1 and prostaglandin E2 levels increase in patients with burns. J Am Coll Surg. 1995;180:318–322.
58 Huribal M, Cunningham ME, D’Aiuto ML, Pleban WE, McMillen MA. Endothelin levels in patients with burns covering more than 20% body surface area. J Burn Care Rehabil. 1995;16:23–26.
59 Loirat P, Rohan J, Baillet A, et al. Increased glomerular filtration rate in patients with major burns and its effect on the pharmacokinetics of tobramycin. N Engl J Med. 1978;299:915–919.
60 Martyn JA, Greenblatt DJ, Hagen J, Hoaglin DC. Alteration by burn injury of the pharmacokinetics and pharmacodynamics of cimetidine in children. Eur J Clin Pharmacol. 1989;36:361–367.
61 Martyn JA, Greenblatt DJ, Abernethy DR. Increased cimetidine clearance in burn patients. JAMA. 1985;253:1288–1291.
62 Luterman A, Dacso CC, Curreri PW. Infections in burn patients. Am J Med. 1986;81:45–52.
63 Garrelts JC, Peterie JD. Altered vancomycin dose vs. serum concentration relationship in burn patients. Clin Pharmacol Ther. 1995;44:9–13.
64 Dailly E, Le FR, Deslandes G, Pannier M, Jolliet P. Influence of glomerular filtration rate on the clearance of vancomycin administered by continuous infusion in burn patients. Int J Antimicrob Agents. 2008;31:537–539.
65 Schiavon M, Di Landro D, Baldo M, De Silvestro G, Chiarelli A. A study of renal damage in seriously burned patients. Burns Incl Therm Inj. 1988;14:107–112.
66 Akrami C, Falkner B, Gould AB, DeClement FA, Bendlin A. Plasma renin and occurrence of hypertension in children with burn injuries. J Trauma. 1980;20:130–134.
67 Falkner B, Roven S, DeClement FA, Bendlin A. Hypertension in children with burns. J Trauma. 1978;18:213–217.
68 Wilmore DW, Goodwin CW, Aulick LH, et al. Effect of injury and infection on visceral metabolism and circulation. Ann Surg. 1980;192:491–504.
69 Tadros T, Traber DL, Herndon DN. Hepatic blood flow and oxygen consumption after burn and sepsis. J Trauma. 2000;49:101–108.
70 Jeschke MG, Barrow RE, Herndon DN. Extended hypermetabolic response of the liver in severely burned pediatric patients. Arch Surg. 2004;139:641–647.
71 Herndon DN, Stein MD, Rutan TC, Abston S, Linares H. Failure of TPN supplementation to improve liver function, immunity, and mortality in thermally injured patients. J Trauma. 1987;27:195–204.
72 Barret JP, Jeschke MG, Herndon DN. Fatty infiltration of the liver in severely burned pediatric patients: autopsy findings and clinical implications. J Trauma. 2001;51:736–739.
73 Martyn J. Clinical pharmacology and drug therapy in the burned patient. Anesthesiology. 1986;65:67–75.
74 Kart T, Christrup LL, Rasmussen M. Recommended use of morphine in neonates, infants and children based on a literature review. II. Clinical use. Pediatr Anaesth. 1997;7:93–101.
75 Herman RA, Veng-Pedersen P, Miotto J, Komorowski J, Kealey GP. Pharmacokinetics of morphine sulfate in patients with burns. J Burn Care Rehabil. 1994;15:95–103.
76 Furman WR, Munster AM, Cone EJ. Morphine pharmacokinetics during anesthesia and surgery in patients with burns. J Burn Care Rehabil. 1990;11:391–394.
77 Cederholm I, Bengtsson M, Bjorkman S, Choonara I, Rane A. Long term high dose morphine, ketamine and midazolam infusion in a child with burns. Br J Clin Pharmacol. 1990;30:901–905.
78 Martyn JA, Greenblatt DJ, Quinby WC. Diazepam kinetics in patients with severe burns. Anesth Analg. 1983;62:293–297.
79 Martyn J, Greenblatt DJ. Lorazepam conjugation is unimpaired in burn trauma. Clin Pharmacol Ther. 1988;43:250–255.
80 Mohnot D, Snead OC, III., Benton JW, Jr. Burn encephalopathy in children. Ann Neurol. 1982;12:42–47.
81 Antoon AY, Volpe JJ, Crawford JD. Burn encephalopathy in children. Pediatrics. 1972;50:609–616.
82 Sheridan RL, McEttrick M, Bacha G, Stoddard F, Tompkins RG. Midazolam infusion in pediatric patients with burns who are undergoing mechanical ventilation. J Burn Care Rehabil. 1994;15:515–518.
83 Chance WT, Berlatzky Y, Minnema K, et al. Burn trauma induces anorexia and aberrations in CNS amine neurotransmitters. J Trauma. 1985;25:501–507.
84 McKee AC, Winkelman MD, Banker BQ. Central pontine myelinolysis in severely burned patients: relationship to serum hyperosmolality. Neurology. 1988;38:1211–1217.
85 Demling RH. Burns. N Engl J Med. 1985;313:1389–1398.
86 Wallner SF, Vautrin R. The anemia of thermal injury: mechanism of inhibition of erythropoiesis. Proc Soc Exp Biol Med. 1986;181:144–150.
87 Vasko SD, Burdge JJ, Ruberg RL, Verghese AS. Evaluation of erythropoietin levels in the anemia of thermal injury. J Burn Care Rehabil. 1991;12:437–441.
88 Housinger TA, Brinkerhoff C, Warden GD. The relationship between platelet count, sepsis, and survival in pediatric burn patients. Arch Surg. 1993;128:65–66.
89 Lawrence C, Atac B. Hematologic changes in massive burn injury. Crit Care Med. 1992;20:1284–1288.
90 Still JMJ, Belcher K, Law EJ, et al. A double-blinded prospective evaluation of recombinant human erythropoietin in acutely burned patients. J Trauma. 1995;38:233–236.
91 Poletes GP, Miller SF, Finley RK, Lincks J. Blood use in the burn unit: a possible role for erythropoietin. J Burn Care Rehabil. 1994;15:37–41.
92 Lundy JB, Hetz K, Chung KK, et al. Outcomes with the use of recombinant human erythropoietin in critically ill burn patients. Am Surg. 2010;76:951–956.
93 Warner P, Fields AL, Braun LC, et al. Thrombocytopenia in the pediatric burn patient. J Burn Care Res. 2011;32:410–414.
94 Simon TL, Curreri PW, Harker LA. Kinetic characterization of hemostasis in thermal injury. J Lab Clin Med. 1977;89:702–711.
95 Cullen JJ, Murray DJ, Kealey GP. Changes in coagulation factors in patients with burns during acute blood loss. J Burn Care Rehabil. 1989;10:517–522.
96 de Macedo JL, Rosa SC, Castro C. Sepsis in burned patients. Rev Soc Bras Med Trop. 2003;36:647–652.
97 Levin GY, Egorihina MN. The role of fibrinogen in aggregation of platelets in burn injury. Burns. 2010;36:806–810.
98 Munster AM. The early management of thermal burns. Surgery. 1980;87:29–40.
99 Sheridan RL, Yu YM, Prelack K, et al. Maximal parenteral glucose oxidation in hypermetabolic young children: a stable isotope study. JPEN J Parenter Enteral Nutr. 1998;22:212–216.
100 Vijfhuize S, Verburg M, Marino L, van DM, Rode H. An evaluation of nutritional practice in a paediatric burns unit. S Afr Med J. 2010;100:383–386.
101 Deitch EA, Rutan R, Waymack JP. Trauma, shock, and gut translocation. New Horiz. 1996;4:289–299.
102 Khorasani EN, Mansouri F. Effect of early enteral nutrition on morbidity and mortality in children with burns. Burns. 2010;36:1067–1071.
103 Andel D, Kamolz LP, Donner A, et al. Impact of intraoperative duodenal feeding on the oxygen balance of the splanchnic region in severely burned patients. Burns. 2005;31:302–305.
104 Herndon DN. Nutritional and pharmacological support of the metabolic response to injury. Minerva Anestesiol. 2003;69:264–274.
105 Burke JF, Wolfe RR, Mullany CJ, Mathews DE, Bier DM. Glucose requirements following burn injury: parameters of optimal glucose infusion and possible hepatic and respiratory abnormalities following excessive glucose intake. Ann Surg. 1979;190:274–285.
106 Williams FN, Branski LK, Jeschke MG, Herndon DN. What, how, and how much should patients with burns be fed? Surg Clin North Am. 2011;91:609–629.
107 Sefton EJ, Boulton-Jones JR, Anderton D, Teahon K, Knights DT. Enteral feeding in patients with major burn injury: the use of nasojejunal feeding after the failure of nasogastric feeding. Burns. 2002;28:386–390.
108 Czaja AJ, McAlhany JC, Andes WA, Pruitt BA, Jr. Acute gastric disease after cutaneous thermal injury. Arch Surg. 1975;110:600–605.
109 Matsui M, Kudo T, Kudo M, Ishihara H, Matsuki A. The endocrine response after burns. Agressologie. 1991;32:233–235.
110 Dolecek R, Adamkova M, Sotornikova T, Zavada M, Kracmar P. Endocrine response after burn. Scand J Plast Reconstr Surg. 1979;13:9–16.
111 Jeschke MG, Finnerty CC, Suman OE, et al. The effect of oxandrolone on the endocrinologic, inflammatory, and hypermetabolic responses during the acute phase postburn. Ann Surg. 2007;246:351–360.
112 Miller JT, Btaiche IF. Oxandrolone treatment in adults with severe thermal injury. Pharmacotherapy. 2009;29:213–226.
113 Sugita H, Kaneki M, Sugita M, et al. Burn injury impairs insulin-stimulated Akt/PKB activation in skeletal muscle. Am J Physiol Endocrinol Metab. 2005;288:E585–E591.
114 Fram RY, Cree MG, Wolfe RR, et al. Intensive insulin therapy improves insulin sensitivity and mitochondrial function in severely burned children. Crit Care Med. 2010;38:1475–1483.
115 Pham TN, Warren AJ, Phan HH, et al. Impact of tight glycemic control in severely burned children. J Trauma. 2005;59:1148–1154.
116 Jeschke MG, Kraft R, Emdad F, et al. Glucose control in severely thermally injured pediatric patients: what glucose range should be the target? Williams FN, Herndon DN. Ann Surg. 2010;252:521–527.
117 Kasper SO, Castle SM, Daley BJ, Enderson BL, Karlstad MD. Blockade of the renin-angiotensin system improves insulin sensitivity in thermal injury. Shock. 2006;26:485–488.
118 Gardner GG, Martin CJ. The mathematical modelling of thermal responses of normal subjects and burned patients. Physiol Meas. 1994;15:381–400.
119 Quinby WC, Jr. Restrictive effects of thoracic burns in children. J Trauma. 1972;12:646–655.
120 Monafo WW, Freedman B. Topical therapy for burns. Surg Clin North Am. 1987;67:133–145.
121 Monafo WW, West MA. Current treatment recommendations for topical burn therapy. Drugs. 1990;40:364–373.
122 Murphy KD, Lee JO, Herndon DN. Current pharmacotherapy for the treatment of severe burns. Expert Opin Pharmacother. 2003;4:369–384.
123 Atiyeh BS, Costagliola M, Hayek SN, Dibo SA. Effect of silver on burn wound infection control and healing: review of the literature. Burns. 2007;33:139–148.
124 Leaper DJ. Silver dressings: their role in wound management. Int Wound J. 2006;3:282–294.
125 Greenhalgh DG. Topical antimicrobial agents for burn wounds. Clin Plast Surg. 2009;36:597–606.
126 Cone JB, Wallace BH, Caldwell FT, Jr. The effect of staged burn wound closure on the rates of heat production and heat loss of burned children and young adults. J Trauma. 1988;28:968–972.
127 Jahoor F, Desai M, Herndon DN, Wolfe RR. Dynamics of the protein metabolic response to burn injury. Metabolism. 1988;37:330–337.
128 Wolfe RR, Herndon DN, Jahoor F, Miyoshi H, Wolfe M. Effect of severe burn injury on substrate cycling by glucose and fatty acids. N Engl J Med. 1987;317:403–408.
129 Aulick LH, McManus AT, Mason ADJ, et al. Effects of infection on oxygen consumption and core temperature in experimental thermal injury. Ann Surg. 1986;204:48–52.
130 Baxter CR. Problems and complications of burn shock resuscitation. Surg Clin North Am. 1978;58:1313–1322.
131 Kien CL, Rohrbaugh DK, Burke JF, Young VR. Whole body protein synthesis in relation to basal energy expenditure in healthy children and in children recovering from burn injury. Pediatr Res. 1978;12:211–216.
132 Aulick LH, Wilmore DW. Increased peripheral amino acid release following burn injury. Surgery. 1979;85:560–565.
133 Deitch EA. The management of burns. N Engl J Med. 1990;323:1249–1253.
134 Jeschke MG, Mlcak RP, Finnerty CC, et al. Burn size determines the inflammatory and hypermetabolic response. Crit Care. 2007;11:R90.
135 Childs C, Little RA. Acute changes in oxygen consumption and body temperature after burn injury. Arch Dis Child. 1994;71:31–34.
136 Gore DC, Chinkes D, Sanford A, et al. Influence of fever on the hypermetabolic response in burn-injured children. Arch Surg. 2003;138:169–174.
137 Szyfelbein SK, Drop LJ, Martyn JAJ, Martyn JA. Persistent ionized hypocalcemia in patients during resuscitation and recovery phases of body burns. Crit Care Med. 1981;9:454–458.
138 Klein GL, Langman CB, Herndon DN. Persistent hypoparathyroidism following magnesium repletion in burn-injured children. Pediatr Nephrol. 2000;14:301–304.
139 Klein GL, Wimalawansa SJ, Kulkarni G, et al. The efficacy of acute administration of pamidronate on the conservation of bone mass following severe burn injury in children: a double-blind, randomized, controlled study. Osteoporos Int. 2005;16:631–635.
140 Coté CJ, Drop LJ, Daniels AL, Hoaglin DC. Calcium chloride versus calcium gluconate: comparison of ionization and cardiovascular effects in children and dogs. Anesthesiology. 1987;66:465–470.
141 Klein GL, Chen TC, Holick MF, et al. Synthesis of vitamin D in skin after burns. Lancet. 2004;363:291–292.
142 De Young AC, Kenardy JA, Cobham VE, Kimble R. Prevalence, comorbidity and course of trauma reactions in young burn-injured children. J Child Psychol Psychiatry. 2012;53:56–63.
143 De Young AC, Kenardy JA, Cobham VE. Diagnosis of posttraumatic stress disorder in preschool children. J Clin Child Adolesc Psychol. 2011;40:375–384.
144 Graf A, Schiestl C, Landolt MA. Posttraumatic stress and behavior problems in infants and toddlers with burns. J Pediatr Psychol. 2011;36:923–931.
145 Stoddard FJ, Ronfeldt H, Kagan J, et al. Young burned children: the course of acute stress and physiological and behavioral responses. Am J Psychiatry. 2006;163:1084–1090.
146 Stoddard FJ, Saxe G, Ronfeldt H, et al. Acute stress symptoms in young children with burns. J Am Acad Child Adolesc Psychiatry. 2006;45:87–93.
147 Robert R, Blakeney PE, Villarreal C, Rosenberg L, Meyer WJ, III. Imipramine treatment in pediatric burn patients with symptoms of acute stress disorder: a pilot study. J Am Acad Child Adolesc Psychiatry. 1999;38:873–882.
148 Tcheung WJ, Robert R, Rosenberg L, et al. Early treatment of acute stress disorder in children with major burn injury. Pediatr Crit Care Med. 2005;6:676–681.
149 Robert R, Meyer WJ, III., Villarreal C, et al. An approach to the timely treatment of acute stress disorder. J Burn Care Rehabil. 1999;20:250–258.
150 Robert R, Tcheung WJ, Rosenberg L, et al. Treating thermally injured children suffering symptoms of acute stress with imipramine and fluoxetine: a randomized, double-blind study. Burns. 2008;34:919–928.
151 Meighen KG, Hines LA, Lagges AM. Risperidone treatment of preschool children with thermal burns and acute stress disorder. J Child Adolesc Psychopharmacol. 2007;17:223–232.
152 Thomas CR, Ayoub M, Rosenberg L, Robert RS, Meyer WJ. Attention deficit hyperactivity disorder & pediatric burn injury: a preliminary retrospective study. Burns. 2004;30:221–223.
153 Mangus RS, Bergman D, Zieger M, Coleman JJ. Burn injuries in children with attention-deficit/hyperactivity disorder. Burns. 2004;30:148–150.
154 Martin-Herz SP, Thurber CA, Patterson DR. Psychological principles of burn wound pain in children. II. Treatment applications. J Burn Care Rehabil. 2000;21:458–472.
155 Kendall D, Ehde DM, Patterson DR, Johnson V. Rates of dysfunction in parents of pediatric patients with burns. J Burn Care Rehabil. 1998;19:312–316.
156 Thomas CR, Blakeney P, Holzer CE, III., Meyer WJ, III. Psychiatric disorders in long-term adjustment of at-risk adolescent burn survivors. J Burn Care Res. 2009;30:458–463.
157 Martyn JA, Matteo RS, Greenblatt DJ, Lebowitz PW, Savarese JJ. Pharmacokinetics of d-tubocurarine in patients with thermal injury. Anesth Analg. 1982;61:241–246.
158 Eklund J, Granberg PO, Liljedahl SO. Studies on renal function in burns. I. Renal osmolal regulation, glomerular filtration rate and plasma solute composition related to age, burned surface area and mortality probability. Acta Chir Scand. 1970;136:627–640.
159 Ciaccio EI, Fruncillo RJ. Urinary excretion of D-glucaric acid by severely burned patients. Clin Pharmacol Ther. 1979;25:340–344.
160 Leibel WS, Martyn JA, Szyfelbein SK, Miller KW. Elevated plasma binding cannot account for the burn-related d-tubocurarine hyposensitivity. Anesthesiology. 1981;54:378–382.
161 Martyn JA, Abernethy DR, Greenblatt DJ. Plasma protein binding of drugs after severe burn injury. Clin Pharmacol Ther. 1984;35:535–539.
162 Han T, Harmatz JS, Greenblatt DJ, Martyn JA. Fentanyl clearance and volume of distribution are increased in patients with major burns. J Clin Pharmacol. 2007;47:674–680.
163 Bloedow DC, Goodfellow LA, Marvin J, Heimbach D. Meperidine disposition in burn patients. Res Commun Chem Pathol Pharmacol. 1986;54:87–99.
164 Denson DD, Concilus RR, Warden G, Raj PP. Pharmacokinetics of continuous intravenous infusion of methadone in the early post-burn period. J Clin Pharmacol. 1990;30:70–75.
165 Brunette KE, Anderson BJ, Thomas J, et al. Exploring the pharmacokinetics of oral ketamine in children undergoing burns procedures. Paediatr Anaesth. 2011;21:653–662.
166 Gronert GA, Theye RA. Pathophysiology of hyperkalemia induced by succinylcholine. Anesthesiology. 1975;43:89–99.
167 Ward JM, Martyn JA. Burn injury-induced nicotinic acetylcholine receptor changes on muscle membrane. Muscle Nerve. 1993;16:348–354.
168 Kim C, Martyn J, Fuke N. Burn injury to trunk of rat causes denervation-like responses in the gastrocnemius muscle. J Appl Physiol. 1988;65:1745–1751.
169 Martyn J, Goldhill DR, Goudsouzian NG. Clinical pharmacology of muscle relaxants in patients with burns. J Clin Pharmacol. 1986;26:680–685.
170 Aprille JR, Aikawa N, Bell TC, Bode HH, Malamud DF. Adenylate cyclase after burn injury: resistance to desensitization by catecholamines. J Trauma. 1979;19:812–818.
171 Kim C, Hirose M, Martyn JA. d-Tubocurarine accentuates the burn-induced upregulation of nicotinic acetylcholine receptors at the muscle membrane. Anesthesiology. 1995;83:309–315.
172 Martyn JA, Richtsfeld M. Succinylcholine-induced hyperkalemia in acquired pathologic states: etiologic factors and molecular mechanisms. Anesthesiology. 2006;104:158–169.
173 Wang C, Martyn JA. Burn injury alters beta-adrenergic receptor and second messenger function in rat ventricular muscle. Crit Care Med. 1996;24:118–124.
174 Kim C, Fuke N, Martyn JA. Burn injury to rat increases nicotinic acetylcholine receptors in the diaphragm. Anesthesiology. 1988;68:401–406.
175 Cone JB, Ransom JM, Tucker WE, et al. The effect of dopamine on postburn myocardial depression. J Trauma. 1982;22:1019–1020.
176 Martyn J, Wilson RS, Burke JF. Right ventricular function and pulmonary hemodynamics during dopamine infusion in burned patients. Chest. 1986;89:357–360.
177 Bonate PL. Pathophysiology and pharmacokinetics following burn injury. Clin Pharmacokinet. 1990;18:118–130.
178 Glew RH, Moellering RC, Jr., Burke JF. Gentamicin dosage in children with extensive burns. J Trauma. 1976;16:819–823.
179 Martyn JA, Goudsouzian NG, Matteo RS, et al. Metocurine requirements and plasma concentrations in burned paediatric patients. Br J Anaesth. 1983;55:263–268.
180 Martyn JA, Liu LM, Szyfelbein SK, Ambalavanar ES, Goudsouzian NG. The neuromuscular effects of pancuronium in burned children. Anesthesiology. 1983;59:561–564.
181 Ernst A, Zibrak JD. Carbon monoxide poisoning. N Engl J Med. 1998;339:1603–1608.
182 Pitt RM, Parker JC, Jurkovich GJ, Taylor AE, Curreri PW. Analysis of altered capillary pressure and permeability after thermal injury. J Surg Res. 1987;42:693–702.
183 Davies JW. Toxic chemicals versus lung tissue: an aspect of inhalation injury revisited. The Everett Idris Evans Memorial Lecture, 1986. J Burn Care Rehabil. 1986;7:213–222.
184 Green GM, Jakab GJ, Low RB, Davis GS. Defense mechanisms of the respiratory membrane. Am Rev Respir Dis. 1977;115:479–514.
185 Nieman GF, Clark WR, Wax SD, et al. The effect of smoke inhalation on pulmonary surfactant. Ann Surg. 1980;191:171–181.
186 Shimazu T, Yukioka T, Hubbard GB, et al. A dose-responsive model of smoke inhalation injury: severity-related alteration in cardiopulmonary function. Ann Surg. 1987;206:89–98.
187 Herndon DN, Langner F, Thompson P, et al. Pulmonary injury in burned patients. Surg Clin North Am. 1987;67:31–46.
188 Nieman GF, Clark WRJ, Goyette D, et al. Wood smoke inhalation increases pulmonary microvascular permeability. Surgery. 1989;105:481–487.
189 Narita H, Kikuchi I, Ogata K, et al. Smoke inhalation injury from newer synthetic building materials: a patient who survived 205 days. Burns Incl Therm Inj. 1987;13:147–152.
190 Desai MH, Rutan RL, Herndon DN. Managing smoke inhalation injuries. Postgrad Med. 1989;86:69–76.
191 Stothert JC, Jr., Herndon DN, Lubbesmeyer HJ, et al. Airway acid injury following smoke inhalation. Prog Clin Biol Res. 1988;264:409–413.
192 Wroblewski DA, Bower GC. The significance of facial burns in acute smoke inhalation. Crit Care Med. 1979;7:335–338.
193 Stone HH. Pulmonary burns in children. J Pediatr Surg. 1979;14:48–52.
194 Zawacki BE, Jung RC, Joyce J, Rincon E. Smoke, burns, and the natural history of inhalation injury in fire victims: a correlation of experimental and clinical data. Ann Surg. 1977;185:100–110.
195 Stephenson SF, Esrig BC, Polk HCJ, et al. The pathophysiology of smoke inhalation injury. Ann Surg. 1975;182:652–660.
196 Demling RH. Postgraduate course: respiratory injury. III. Pulmonary dysfunction in the burn patient. J Burn Care Rehabil. 1986;7:277–284.
197 Quinby WC, Jr. Restrictive effects of thoracic burns in children. J Trauma. 1972;12:646–655.
198 Hollingsed TC, Saffle JR, Barton RG, Craft WB, Morris SE. Etiology and consequences of respiratory failure in thermally injured patients. Am J Surg. 1993;166:592–596.
199 Rue LW, Cioffi WG, Mason AD, McManus WF, Pruitt BA, Jr. Improved survival of burned patients with inhalation injury. Arch Surg. 1993;128:772–778.
200 Cancio LC. Airway management and smoke inhalation injury in the burn patient. Clin Plast Surg. 2009;36:555–567.
201 Cancio LC, Batchinsky AI, Dubick MA, et al. Inhalation injury: pathophysiology and clinical care proceedings of a symposium conducted at the Trauma Institute of San Antonio, San Antonio, TX, USA on 28 March 2006. Burns. 2007;33:681–692.
202 Cha SI, Kim CH, Lee JH, et al. Isolated smoke inhalation injuries: acute respiratory dysfunction, clinical outcomes, and short-term evolution of pulmonary functions with the effects of steroids. Burns. 2007;33:200–208.
203 Dai NT, Chen TM, Cheng TY, et al. The comparison of early fluid therapy in extensive flame burns between inhalation and noninhalation injuries. Burns. 1998;24:671–675.
204 Endorf FW, Gamelli RL. Inhalation injury, pulmonary perturbations, and fluid resuscitation. J Burn Care Res. 2007;28:80–83.
205 Hantson P, Butera R, Clemessy JL, Michel A, Baud FJ. Early complications and value of initial clinical and paraclinical observations in victims of smoke inhalation without burns. Chest. 1997;111:671–675.
206 Irrazabal CL, Capdevila AA, Revich L, et al. Early and late complications among 15 victims exposed to indoor fire and smoke inhalation. Burns. 2008;34:533–538.
207 Kasten KR, Makley AT, Kagan RJ. Update on the critical care management of severe burns. J Intensive Care Med. 2011;26:223–236.
208 Marek K, Piotr W, Stanislaw S, et al. Fibreoptic bronchoscopy in routine clinical practice in confirming the diagnosis and treatment of inhalation burns. Burns. 2007;33:554–560.
209 Mlcak R, Cortiella J, Desai M, Herndon D. Lung compliance, airway resistance, and work of breathing in children after inhalation injury. J Burn Care Rehabil. 1997;18:531–534.
210 Mlcak RP, Suman OE, Herndon DN. Respiratory management of inhalation injury. Burns. 2007;33:2–13.
211 Palmieri TL. Inhalation injury: research progress and needs. J Burn Care Res. 2007;28:549–554.
212 Brown DL, Archer SB, Greenhalgh DG, et al. Inhalation injury severity scoring system: a quantitative method. J Burn Care Rehabil. 1996;17:552–557.
213 Ryan CM, Schoenfeld DA, Thorpe WP, et al. Objective estimates of the probability of death from burn injuries. N Engl J Med. 1998;338:362–366.
214 Sheridan R, Remensnyder J, Prelack K, Petras L, Lydon M. Treatment of the seriously burned infant. J Burn Care Rehabil. 1998;19:115–118.
215 Morrow SE, Smith DL, Cairns BA, et al. Etiology and outcome of pediatric burns. J Pediatr Surg. 1996;31:329–333.
216 Eckhauser FE, Billote J, Burke JF, Quinby WC. Tracheostomy complicating massive burn injury: a plea for conservatism. Am J Surg. 1974;127:418–423.
217 Silver GM, Freiburg C, Halerz M, et al. A survey of airway and ventilator management strategies in North American pediatric burn units. J Burn Care Rehabil. 2004;25:435–440.
218 Palmieri TL, Jackson W, Greenhalgh DG. Benefits of early tracheostomy in severely burned children. Crit Care Med. 2002;30:922–924.
219 Barret JP, Desai MH, Herndon DN. Effects of tracheostomies on infection and airway complications in pediatric burn patients. Burns. 2000;26:190–193.
220 Sheridan RL. Uncuffed endotracheal tubes should not be used in seriously burned children. Pediatr Crit Care Med. 2006;7:258–259.
221 Weiss M, Dullenkopf A. Cuffed tracheal tubes in children: past, present and future. Expert Rev Med Devices. 2007;4:73–82.
222 Weiss M, Dullenkopf A, Fischer JE, Keller C, Gerber AC. Prospective randomized controlled multi-centre trial of cuffed or uncuffed endotracheal tubes in small children. Br J Anaesth. 2009;103:867–873.
223 Sheridan RL, Kacmarek RM, McEttrick MM, et al. Permissive hypercapnia as a ventilatory strategy in burned children: effect on barotrauma, pneumonia, and mortality. J Trauma. 1995;39:854–859.
224 Dullenkopf A, Schmitz A, Frei M, Gerber AC, Weiss M. Air leakage around endotracheal tube cuffs. Eur J Anaesth. 2004;21:448–453.
225 Dullenkopf A, Gerber A, Weiss M. Fluid leakage past tracheal tube cuffs: evaluation of the new Microcuff endotracheal tube. Intensive Care Med. 2003;29:1849–1853.
226 Dullenkopf A, Schmitz A, Gerber AC, Weiss M. Tracheal sealing characteristics of pediatric cuffed tracheal tubes. Paediatr Anaesth. 2004;14:825–830.
227 Dullenkopf A, Gerber AC, Weiss M. Fit and seal characteristics of a new paediatric tracheal tube with high volume-low pressure polyurethane cuff. Acta Anaesthesiol Scand. 2005;49:232–237.
228 Weiss M, Balmer C, Dullenkopf A, et al. Intubation depth markings allow an improved positioning of endotracheal tubes in children: [Le marquage de la profondeur d’insertion permet d’ameliorer la mise en place de tubes endotracheaux chez les enfants]. Can J Anaesth. 2005;52:721–726.
229 Shank ES, Muth CM. Decompression illness, iatrogenic gas embolism, and carbon monoxide poisoning: the role of hyperbaric oxygen therapy. Int Anesth Clin. 2000;38:111–138.
230 Kao LW, Nanagas KA. Toxicity associated with carbon monoxide. Clin Lab Med. 2006;26:99–125.
231 Hardy KR, Thom SR. Pathophysiology and treatment of carbon monoxide poisoning. J Toxicol Clin Toxicol. 1994;32:613–629.
232 Meert KL, Heidemann SM, Sarnaik AP. Outcome of children with carbon monoxide poisoning treated with normobaric oxygen. J Trauma. 1998;44:149–154.
233 Walker AR. Emergency department management of house fire burns and carbon monoxide poisoning in children. Curr Opin Pediatr. 1996;8:239–242.
234 Barker SJ, Tremper KK. The effect of carbon monoxide inhalation on pulse oximetry and transcutaneous PO2. Anesthesiology. 1987;66:677–679.
235 Barker SJ, Tremper KK, Hyatt J. Effects of methemoglobinemia on pulse oximetry and mixed venous oximetry. Anesthesiology. 1989;70:112–117.
236 Tremper KK, Barker SJ. Pulse oximetry. Anesthesiology. 1989;70:98–108.
237 Roth D, Herkner H, Schreiber W, et al. Accuracy of noninvasive multiwave pulse oximetry compared with carboxyhemoglobin from blood gas analysis in unselected emergency department patients. Ann Emerg Med. 2011;58:74–79.
238 Roth D, Hubmann N, Havel C, et al. Victim of carbon monoxide poisoning identified by carbon monoxide oximetry. J Emerg Med. 2011;40:640–642.
239 Barker SJ, Curry J, Redford D, Morgan S. Measurement of carboxyhemoglobin and methemoglobin by pulse oximetry: a human volunteer study. Anesthesiology. 2006;105:892–897.
240 Haldane J, Loraine-Smith J. The absorption of oxygen by the lungs. J Physiol. 1897;22:231–258.
241 Douglas CG, Haldane JS, Haldane JBS. The laws of combination of hemoglobin with carbon monoxide and oxygen. J Physiol. 1912;44:275–304.
242 Stewart RD, Stewart RS, Stamm W, Seelen RP. Rapid estimation of carboxyhemoglobin level in fire fighters. JAMA. 1976;235:390–392.
243 Ginsberg MD. Carbon monoxide intoxication: clinical features, neuropathology and mechanisms of injury. J Toxicol Clin Toxicol. 1985;23:281–288.
244 Gorman DF, Runciman WB. Carbon monoxide poisoning. Anaesth Intensive Care. 1991;19:506–511.
245 Keenan HT, Bratton SL, Norkool DM, Brogan TV, Hampson NB. Delivery of hyperbaric oxygen therapy to critically ill, mechanically ventilated children. J Crit Care. 1998;13:7–12.
246 Brannen AL, Still J, Haynes M, et al. A randomized prospective trial of hyperbaric oxygen in a referral burn center population. Am Surg. 1997;63:205–208.
247 Villanueva E, Bennett MH, Wasiak J, Lehm JP. Hyperbaric oxygen therapy for thermal burns. Cochrane Database Syst Rev. 2004. CD004727
248 Prockop LD, Chichkova RI. Carbon monoxide intoxication: an updated review. J Neurol Sci. 2007;262:122–130.
249 Stoller KP. Hyperbaric oxygen and carbon monoxide poisoning: a critical review. Neurol Res. 2007;29:146–155.
250 Thom SR. Hyperbaric oxygen therapy for carbon monoxide poisoning: is it time to end the debates? Toxicol Rev. 2005;24:157–158.
251 Buckley NA, Juurlink DN, Isbister G, Bennett MH, Lavonas EJ. Hyperbaric oxygen for carbon monoxide poisoning. Cochrane Database Syst Rev. 2011. CD002041
252 Olson KR. Hyperbaric oxygen or normobaric oxygen? Toxicol Rev. 2005;24:151–160.
253 Olson KR, Seger D. Hyperbaric oxygen for carbon monoxide poisoning: does it really work? Ann Emerg Med. 1995;25:535–537.
254 Buehler JH, Berns AS, Webster JR, Addington WW, Cugell DW. Lactic acidosis from carboxyhemoglobinemia after smoke inhalation. Ann Intern Med. 1975;82:803–805.
255 Tibbles PM, Edelsberg JS. Hyperbaric-oxygen therapy. N Engl J Med. 1996;334:1642–1648.
256 Tibbles PM, Perrotta PL. Treatment of carbon monoxide poisoning: a critical review of human outcome studies comparing normobaric oxygen with hyperbaric oxygen. Ann Emerg Med. 1994;24:269–276.
257 Chang KH, Han MH, Kim HS, Wie BA, Han MC. Delayed encephalopathy after acute carbon monoxide intoxication: MR imaging features and distribution of cerebral white matter lesions. Radiology. 1992;184:117–122.
258 Kim JH, Chang KH, Song IC, et al. Delayed encephalopathy of acute carbon monoxide intoxication: diffusivity of cerebral white matter lesions. AJNR Am J Neuroradiol. 2003;24:1592–1597.
259 Buckley NA, Isbister GK, Stokes B, Juurlink DN. Hyperbaric oxygen for carbon monoxide poisoning: a systematic review and critical analysis of the evidence. Toxicol Rev. 2005;24:75–92.
260 Chou KJ, Fisher JL, Silver EJ. Characteristics and outcome of children with carbon monoxide poisoning with and without smoke exposure referred for hyperbaric oxygen therapy. Pediatr Emerg Care. 2000;16:151–155.
261 Jasper BW, Hopkins RO, Duker HV, Weaver LK. Affective outcome following carbon monoxide poisoning: a prospective longitudinal study. Cogn Behav Neurol. 2005;18:127–134.
262 Scheinkestel CD, Jones K, Myles PS, et al. Where to now with carbon monoxide poisoning? Emerg Med Australas. 2004;16:151–154.
263 Scheinkestel CD, Bailey M, Myles PS, et al. Hyperbaric or normobaric oxygen for acute carbon monoxide poisoning: a randomized controlled clinical trial. Undersea Hyperb Med. 2000;27:163–164.
264 Scheinkestel CD, Bailey M, Myles PS, et al. Hyperbaric or normobaric oxygen for acute carbon monoxide poisoning: a randomised controlled clinical trial. Med J Aust. 1999;170:203–210.
265 Weaver LK, Hopkins RO, Chan KJ, et al. Carbon Monoxide Research Group, LDS Hospital, Utah in reply to Scheinkestel et al. and Emerson: the role of hyperbaric oxygen in carbon monoxide poisoning. Emerg Med Australas. 2004;16:394–399.
266 Weaver LK, Hopkins RO, Chan KJ, et al. Hyperbaric oxygen for acute carbon monoxide poisoning. N Engl J Med. 2002;347:1057–1067.
267 Yarar C, Yakut A, Akin A, Yildiz B, Dinleyici EC. Analysis of the features of acute carbon monoxide poisoning and hyperbaric oxygen therapy in children. Turk J Pediatr. 2008;50:235–241.
268 Chang DC, Lee JT, Lo CP, et al. Hyperbaric oxygen ameliorates delayed neuropsychiatric syndrome of carbon monoxide poisoning. Undersea Hyperb Med. 2010;37:23–33.
269 Choi IS. Delayed neurologic sequelae in carbon monoxide intoxication. Arch Neurol. 1983;40:433–435.
270 Mathieu D, Nolf M, Durocher A, et al. Acute carbon monoxide poisoning: risk of late sequelae and treatment by hyperbaric oxygen. J Toxicol Clin Toxicol. 1985;23:315–324.
271 Myers RA, Snyder SK, Emhoff TA. Subacute sequelae of carbon monoxide poisoning. Ann Emerg Med. 1985;14:1163–1167.
272 Werner B, Back W, Akerblom H, Barr PO. Two cases of acute carbon monoxide poisoning with delayed neurological sequelae after a “free” interval. J Toxicol Clin Toxicol. 1985;23:249–265.
273 Thom SR, Bhopale VM, Fisher D. Hyperbaric oxygen reduces delayed immune-mediated neuropathology in experimental carbon monoxide toxicity. Toxicol Appl Pharmacol. 2006;213:152–159.
274 Thom SR, Bhopale VM, Fisher D, Zhang J, Gimotty P. Delayed neuropathology after carbon monoxide poisoning is immune-mediated. Proc Natl Acad Sci U S A. 2004;101:13660–13665.
275 Neufeld MY, Swanson JW, Klass DW. Localized EEG abnormalities in acute carbon monoxide poisoning. Arch Neurol. 1981;38:524–527.
276 Myers RA, Britten JS. Are arterial blood gases of value in treatment decisions for carbon monoxide poisoning? Crit Care Med. 1989;17:139–142.
277 Hampson NB, Hauff NM. Carboxyhemoglobin levels in carbon monoxide poisoning: do they correlate with the clinical picture? Am J Emerg Med. 2008;26:665–669.
278 Thom SR, Keim LW. Carbon monoxide poisoning: a review epidemiology, pathophysiology, clinical findings, and treatment options including hyperbaric oxygen therapy. J Toxicol Clin Toxicol. 1989;27:141–156.
279 Thom SR, Taber RL, Mendiguren, II., et al. Delayed neuropsychologic sequelae after carbon monoxide poisoning: prevention by treatment with hyperbaric oxygen. Ann Emerg Med. 1995;25:474–480.
280 Norkool DM, Kirkpatrick JN. Treatment of acute carbon monoxide poisoning with hyperbaric oxygen: a review of 115 cases. Ann Emerg Med. 1985;14:1168–1171.
281 Grube BJ, Marvin JA, Heimbach DM. Therapeutic hyperbaric oxygen: help or hindrance in burn patients with carbon monoxide poisoning? J Burn Care Rehabil. 1988;9:249–252.
282 Sloan EP, Murphy DG, Hart R, et al. Complications and protocol considerations in carbon monoxide-poisoned patients who require hyperbaric oxygen therapy: report from a ten-year experience. Ann Emerg Med. 1989;18:629–634.
283 Lawson-Smith P, Jansen EC, Hilsted L, Johnsen AH, Hyldegaard O. Effect of acute and delayed hyperbaric oxygen therapy on cyanide whole blood levels during acute cyanide intoxication. Undersea Hyperb Med. 2011;38:17–26.
284 Silverman SH, Purdue GF, Hunt JL, Bost RO. Cyanide toxicity in burned patients. J Trauma. 1988;28:171–176.
285 Moncrief JA. Medical progress: burns. N Engl J Med. 1973;288:444–454.
286 Wilmore DW, Long JM, Mason AD, Jr., Skreen RW, Pruitt BA, Jr. Catecholamines: mediator of the hypermetabolic response to thermal injury. Ann Surg. 1974;180:653–669.
287 Wisnicki JL, Sato RM, Baxter CR. Current concepts in burn care. Ann Plast Surg. 1986;16:242–249.
288 Baxter C. Fluid resuscitation, burn percentage, and physiologic age. J Trauma. 1979;19:864–865.
289 Baxter CR. Fluid volume and electrolyte changes of the early postburn period. Clin Plast Surg. 1974;1:693–703.
290 Bowser-Wallace BH, Caldwell FT, Jr. A prospective analysis of hypertonic lactated saline v. Ringer’s lactate-colloid for the resuscitation of severely burned children. Burns Incl Therm Inj. 1986;12:402–409.
291 Bowser-Wallace BH, Caldwell FT, Jr. Fluid requirements of severely burned children up to 3 years old: hypertonic lactated saline vs. Ringer’s lactate-colloid. Burns Incl Therm Inj. 1986;12:549–555.
292 Caldwell FT, Bowser BH. Critical evaluation of hypertonic and hypotonic solutions to resuscitate severely burned children: a prospective study. Ann Surg.. 1979;189:546–552.
293 Jelenko C, 3rd., Williams JB, Wheeler ML, et al. Studies in shock and resuscitation, I: use of a hypertonic, albumin-containing, fluid demand regimen (HALFD) in resuscitation. Crit Care Med. 1979;7:157–167.
294 Monafo WW, Chuntrasakul C, Ayvazian VH. Hypertonic sodium solutions in the treatment of burn shock. Am J Surg. 1973;126:778–783.
295 Boswick JA, Jr., Thompson JD, Kershner CJ. Critical care of the burned patient. Anesthesiology. 1977;47:164–170.
296 Carvajal HF, Parks DH. Optimal composition of burn resuscitation fluids. Crit Care Med. 1988;16:695–700.
297 Graves TA, Cioffi WG, McManus WF, Mason AD, Jr., Pruitt BA, Jr. Fluid resuscitation of infants and children with massive thermal injury. J Trauma. 1988;28:1656–1659.
298 Merrell SW, Saffle JR, Sullivan JJ, et al. Fluid resuscitation in thermally injured children. Am J Surg. 1986;152:664–669.
299 Lawrence A, Faraklas I, Watkins H, et al. Colloid administration normalizes resuscitation ratio and ameliorates “fluid creep.”. J Burn Care Res. 2010;31:40–47.
300 Horton JW, White J, Baxter CR, White DJ. Hypertonic saline dextran resuscitation of thermal injury. Ann Surg. 1990;211:301–311.
301 McAlister V, Burns KE, Znajda T, Church B. Hypertonic saline for peri-operative fluid management. Cochrane Database Syst Rev. 2010. CD005576
302 Faraklas I, Lam U, Cochran A, Stoddard G, Saffle J. Colloid normalizes resuscitation ratio in pediatric burns. J Burn Care Res. 2011;32:91–97.
303 Lawrence A, Faraklas I, Watkins H, et al. Colloid administration normalizes resuscitation ratio and ameliorates “fluid creep.”. J Burn Care Res. 2010;31:40–47.
304 Shirani KZ, Vaughan GM, Mason AD, Jr., Pruitt BA, Jr. Update on current therapeutic approaches in burns. Shock. 1996;5:4–16.
305 Martyn JA, McKusick K, Strauss HW, Burke JF. Ventricular volume and ejection fraction in the diagnosis of the aetiology of low cardiac output in burned patients. Anaesthesia. 1986;41:511–515.
306 Hoffman MJ, Greenfield LJ, Sugerman HJ, Tatum JL. Unsuspected right ventricular dysfunction in shock and sepsis. Ann Surg. 1983;198:307–319.
307 Steinberg JM, Schiller HJ, Tsvaygenbaum B, et al. Wood smoke inhalation causes alveolar instability in a dose-dependent fashion. Respir Care. 2005;50:1062–1070.
308 Gallagher JJ. Intra-abdominal hypertension: detecting and managing a lethal complication of critical illness. AACN Adv Crit Care. 2010;21:205–219.
309 Yeroshalmi F, Sidoti EJ, Jr., Adamo AK, Lieberman BL, Badner VM. Oral electrical burns in children: a model of multidisciplinary care. J Burn Care Res. 2011;32:e25–e30.
310 Choi M, Armstrong MB, Panthaki ZJ. Pediatric hand burns: thermal, electrical, chemical. J Craniofac Surg. 2009;20:1045–1048.
311 Zubair M, Besner GE. Pediatric electrical burns: management strategies. Burns. 1997;23:413–420.
312 Garcia CT, Smith GA, Cohen DM, Fernandez K. Electrical injuries in a pediatric emergency department. Ann Emerg Med. 1995;26:604–608.
313 Wallace BH, Cone JB, Vanderpool RD, et al. Retrospective evaluation of admission criteria for paediatric electrical injuries. Burns. 1995;21:590–593.
314 Hammond JS, Ward CG. High-voltage electrical injuries: management and outcome of 60 cases. South Med J. 1988;81:1351–1352.
315 Solem L, Fischer RP, Strate RG. The natural history of electrical injury. J Trauma. 1977;17:487–492.
316 Arnoldo B, Klein M, Gibran NS. Practice guidelines for the management of electrical injuries. J Burn Care Res. 2006;27:439–447.
317 Dixon GF. The evaluation and management of electrical injuries. Crit Care Med. 1983;11:384–387.
318 Lamb JD. Anaesthetic considerations for major thermal injury. Can Anaesth Soc J. 1985;32:84–92.
319 Prelack K, Dylewski M, Sheridan RL. Practical guidelines for nutritional management of burn injury and recovery. Burns. 2007;33:14–24.
320 Osgood PF, Szyfelbein SK. Management of burn pain in children. Pediatr Clin North Am. 1989;36:1001–1013.
321 Sheridan RL, Hinson M, Nackel A, et al. Development of a pediatric burn pain and anxiety management program. J Burn Care Rehabil. 1997;18:455–459.
322 Browne DA, de Boeck R, Morgan M. An evaluation of the Level 1 blood warmer series. Anaesthesia. 1990;45:960–963.
323 Presson RG, Jr., Haselby KA, Bezruczko AP, Barnett E. Evaluation of a new high-efficiency blood warmer for children. Anesthesiology. 1990;73:173–176.
324 Rothen HU, Lauber R, Mosimann M. An evaluation of the Rapid Infusion System. Anaesthesia. 1992;47:597–600.
325 Coté CJ, Petkau AJ. Thiopental requirements may be increased in children reanesthetized at least one year after recovery from extensive thermal injury. Anesth Analg. 1985;64:1156–1160.
326 White PF, Way WL, Trevor AJ. Ketamine: its pharmacology and therapeutic uses. Anesthesiology. 1982;56:119–136.
327 Fidkowski CW, Fuzaylov G, Sheridan RL, Coté CJ. Inhalation burn injury in children. Paediatr Anaesth. 2009;19(Suppl 1):147–154.
328 Martyn JA, Fukushima Y, Chon JY, Yang HS. Muscle relaxants in burns, trauma, and critical illness. Int Anesthesiol Clin. 2006;44:123–143.
329 Martyn JA, Matteo RS, Szyfelbein SK, Kaplan RF. Unprecedented resistance to neuromuscular blocking effects of metocurine with persistence after complete recovery in a burned patient. Anesth Analg. 1982;61:614–617.
330 Mazurek AJ, Rae B, Hann S, et al. Rocuronium versus succinylcholine: are they equally effective during rapid-sequence induction of anesthesia? Anesth Analg. 1998;87:1259–1262.
331 Mills AK, Martyn JA. Evaluation of atracurium neuromuscular blockade in paediatric patients with burn injury. Br J Anaesth. 1988;60:450–455.
332 Mills AK, Martyn JA. Neuromuscular blockade with vecuronium in paediatric patients with burn injury. Br J Clin Pharmacol. 1989;28:155–159.
333 Satwicz PR, Martyn JA, Szyfelbein SK, Firestone S. Potentiation of neuromuscular blockade using a combination of pancuronium and dimethyltubocurarine: studies in children following acute burn injury or during reconstructive surgery. Br J Anaesth. 1984;56:479–484.
334 Han T, Kim H, Bae J, Kim K, Martyn JA. Neuromuscular pharmacodynamics of rocuronium in patients with major burns. Anesth Analg. 2004;99:386–392.
335 Owens VF, Palmieri TL, Comroe CM, et al. Ketamine: a safe and effective agent for painful procedures in the pediatric burn patient. J Burn Care Res. 2006;27:211–216.
336 Himmelseher S, Durieux ME. Ketamine for perioperative pain management. Anesthesiology. 2005;102:211–220.
337 White MC, Karsli C. Long-term use of an intravenous ketamine infusion in a child with significant burns. Paediatr Anaesth. 2007;17:1102–1104.
338 Bayat A, Ramaiah R, Bhananker SM. Analgesia and sedation for children undergoing burn wound care. Expert Rev Neurother. 2010;10:1747–1759.
339 Tosun Z, Esmaoglu A, Coruh A. Propofol-ketamine vs propofol-fentanyl combinations for deep sedation and analgesia in pediatric patients undergoing burn dressing changes. Paediatr Anaesth. 2008;18:43–47.
340 Owens VF, Palmieri TL, Comroe CM. Ketamine: a safe and effective agent for painful procedures in the pediatric burn patient. J Burn Care Res. 2006;27:211–216.
341 Lin H, Faraklas I, Sampson C, Saffle JR, Cochran A. Use of dexmedetomidine for sedation in critically ill mechanically ventilated pediatric burn patients. J Burn Care Res. 2011;32:98–103.
342 Coté CJ, Goldstein EA, Fuchsman WH, Hoaglin DC. The effect of nail polish on pulse oximetry. Anesth Analg. 1988;67:683–686.
343 Coté CJ, Goldstein EA, Coté MA, Hoaglin DC, Ryan JF. A single-blind study of pulse oximetry in children. Anesthesiology. 1988;68:184–188.
344 Coté CJ, Daniels AL, Connolly M, Szyfelbein SK, Wickens CD. Tongue oximetry in children with extensive thermal injury: comparison with peripheral oximetry. Can J Anaesth. 1992;39:454–457.
345 Jobes DR, Nicolson SC. Monitoring of arterial hemoglobin oxygen saturation using a tongue sensor. Anesth Analg. 1988;67:186–188.
346 Sheridan RL, Prelack KM, Petras LM, Szyfelbein SK, Tompkins RG. Intraoperative reflectance oximetry in burn patients. J Clin Monit. 1995;11:32–34.
347 Sheridan RL, Szyfelbein SK, Petras L, Lydon M. Staged high dose epinephrine clysis in pediatric burn excisions. J Burn Care Rehabil. 1998;19:S199.
348 Losee JE, Fox I, Hua LB, Cladis FP, Serletti JM. Transfusion-free pediatric burn surgery: techniques and strategies. Ann Plast Surg. 2005;54:165–171.
349 Sheridan RL, Hurley J, Smith MA, et al. The acutely burned hand: management and outcome based on a ten-year experience with 1047 acute hand burns. J Trauma. 1995;38:406–411.
350 Tompkins RG, Remensnyder JP, Burke JF, et al. Significant reductions in mortality for children with burn injuries through the use of prompt eschar excision. Ann Surg. 1988;208:577–585.
351 Sheridan RL, Tompkins RG, Burke JF. Management of burn wounds with prompt excision and immediate closure. J Intensive Care Med. 1994;9:6–19.
352 Moran KT, O’Reilly TJ, Furman W, Munster AM. A new algorithm for calculation of blood loss in excisional burn surgery. Am Surg. 1988;54:207–208.
353 Housinger TA, Lang D, Warden GD. A prospective study of blood loss with excisional therapy in pediatric burn patients. J Trauma. 1993;34:262–263.
354 Rutan RL, Bjarnason DL, Desai MH, Herndon DN. Incidence of HIV seroconversion in paediatric burn patients. Burns. 1992;18:216–219.
355 Morris JAJ, Wilcox TR, Reed GW, et al. Safety of the blood supply: surrogate testing and transmission of hepatitis C in patients after massive transfusion. Ann Surg. 1994;219:517–525.
356 Fischer CG, Lloyd S, Kopcha R, Warden GD, McCall JE. The safety of adding bupivacaine to the subcutaneous infiltration solution used for donor site harvest. J Burn Care Rehabil. 2003;24:361–364.
357 Desai MH, Herndon DN, Broemeling L, et al. Early burn wound excision significantly reduces blood loss. Ann Surg. 1990;211:753–759.
358 Budny PG, Regan PJ, Roberts AH. The estimation of blood loss during burns surgery. Burns. 1993;19:134–137.
359 Kahn RC, Jascott D, Carlon GC, et al. Massive blood replacement: correlation of ionized calcium, citrate, and hydrogen ion concentration. Anesth Analg. 1979;58:274–278.
360 Stulz PM, Scheidegger D, Drop LJ, et al. Ventricular pump performance during hypocalcemia: clinical and experimental studies. J Thorac Cardiovasc Surg. 1979;78:185–194.
361 Denlinger JK, Nahrwold ML, Gibbs PS, Lecky JH. Hypocalcaemia during rapid blood transfusion in anaesthetized man. Br J Anaesth. 1976;48:995–1000.
362 Coté CJ, Drop LJ, Hoaglin DC, Daniels AL, Young ET. Ionized hypocalcemia after fresh frozen plasma administration to thermally injured children: effects of infusion rate, duration, and treatment with calcium chloride. Anesth Analg. 1988;67:152–160.
363 Coté CJ. Depth of halothane anesthesia potentiates citrate-induced ionized hypocalcemia and adverse cardiovascular events in dogs. Anesthesiology. 1987;67:676–680.
364 Marathe PH, Dwersteg JF, Pavlin EG, et al. Effect of thermal injury on the pharmacokinetics and pharmacodynamics of atracurium in humans. Anesthesiology. 1989;70:752–755.
365 Haastrup AA, Mendez P, Coté CJ. Suction the tongue: a new adjunct for improving the laryngeal view for fiberoptic intubation. Anesth Analg. 2011;112:1512–1513.
366 Phillips GD. Total parenteral nutrition in acute illness. Anaesth Intensive Care. 1985;13:288–299.
367 Sheridan RL, Weber JM. Mechanical and infectious complications of central venous cannulation in children: lessons learned from a 10-year experience placing over 1000 catheters. J Burn Care Res. 2006;27:713–718.
368 Sites BD, Macfarlane AJ, Sites VR, et al. Clinical sonopathology for the regional anesthesiologist. I. Vascular and neural. Reg Anesth Pain Med. 2010;35:272–280.
369 Ng I, Vaghadia H, Choi PT, Helmy N. Ultrasound imaging accurately identifies the lateral femoral cutaneous nerve. Anesth Analg. 2008;107:1070–1074.
370 Damarey B, Demondion X, Boutry N, et al. Sonographic assessment of the lateral femoral cutaneous nerve. J Clin Ultrasound. 2009;37:89–95.
371 Shank ES, Martyn JA, Keaney T, Driscoll D. Ultrasound guided blocks of the lateral femoral cutaneous nerve in pediatric reconstructive burn patients. Personal communication. 2011.
372 Lichtenstein D, Meziere G, Biderman P, Gepner A. The “lung point”: an ultrasound sign specific to pneumothorax. Intensive Care Med. 2000;26:1434–1440.
373 Lichtenstein D, Meziere G, Biderman P, Gepner A. The comet-tail artifact: an ultrasound sign ruling out pneumothorax. Intensive Care Med. 1999;25:383–388.
374 Lichtenstein D, Meziere G. Ultrasound probably has a bright future in the diagnosis of pneumothorax. J Trauma. 2002;52:607.
375 Gunst M, Ghaemmaghami V, Sperry J, et al. Accuracy of cardiac function and volume status estimates using the bedside echocardiographic assessment in trauma/critical care. J Trauma. 2008;65:509–516.
376 Gunst M, Sperry J, Ghaemmaghami V, et al. Bedside echocardiographic assessment for trauma/critical care: the BEAT exam. J Am Coll Surg. 2008;207:e1–e3.
377 Kavanagh C. Psychological intervention with the severely burned child: report of an experimental comparison of two approaches and their effects on psychological sequelae. J Am Acad Child Psychiatry. 1983;22:145–156.
378 Kavanagh C. A new approach to dressing change in the severely burned child and its effect on burn-related psychopathology. Heart Lung. 1983;12:612–619.
379 Perry S, Heidrich G. Management of pain during debridement: a survey of U.S. burn units. Pain. 1982;13:267–280.
380 Porter J, Jick H. Addiction rare in patients treated with narcotics. N Engl J Med. 1980;302:123.
381 Goodman JE, McGrath PJ. The epidemiology of pain in children and adolescents: a review. Pain. 1991;46:247–264.
382 McGrath PJ, Finley GA. Attitudes and beliefs about medication and pain management in children. J Palliat Care. 1996;12:46–50.
383 Wang S, Lim G, Yang L, et al. A rat model of unilateral hindpaw burn injury: slowly developing rightwards shift of the morphine dose-response curve. Pain. 2005;116:87–95.
384 Angst MS, Clark JD. Opioid-induced hyperalgesia: a qualitative systematic review. Anesthesiology. 2006;104:570–587.
385 Alexander M, Daniel T, Chaudry IH, Schwacha MG. Opiate analgesics contribute to the development of post-injury immunosuppression. J Surg Res. 2005;129:161–168.
386 Walker J, Maccallum M, Fischer C, et al. Sedation using dexmedetomidine in pediatric burn patients. J Burn Care Res. 2006;27:206–210.
387 Turan A, Memis D, Kaya G, Karamanlioglu B. The prevention of pain from injection of propofol by dexmedetomidine and comparison with lidocaine. Can J Anaesth. 2005;52:548–549.
388 Kontinen VK, Paananen S, Kalso E. The effects of the alpha2-adrenergic agonist, dexmedetomidine, in the spinal nerve ligation model of neuropathic pain in rats. Anesth Analg. 1998;86:355–360.
389 Shimode N, Fukuoka T, Tanimoto M, et al. The effects of dexmedetomidine and halothane on Fos expression in the spinal dorsal horn using a rat postoperative pain model. Neurosci Lett. 2003;343:45–48.
390 Unlugenc H, Gunduz M, Guler T, Yagmur O, Isik G. The effect of pre-anaesthetic administration of intravenous dexmedetomidine on postoperative pain in patients receiving patient-controlled morphine. Eur J Anaesthesiol. 2005;22:386–391.
391 Aho MS, Erkola OA, Scheinin H, Lehtinen AM, Korttila KT. Effect of intravenously administered dexmedetomidine on pain after laparoscopic tubal ligation. Anesth Analg. 1991;73:112–118.
392 Weinbroum AA, Gorodezky A, Niv D, et al. Dextromethorphan attenuation of postoperative pain and primary and secondary thermal hyperalgesia. Can J Anaesth. 2001;48:167–174.
393 Cuignet O, Pirson J, Soudon O, Zizi M. Effects of gabapentin on morphine consumption and pain in severely burned patients. Burns. 2007;33:81–86.
394 Reynolds LW, Hoo RK, Brill RJ, et al. The COX-2 specific inhibitor, valdecoxib, is an effective, opioid-sparing analgesic in patients undergoing total knee arthroplasty. J Pain Symptom Manage. 2003;25:133–141.
395 Sim R, Cheong DM, Wong KS, Lee BM, Liew QY. Prospective randomized, double-blind, placebo-controlled study of pre- and postoperative administration of a COX-2-specific inhibitor as opioid-sparing analgesia in major colorectal surgery. Colorectal Dis. 2007;9:52–60.
396 Camu F, Beecher T, Recker DP, Verburg KM. Valdecoxib, a COX-2-specific inhibitor, is an efficacious, opioid-sparing analgesic in patients undergoing hip arthroplasty. Am J Ther. 2002;9:43–51.
397 Weinbroum AA, Ben-Abraham R. Dextromethorphan and dexmedetomidine: new agents for the control of perioperative pain. Eur J Surg. 2001;167:563–569.
398 Shank ES, Sheridan RL, Ryan CM, Keaney TJ, Martyn JA. Hemodynamic responses to dexmedetomidine in critically ill intubated pediatric burned patients: a preliminary study. J Burn Care Res. 2012. Aug 27. [Epub ahead of print]
399 Walker J, Maccallum M, Fischer C, et al. Sedation using dexmedetomidine in pediatric burn patients. J Burn Care Res. 2006;27:206–210.
400 Lin H, Faraklas I, Sampson C, Saffle JR, Cochran A. Use of dexmedetomidine for sedation in critically ill mechanically ventilated pediatric burn patients. J Burn Care Res. 2011;32:98–103.
401 Diaz SM, Rodarte A, Foley J, Capparelli EV. Pharmacokinetics of dexmedetomidine in postsurgical pediatric intensive care unit patients: preliminary study. Pediatr Crit Care Med. 2007;8:419–424.
402 Potts AL, Anderson BJ, Warman GR, et al. Dexmedetomidine pharmacokinetics in pediatric intensive care: a pooled analysis. Paediatr Anaesth. 2009;19:1119–1129.
403 Potts AL, Warman GR, Anderson BJ. Dexmedetomidine disposition in children: a population analysis. Paediatr Anaesth. 2008;18:722–730.
404 Gunduz M, Sakalli S, Gunes Y, et al. Comparison of effects of ketamine, ketamine-dexmedetomidine and ketamine-midazolam on dressing changes of burn patients. J Anaesthesiol Clin Pharmacol. 2011;27:220–224.
405 Talon MD, Woodson LC, Sherwood ER, et al. Intranasal dexmedetomidine premedication is comparable with midazolam in burn children undergoing reconstructive surgery. J Burn Care Res. 2009;30:599–605.
406 Iirola T, Vilo S, Manner T, et al. Bioavailability of dexmedetomidine after intranasal administration. Eur J Clin Pharmacol. 2011;67:825–831.
407 Borland ML, Bergesio R, Pascoe EM, Turner S, Woodger S. Intranasal fentanyl is an equivalent analgesic to oral morphine in paediatric burns patients for dressing changes: a randomised double blind crossover study. Burns. 2005;31:831–837.
408 Steele E, Grimmer K, Thomas B, et al. Virtual reality as a pediatric pain modulation technique: a case study. Cyberpsychol Behav. 2003;6:633–638.
409 Hoffman HG, Doctor JN, Patterson DR, Carrougher GJ, Furness TA, III. Virtual reality as an adjunctive pain control during burn wound care in adolescent patients. Pain. 2000;85:305–309.
410 Whitehead-Pleaux AM, Baryza MJ, Sheridan RL. The effects of music therapy on pediatric patients’ pain and anxiety during donor site dressing change. J Music Ther. 2006;43:136–153.
411 Foertsch CE, O’Hara MW, Stoddard FJ, Kealey GP. Treatment-resistant pain and distress during pediatric burn-dressing changes. J Burn Care Rehabil. 1998;19:219–224.
412 Stoddard FJ. Coping with pain: a developmental approach to treatment of burned children. Am J Psychiatry. 1982;139:736–740.
413 Roca RP, Spence RJ, Munster AM. Posttraumatic adaptation and distress among adult burn survivors. Am J Psychiatry. 1992;149:1234–1238.
414 Stoddard FJ, Sheridan RL, Saxe GN, et al. Treatment of pain in acutely burned children. J Burn Care Rehabil. 2002;23:135–156.
415 Schechter NL, Blankson V, Pachter LM, Sullivan CM, Costa L. The ouchless place: no pain, children’s gain. Pediatrics. 1997;99:890–894.
416 Weisman SJ, Schechter NL. The management of pain in children. Pediatr Rev. 1991;12:237–243.
417 Walco GA, Cassidy RC, Schechter NL. Pain, hurt, and harm: the ethics of pain control in infants and children. N Engl J Med. 1994;331:541–544.
418 Zeltzer LK, Anderson CT, Schechter NL. Pediatric pain: current status and new directions. Curr Probl Pediatr. 1990;20:409–486.
*References 2, 68, 85, 105, 121, 126–132.
†References 14, 17, 18, 38, 40, 134.
*References 59, 60, 63, 68, 73, 79, 157–161.
*References 2, 5, 19, 85, 130, 133, 285–296.
†References 2, 85, 133, 290, 291, 296–298, 300.
*References 73, 157, 160, 169, 179, 180.
*References 2, 59–61, 73, 77–79, 160–164, 169, 177–180, 331–333, 356.