Bone Marrow Transplantation
At the conclusion of this chapter, the reader should be able to:
• Identify and discuss various types of cancer treated with progenitor cell transplants.
• Define the term progenitor cell.
• Name three types of stem cell transplants.
• Discuss available treatment options for cancer.
• Discuss the evaluation of candidates for transplantation.
• Describe the process of obtaining blood stem cells.
• Discuss the transplantation protocol, related complications, graft manipulation and storage, and cell infusion.
• Compare at least three current directions in bone marrow transplantation.
• Identify and discuss directions in bone marrow transplantation.
• Analyze laboratory and clinical data of the cited case study and apply these concepts to the field of bone marrow transplantation.
• Correctly answer case study related multiple choice questions.
• Be prepared to participate in a discussion of critical thinking questions.
Cancers Treated with Progenitor Cell Transplants
Leukemia
In most types of leukemia, the body produces large numbers of immature white blood cells (WBCs) that do not function properly. Under appropriate conditions, bone marrow transplantation may be useful in treating certain types of leukemia (Box 32-1).
Non-Hodgkin’s and Hodgkin’s Lymphoma
For patients with lymphoma, chances of survival depend on the grade and stage of cancer, overall patient health, and response to treatment. Hodgkin’s lymphoma is one of the most curable forms of cancer. Patients diagnosed with stage I disease have more than a 90% chance of living 10 years or longer. Of interest, higher grade aggressive types are more likely to be cured with chemotherapy. Lower-grade lymphoma often can have longer average survival times, with a mean survival of 10 years in some cases. Most children respond well to treatment, even though children tend to have the higher grades of lymphoma. From 70% to 90% of these children survive 5 years or longer (Table 32-1).
Table 32-1
Estimated 5-Year Survival Rates after Transplantation∗
Disease | Allogeneic (%) | Autologous (%) |
Severe combined immunodeficiency | 90 | N/A |
Aplastic anemia | 90 | N/A |
Thalassemia | 90 | N/A |
Acute myeloid leukemia | ||
First remission | 55-60 | 50 |
Second remission | 40 | 30 |
Acute lymphocytic leukemia | ||
First remission | 50 | 40 |
Second remission | 40 | 30 |
Chronic myeloid leukemia | ||
Chronic phase | 70 | ID |
Blast crisis | 15 | ID |
Chronic lymphocytic leukemia | 50 | ID |
Myelodysplasia | 45 | ID |
Multiple myeloma | 30 | 35 |
Non-Hodgkin’s lymphoma, first relapse, second remission | 40 | 40 |
Hodgkin’s disease, first relapse, second remission | 40 | 50 |
ID, Insufficient data; N/A, not applicable.
∗These estimates are based on data reported by the International Bone Marrow Transplant Registry.
Traditional Treatment Options
Chemotherapy
Chemotherapeutic drugs can be divided into the following:
1. Agents that are active against both dividing and nondividing cells
2. Drugs that are active against dividing cells and affect a particular phase of cell division
3. Agents that affect all or most of the phases of the cell cycle (Box 32-2)
Evaluation of Candidates for Peripheral Blood Stem Cell and Bone Marrow Transplantation
Pretransplantation evaluation and testing (Fig. 32-1) may include HLA tissue typing, bone marrow biopsy and aspiration, electrocardiography, echocardiography, complete history and physical examination, chest x-ray study, pulmonary function tests, dental cleaning, blood tests such as complete blood count (CBC) and blood chemistries, and screening for viruses such as hepatitis, human T lymphotropic virus I and II, cytomegalovirus (CMV), herpes, and human immunodeficiency virus (HIV).
ABO Blood Group and Human Leukocyte Antigen Matching
HLA matching is important because a close HLA match does the following:
• Improves the chances for a successful transplantation
• Promotes engraftment, the process of donated cells beginning to grow and produce new blood cells in the host
• Reduces the risk of post-transplantation graft-versus-host disease (GVHD)
Minimum matching levels must be met before a donor or unit of cord blood cells can be transplanted. The National Marrow Donor Program (NMDP) program requires that at least a 6 out of 8 match exist. However, some transplant centers set more stringent requirements for a 7 out of 8 match between patient and donor (Fig. 32-2).
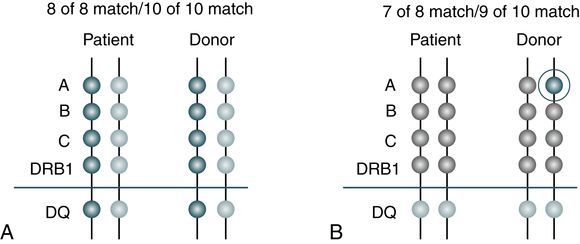
A, All the patient’s markers match the donor’s. The 8 of 8 match means that there is a match at A, B, C, and DRB1. A 10 of 10 match means that there is a match at A, B, DRB1, C and DQ. B, One of the patient’s A markers does not match one of the donor’s A markers. Therefore, this is a 7 of 8 match or a 9 of 10 match. (©National Marrow Donor Program, 2012, www.marrow.org/patient. Reprinted with permission.)
Obtaining Cells for Transplantation
Bone Marrow
In the procedure for harvesting bone marrow, the donor is given general or regional anesthesia and marrow is usually aspirated with large needles from the posterior iliac crest; the anterior crest can also be used in certain cases (Fig. 32-3). The goal of the procedure is to collect 10 to 15 mL of marrow/kg of recipient weight. Approximately 600 to 900 mL of marrow is collected. The aspirated marrow is collected in bags containing a buffered isotonic solution and heparin to prevent coagulation.
Transplantation
Graft Manipulation and Storage
Automated procedures involving apheresis equipment; such as the COBE Spectra (Terumo BCT, Lakewood, Colo) and Fenwall (Americus, Lake Zurich, IL) use a closed sterile system that rapidly recovers the desired mononuclear cells (Fig. 32-4).Minor ABO mismatches are present in 15% to 20% of HLA-matched donor-recipient pairs. Patients who receive hematopoietic progenitor cells from a minor ABO-incompatible donor are at risk of developing immediate immune hemolysis caused by isohemagglutinins infused with the marrow or PBSCs, or delayed hemolysis caused by isohemagglutinins produced by the donor lymphocytes (i.e., B cells). Immediate hemolysis can be avoided by simple removal of plasma from the graft before infusion. However, delayed hemolysis caused by antibody production from donor-derived B lymphocytes requires the ex vivo removal of lymphocytes or suppression of T lymphocyte function by cyclosporine.
Chapter Highlights
• The goal of transplanting bone marrow or peripheral blood progenitor cells is to achieve a potential cure or help patients recover from high-dose chemotherapy that has destroyed healthy stem cells or marrow cells.
• Bone marrow and peripheral blood progenitor cells are capable of reconstituting a patient’s immune system because they contain the precursor to the cells that make up the blood. Some stem cells circulate in the bloodstream and are called peripheral blood stem cells (PBSCs).
• There are three major types of transplants—allogeneic, syngeneic, and autologous.
• Chemotherapy and radiation target rapidly dividing cells. These therapies are used to treat cancers because cancer cells divide more rapidly than healthy cells. Bone marrow cells also divide at a rapid rate and can be severely damaged or destroyed by high-dose treatment.
• Without healthy bone marrow, a patient cannot make the blood cells that are needed to fight off infections, carry oxygen, and prevent bleeding. Bone marrow and PBSC transplants can replace the normal and abnormal cells that were destroyed during treatment.
• Factors that influence the eligibility for bone marrow transplantation include age, disease status, performance status for the recipient, organ function, infectious disease status, compatibility of the donor and recipient, and psychosocial status.
• The procedure for obtaining or harvesting bone marrow is the same for all types of transplants. The goal of the harvest procedure is to collect 10 to 15 mL of bone marrow/kg recipient weight.
• Complications that develop from transplantation of bone marrow or PBSCs range from infection, GVHD, rejection, and organ damage to infertility and death.