Body Positioning
Specific indications for body positioning and the decision-making process are highlighted. The chapter does not provide treatment prescriptions for given conditions because no specific patient is being considered. Understanding the physiological effects of body position on oxygen transport and how pathophysiology disrupts these normal processes is fundamental to prescribing body positioning for a given patient. An optimal body position can be prescribed only on the basis of consideration of all factors that impact on oxygen transport: the effects of the patient’s pathophysiology and its specific presentation in that individual, the effect of restricted mobility and recumbency, the effect of extrinsic factors related to the patient’s care, and the effect of intrinsic factors related to the patient (Chapter 17). It is only with an integrated analysis of these factors overall that (1) the most beneficial body positions can be predicted; (2) the least beneficial body positions can be identified and used minimally; and (3) the appropriate outcome measures can be selected.
Gravity and Normal Physiological Function: Physical Therapy Implications
The human is an orthograde organism. From moment to moment, gravity exerts its influence on the human body and particularly affects oxygen transport. The combined effects of gravity on the lungs, heart, and peripheral circulation are central to their interdependent function and to establishing normal oxygen transport. Knowledge of the effects of gravity on cardiovascular and pulmonary function in health and the deleterious effects of pathophysiological states on cardiovascular and pulmonary function (both primary pathology of the cardiovascular and pulmonary system and pathology of this system secondary to pathology in one or more other systems, as described in Chapter 6) provides a foundation for therapeutic body positioning as a primary intervention to optimize oxygen transport. Because of its potent and direct effect on oxygen transport, therapeutic body positioning can maintain or augment arterial oxygenation so that invasive, mechanical, and pharmacological forms of respiratory support can be postponed, reduced, or avoided—a primary objective of cardiovascular and pulmonary physical therapy.
In health, normal oxygen transport is maintained by being upright and moving—the physiological body position. A patient who may have prolonged periods of recumbency is also exposed to gravity continuously, but not to the same extent as in the upright position. Each body position the patient assumes differentially affects the steps of the oxygen transport pathway as a result of alterations in gravitational stress. Oxygen transport can be improved, maintained, or worsened with changes in body position. Despite being essential to normal cardiovascular and pulmonary function, gravity is the principal contributor to inhomogeneity of physiological function down the lungs.1 Figure 20-1 illustrates the effect of this gradient with respect to alveolar ventilation (), perfusion (Q), ventilation and perfusion ratio (
), PaO2, PCO2, PN2, oxygen content, carbon dioxide (CO2) content, pH, and the flow of oxygen and CO2 in and out of the lungs. Thus the lungs should not be likened to balloons, either physiologically or anatomically.
Based on a detailed analysis of all factors contributing to impaired oxygen transport and gas exchange (Chapter 17), the body positions that will have an optimal effect on oxygenation and those that may be deleterious must be distinguished from one another. In this way, a greater proportion of time can be spent by the patient in beneficial positions and less time in deleterious positions. Body positions themselves are associated with energetic stress, particularly in positions with greater gravitational stress, so the upright position can be more energetically demanding than supine positions, which are more demanding than lateral positions.2 Compression forces also affect the heart and cardiac output. Lying on the left side, for example, can compromise cardiac output, particularly within 12 hours after surgery in patients whose cardiac index is less than 2.3 L/min/m2.3
The length of time a body position is maintained is response-dependent rather than time-dependent,4 and it reflects individual differences, including pathology, severity, age, and body mass. Knowledge of the deleterious effects of prolonged periods in a single position supports the prescription of both frequent body position changes and extreme sequential body positions. These perturbations simulate the normal perturbations that the cardiovascular and pulmonary systems experience in health during normal mobility and body position changes in daily life. The ability to weigh the relative beneficial and deleterious effects of each possible body position (through 360 degrees in the horizontal plane and 180 degrees in the vertical plane, ranging from approximately 20 degrees head down to 20 degrees leaning forward) on a given patient’s gas exchange is critical in prescribing body positioning.
Prescriptive Versus Routine Body Positioning
The literature supports the benefits of frequent body position changes, particularly for a patient who is relatively immobile, not alert, severely debilitated, obtunded, breathing at low lung volumes, obese, aged or very young, or has lost the sigh mechanism. Even though the effectiveness of routinely turning patients every 2 hours has not been firmly established, it continues to be an accepted standard of care (although intensive care units fall short of this minimal guideline).5 The practice is based on the belief that the deleterious consequences associated with assuming a static position for a prolonged period will be prevented. More frequent turning, however, may have greater physiological benefits in patients who are critically ill, and this suggests that patients who are less severely ill may also benefit from being gravitationally challenged systematically. The preventive effects of a routine turning regimen are distinct from the acute effects of body positioning on oxygen transport, which is the primary focus of this chapter.
Physiological Effects of Various Body Positions
Body positioning has potent and direct effects on most steps of the oxygen transport pathway, so it can be prescribed to elicit these effects preferentially. Because humans function optimally when upright and moving, therapeutic interventions that elicit or simulate being upright and moving (i.e., elicit both gravitational and exercise stress) are most justified physiologically (see Chapter 17). The recumbent supine position, a common position assumed by patients who are hospitalized, is nonphysiological and is deleterious to oxygen transport. The side-lying positions have an effect that is intermediate between that of upright and supine. The prone position, which is underutilized clinically, can have such a significant powerful effect on oxygen transport that a good rationale must be made for not incorporating this position into the treatment prescription.
The indications for therapeutic body positioning and for frequent body position changes to optimize oxygen transport are shown in Tables 20-1 and 20-2. For each of the indications listed, an optimal therapeutic body position can be selected for a given patient. A description of the physiological effects of several primary body positions follow, namely, the upright/supine, side-lying, head-down, and prone positions. However, this information cannot be applied out of context. The specific positions prescribed for a given patient are based on consideration of the multiple factors that impair oxygen transport (Chapter 17) in conjunction with a physiological analysis of the most justifiable positions.
Table 20-1
Indications for Body Positioning to Optimize Oxygen Transport
Suboptimal preload and afterload
Impaired systolic ejection fraction to pulmonary and systemic circulations
Untoward gravitational, mechanical, and compression forces on the myocardium, great vessels, mediastinal structures, and lymphatic system
Suboptimal fluid shift from central to dependent areas (extremities) and vice versa to maintain fluid volume regulating mechanisms
Table 20-2
Indications for Frequent Changing of Body Position
Because of the potent and direct effects of body positioning on the steps in the oxygen transport pathway in health and in disease, it is unknown whether the beneficial effects reported with the use of postural drainage are attributable to enhanced mucociliary transport; or to the direct effect of positioning the good lung down on improving the gas exchange of that lung by increasing alveolar volume of the affected, nondependent lung; or to both of these mechanisms. Typically, studies evaluating conventional chest physical therapy, including postural drainage, have failed to control for the direct effect of body positioning on oxygen transport or for the direct effects of increased arousal and mobilization that occur when a patient’s body position is changed.6,7 This is a serious methodological problem that pervades the literature evaluating conventional practices, so-called chest physical therapy, and one that has to be considered when interpreting the results of studies of these procedures. Unless these potent confounding variables are controlled, the degree to which conventional chest physical therapy has a beneficial effect over and above the effects of positioning, as well as of mobilization and increased arousal, cannot be established.8
Upright Positions
Although the upright position is common to the physiological and anatomical positions, movement in the upright constitutes the true physiological position in that the upright position coupled with movement (e.g., walking, cycling, or movement in sitting) is consistent with the requirements of daily activities. To meet the energetic demands of these activities, oxygen transport is optimized to the greatest degree, in that ventilation and perfusion are more uniform than they would be without the additional exercise stimulus. The upright standing position maximizes lung volumes and capacities, with the exception of closing volume, which is decreased.9 Functional residual capacity (FRC), the volume of air remaining in the lungs at the end of end-tidal expiration, is greater in standing than in sitting and exceeds that in the supine position by as much as 50% (Figure 20-2). Maximizing FRC is associated with reduced airway closure and maximal arterial oxygenation.10,11 Figure 20-3 illustrates the relationship of FRC and closing capacity as a function of age. Because of age-related pulmonary changes, the closing capacity of the dependent airways increases with age; this effect is further accentuated with recumbency. Airway closure is evident in the supine position in the healthy 45-year-old person and in the upright seated position in the healthy 65-year-old person.12 Compression atelectasis results from cardiac weight, abdominal pressure, and pleural effusions, the effects of which are determined by the specific position of the patient.13 These positional effects are further accentuated in patient populations with cardiovascular and pulmonary, thoracic, and abdominal pathologies, so the upright position is favored, and the supine position should be minimized so as to prevent airway closure and impaired gas exchange.
With respect to pulmonary function testing, the upright sitting position with legs dependent is the standard reference position.14,15 When upright, the diameter of the main airways increases slightly. If the airways are obstructed, even small degrees of airway narrowing induced by recumbency can increase airway resistance (Figure 20-4). When a person is upright, the vertical gravitational gradient is maximal, the anteroposterior dimension of the chest wall is the greatest, and compression of the heart and lungs is minimized.16 The shortened position of the diaphragmatic fibers is countered by an increase in the neural drive to breathe when a person is in the upright position.17 Maximal expiratory pressure is augmented with progressively upright positions in patients with chronic obstructive lung disease, as well as in those in health; standing results in the highest values and head-down positions the lowest.18 Thus coughing and other forced expiratory maneuvers should be encouraged when the individual is in the optimal erect, upright position.
The distribution of ventilation is determined primarily by the effect of gravity, which changes down the lung because of the anatomical position and the suspension of the lungs within the chest. At FRC in the upright position, the intrapleural pressure at the apex is −10 cm H2O and at the base −2.5 cm H2O (Figure 20-5). The intrapleural pressure is less negative in the base because of the suspended mass of the lungs. As a result of the greater negative intrapleural pressure in the apices, thus low compliance, these lung units have a larger initial volume; hence smaller volume changes occur during respiration. Because the lung units at the base have a smaller initial volume, thus high compliance, larger volume changes occur during respiration. Therefore, depending on their relative position with respect to gravity, different regions of the lung are at different points on the pressure volume curve.
A common clinical concern is patients breathing at low lung volumes—for example, patients in pain, patients who have undergone thoracic or abdominal incisions, older and younger patients, obese patients, pregnant patients, patients with gastrointestinal dysfunction (such as paralytic ileus and ascites, organomegaly, or intrathoracic or intraabdominal masses) patients who are malnourished, and patients who are mechanically ventilated or have spinal cord injuries). Breathing at low lung volumes reverses the normal intrapleural pressure gradient such that in the upright lung the apices have a negative intrapleural pressure compared with the bases, which have a positive intrapleural pressure (i.e., exceeding airway pressure) (Figure 20-6). This results in the apices being more compliant, thus better ventilated, than the bases. The bases are prone to airway closure in individuals breathing at low lung volumes.
Another factor that reverses the normal intrapleural pressure gradient is mechanical ventilation. Despite its necessity in the management of patients in respiratory failure, mechanical ventilation can contribute to hypoxemia in several ways. First, it reverses the normal intrapleural pressure gradient so that the uppermost lung fields are preferentially ventilated. Because the dependent lung fields are preferentially perfused, mismatch is promoted. Positive pressure ventilation has the additional complication of increasing intrathoracic pressure and reducing venous return and cardiac output. These factors, in addition to the negative pressure required to open the inspiratory valve, can increase the work of breathing associated with mechanical ventilation.19–21
Although gravity is the primary determinant of interregional differences in the distribution of ventilation in the healthy lung, intraregional differences, secondary to differences in compliance and resistance of contiguous lung units, also contribute.22 These effects are exaggerated in patient populations.23
The distribution of perfusion down the upright lung is also primarily dependent on gravity (Figure 20-7). The pressures affecting blood flow through the pulmonary capillaries and resulting in the typical uneven distribution (inhomogeneity) of blood flow are alveolar pressure and the arterial and venous pressures. In zone 1, at the apex, alveolar pressure predominates over arterial and venous pressures, so there is little or no blood flow. Zone 2, in the middle zone, reflects the blood flow from the recruitment of pulmonary vessels. Arterial pressure exceeds alveolar pressure and blood flow. Zone 3, in the lower area of the lung, reflects the blood flow from the distension of pulmonary vessels; arterial and venous pressures exceed alveolar pressure. And zone 4 (not illustrated), in the most dependent portion of the lung, has little or no pulmonary blood flow because of the interstitial pressure acting on the pulmonary blood vessels and creating a compression force.1
The matching of and Q reflects the interfacing of the distributions of
and Q down the upright lung. Both
and Q increase down the upright lung; however,
increases disproportionately more than Q (Figure 20-8). As a result, the optimal area for
matching is in the midzone, where the ratio is about 1.0.24
The upright position is associated with marked hemodynamic effects. These effects reflect primarily the central blood volume, which is shifted from the thoracic compartment to the dependent venous compartments when a person assumes the upright position from the supine.25–27 End-diastolic volume and stroke volume are decreased, which results in a compensatory increase in heart rate.28 Cardiac output is correspondingly decreased. The net effect of these physiological changes is a reduction in myocardial work.29 This finding is corroborated by the observation that the anginal threshold is increased in patients with cardiac conditions when they are upright.30 Further, intermittent gravitational stress following myocardial infarction or bypass surgery maintains orthostatic tolerance and thereby prevents bed rest deconditioning.31
Peripheral vascular resistance increases and blood flow decreases with the assumption of an upright position greater than 45 degrees to offset dependent fluid shifts and potential blood pressure drop (Figure 20-9). An upright angle of at least 60 degrees is needed to optimize cardiac output and sympathetic tone.32 Another important effect of body position on fluid volume is the promotion of urinary drainage from the renal pelvi to the bladder when in the upright position, as a result of the reduced area for urinary stasis when in this position as opposed to the supine position (Figure 20-10). Optimal renal function is essential to preserving normal hemodynamic status.
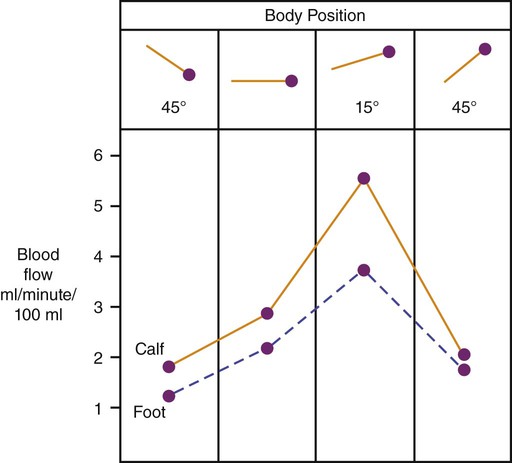
Older individuals who are relatively immobile tend to sit for prolonged periods. Without frequent exposure to standing upright, however, the phenomenon of seated postural hypotension may result.33 In addition, dependent circulatory stasis and other consequences of restricted mobility such as deconditioning are promoted in this position.
Supine Position
The indiscriminate use and overwhelming acceptance of bed rest evolved historically over the past 150 years. In the mid-1800s, the use of immobilization to heal bones and injuries was extended to the management of other conditions in the belief that internal organs could be rested as a therapeutic intervention as well. The injudicious application and overuse of bed rest to address medical problems has been challenged over several decades.34–38 Although there has been a marked decrease in the use of prolonged periods of bed rest based on innumerable studies over the past several decades of its negative sequelae,39 its use is still quite common despite the fact that its merits as a therapeutic modality and the parameters for its prescription (i.e., indications and specifications to achieving healing and recovery without deterioration) have not been scientifically established.
The supine position inherent in bed rest alters the configuration of the chest wall, the anteroposterior position of the hemidiaphragms, the intrathoracic pressure, the intraabdominal pressure secondary to the shifting of the abdominal viscera in this position, and the mechanics of cardiac function.40–45 The normal anteroposterior configuration becomes more transverse. The hemidiaphragms are displaced cephalad, which reduces FRC in this position.10 Prefaut and Engel46 observed that hypoxic vasoconstriction secondary to closure of the dependent airways in the supine position contributed to preferential perfusion of the nondependent lung zones. Finally, in the supine position, excess pulmonary secretions tend to pool on the dependent sides of the airways. The upper side may dry out, exposing the patient to infection and obstruction (see Figure 20-4).
An increase in intrathoracic blood volume in the supine position also contributes to a reduction in FRC and lung compliance and to increased airway resistance.47,48 Collectively, these effects predispose the patient to airway closure and an increase in the work of breathing. Although a healthy person can accommodate to these physiological changes, a healthy person does not assume this position for prolonged periods without unconsciously shifting. A hospitalized patient, however, is less likely to adapt to these immediate changes and their long-term effects. They may be less responsive to the need to shift position or unable to respond to afferent stimuli prompting a need to change position. These effects are accentuated in older people whose arterial oxygen tensions progressively diminish with age.47,49 Compared with younger people, the arterial oxygen tensions of older people are particularly lower in the supine position compared with the reference sitting position.50
Several cardiovascular changes occur when the supine position is assumed (e.g., hemodynamic or intolerance to position change). A central shift of blood volume from the extremities to the central circulation initiates orthostatism.27,51 This fluid shift increases both the preload and the afterload of the right side of the heart. This increased volume tends to distort the interventricular septum and reduce left ventricular volume and preload. The relatively increased central blood volume inhibits the release of antidiuretic hormone and atrial natriuretic peptide from the right side of the heart52 and diuresis is stimulated.53 Between 10% and 15% of fluid is lost within 24 hours,27 which can manifest clinically as cardiac underfilling, orthostatic intolerance, and negative fluid balance.54 Altered autonomic nervous system function also has been implicated in the etiology of bed rest deconditioning.55,56 Individuals with impaired autonomic control (those with high spinal cord lesions) exhibit greater reductions in mean arterial pressure and stroke volume compared with able-bodied individuals.57 Because of local vascular regulation, cerebral circulation can be maintained. Finally, when individuals are confined to bed rest abruptly, they show more dehydration than do individuals confined to bed rest for a longer term, and it occurs faster, possibly because of less opportunity to compensate.58 Although the prescriptive parameters regarding the optimal gravitational stress required to offset the hemodynamic changes that occur with the supine position have yet to be elucidated (degree of upright angle, duration, and frequency),59 there are other effects of the upright position that support its frequent use in patients whose mobility is restricted. Anatomical regions adapt to the relative microgravity effects of the supine position differently according to the redistribution of transmural pressures and flows within the various vascular beds.60 Knowledge of these physiological adaptations is essential for refining therapeutic countermeasures.
Although lower body negative pressure (LBNP) may have a role in space exploration for maintaining orthostatic stability and has been shown to offset change with bed rest such that exercise capacity is maintained,61 LBNP will not likely be a feasible or practical modality in acute management. Despite its reported benefit in countering the adverse fluid shifts associated with recumbency, LBNP does not address other negative effects of bed rest.
Because of a reduction in the vertical gravitational gradient, and therefore in the intrapleural pressure gradient of the lung, in the supine position, the distribution of matching appears more uniform and evenly matched in horizontal positions.62,63 These changes, however, must be considered in conjunction with other changes associated with the supine position: namely, reduced FRC, reduced vital capacity, reduced flow rates, increased area of dependent lung, and increased closure of the dependent airways, as well as its associated hemodynamic consequences. These deleterious factors offset any theoretical benefit of the supine position on V/Q matching.64 However, in a patient who is unresponsive, hemodynamically unstable, and clinging to life, a recumbent position may be the only alternative (see end-of-life issues in Chapter 33).
The position of the diaphragm and its function are dependent on body position. Figure 20-11 illustrates the effect of body position on the level and movement of the diaphragm consistent with compromised lung function recorded in recumbent positions. In the supine position, the resting level of the diaphragm is influenced differentially by anesthesia and neuromuscular blockade.65 Figure 20-12 illustrates these effects. In the spontaneously breathing subject, the excursion of the diaphragm is greater posteriorly because of the dependent viscera beneath the posterior portion of the diaphragm. During anesthesia with or without paralysis, the diaphragm ascends 2 cm into the chest. When paralysis is induced, the loss of diaphragmatic tone results in greater excursion of the nondependent rather than the dependent part of the diaphragm.
A study of hemodynamic postural effects in healthy subjects supports that the horizontal position maximizes central blood volume.66 This position, however, cannot be considered clinically optimal because of such effects as intrathoracic and intraabdominal compression.
Side-Lying Positions
Side-lying may be theoretically less deleterious compared with the supine position.22,45,67 The side-lying position accentuates anteroposterior expansion at the expense of transverse excursion of the dependent chest wall. In this position, the dependent hemidiaphragm is displaced cephalad because of the compression of the viscera beneath it. This results in a greater excursion during respiration and to greater contribution to the ventilation of that lung and to gas exchange as a whole. The FRC in side-lying falls between that in the upright and supine positions. Compared with supine, in side-lying compliance is increased, resistance is reduced, and the work of breathing is reduced, whereas these measures are reversed when side-lying is compared with the upright position. Figure 20-13 illustrates the difference in lung volumes between maximal inspiration and FRC in a spontaneously breathing subject in right side-lying. Although ventilation is enhanced to the dependent lung, inspiratory lung volume and FRC are reduced. Compared with the reference sitting position, FEV1 and FVC are reduced similarly in left and right side-lying, with no differential effects of side-lying on diffusing capacity and closing volume.68 These lung-function effects in side-lying may reflect altered lung geometry with position change and a reduction in the vertical diameter of each lung in side-lying compared with that which occurs in the supine position.69
Side-lying increases end-diastolic ventricular pressure on the dependent side secondary to compression of the viscera beneath the diaphragm and to reduced lung compliance on that side.70 Although such changes can be readily accommodated in health, they may further impair gas exchange in individuals with oxygen transport deficits.
Optimal matching occurs in the upper one-third of each lung in the side-lying position.71 The total area for optimal
matching, therefore, is likely greater than that in the upright position, which contributes theoretically to an improved
matching. These apparent improvements, however, are offset by reduced lung volumes and airflow rates in this position.
In healthy people and in patients, arterial oxygen tension is greater in side-lying than in supine position.72 This is true for patients receiving supplemental oxygen, as well as for those who are not. Thus side-lying can be used to enhance the efficiency of gas exchange and thereby minimize or avoid the use of supplemental oxygen. Arterial blood gases have been reported to be improved in patients with unilateral lung disease73,74 when they are positioned with the good lung down and worsened when the affected lung is down. When lung pathology is bilateral, arterial blood gas values are better when patients lie on the right side than when they lie on the left. This can be explained by the greater size of the right lung and the reduced compression of the heart on the lung in this position compared with left side-lying.75,76 The practice of “down with the good lung,” however, has been questioned in patients with unilateral lung collapse secondary to central airway lesions.77 Not all patients respond favorably when the good lung is down. Research is necessary to distinguish the characteristics of responders and nonresponders to refine the prescription of body positions.
The side-lying rather than the supine position is often a preferred position for patients who are hospitalized; yet its physiological consequences are not well understood. The pulmonary effects of side-lying have been reported for healthy older people.68 FEV1 and FVC are reduced comparably for right and left side-lying compared with a reference sitting position. Although diffusing capacity and homogeneity of ventilation appear to be unchanged, they may be adversely affected in patient populations with single and combined pathologies that affect oxygen transport.
Patients who are hemodynamically unstable require special consideration with respect to body positioning. These patients may be less able to benefit from active mobilization and are more reliant on positional perturbation than on movement to promote optimal gas exchange. In one study of patients with severe respiratory failure and receiving inotropic support, the extreme left lateral position contributed to a hyperdynamic state, and the right lateral position predisposed the patients to hypotension, likely as a result of impaired right ventricular preload.78 Spirometric evidence from patients who were intubated supports decreased dynamic lung compliance in the lateral and prone positions compared with supine.79 Body positioning warrants judicious prescription, with particular attention to the angle of the lateral position and the duration and to monitoring to observe the effect.
Prolonged side-lying has been shown to mobilize lung water in patients with pulmonary edema, and to a lesser extent in patients with pulmonary inflammation.80 Physical therapy interventions that entail gravitational challenges, therefore, can have a direct effect on lung water distribution and possibly compartmentalization, which in turn, may affect pulmonary compliance and gas exchange. Similarly, pleural effusions respond to the effect of gravity. In patients with unilateral effusions, when the effusion is positioned lowermost in side-lying, oxygenation is lower than when the effusion is positioned uppermost.81 This may be explained by the effusion impairing matching and gas exchange in the lowermost lung.
Head-Down Position
The head-down position augments oxygen transport in some patients by improving pulmonary mechanics. Patients with chronic airflow limitation, for example, tend to have hyperinflated chests and flattened diaphragms in which the contraction of the diaphragm is inefficient because of the position of its muscle fibers on the length tension curve. The head-down position causes the viscera to be displaced cephalad beneath the diaphragm. The diaphragm is positioned more normally and rests at a higher and more mechanically efficient position in the chest.82,83 In this position, patients may experience relief from dyspnea, reduced accessory muscle use, reduced upper chest breathing patterns, and reduced minute ventilation. Patients with pathology in the lung bases may also benefit from the head-down position in that this position favors gas exchange in the more functional upper lung fields and promotes alveolar distension of bases, which are uppermost in the head-down position. Other patients, however, such as those with respiratory muscle fatigue, may have increased respiratory distress in this position due to the added resistive loading of the diaphragm because of the weight of the viscera beneath. These observations support the need to prescribe body positioning on an individual basis with consideration of the multiple factors that may impair oxygenation as well as enhance it.
Prone Position
There is mounting physiological and scientific justification for use of the prone position to enhance arterial oxygenation and reduce the work of breathing in patients with cardiovascular and pulmonary dysfunction who may or may not be mechanically ventilated. The prone position shifts the mobile structures of the thoracic and abdominal cavities.84,85 The heart and great vessels are displaced anteriorly. The liver, spleen, and kidney shift anteriorly and caudally.
The prone position increases arterial oxygen tension, tidal volume, and dynamic lung compliance.86–88 The pleural pressure gradient is homogenized; hence the distribution of and alveolar inflation are augmented. Ventilation to perfusion matching has been shown to be more uniform in horizontal positions,62 reflecting more uniform pleural pressure gradient and less lung compression by the heart.89 In addition, the prone position reduces stroke volume, increases sympathetic activity, and augments urine output.90 There has been increasing interest in exploiting these benefits in patients who are critically ill where mobilizing options are more limited (see Chapters 34 and 35). The prone position has some role in avoiding mechanical ventilation in patients who are conscious and alert, thereby reducing the risk for ventilator-associated complications.88 Over the years, studies of prone positioning have been conducted largely in patients with acute respiratory distress syndrome (see Chapter 36).91–93 Prone is associated with improving oxygenation in 70% to 80% of cases.94 The effect of prolonged duration in the prone position has been studied, and the benefits appear to be dose-dependent.95 The physiological outcomes of prone positioning in the management of acute lung injury are thought to reflect the particular pathoetiology.
Complications associated with prolonged periods in the prone position, particularly skin breakdown, are common; thus close monitoring of skin over bony prominences is essential. To prevent or manage these complications, intermittent prone positioning has been advocated. Intermittent prone positioning of patients with acute respiratory distress syndrome and best positive end-expiratory pressure (PEEP) therapy can reduce FIO2 levels to less than 0.50, the critical level for oxygen toxicity.96
In a study using a canine model of acute lung injury, the prone position was observed to augment oxygenation in conjunction with low PEEP and to offset the negative hemodynamic effects of high PEEP.97 This finding is consistent with clinical outcome studies. The two common variants of the prone position are prone abdomen-restricted and prone abdomen-free. Prone abdomen-restricted refers to lying prone with the abdomen in contact with the bed, whereas in the prone abdomen-free position, the patient’s hips and chest are elevated so that the abdomen is free. Both prone positions augment gas exchange, but the prone abdomen-free position, comparable to the hands-and-knees position,98 enhances lung compliance, tidal volume, FRC, and diaphragmatic excursion to a greater degree and minimizes compression of the heart and abdominal viscera on the lungs.13 In one study, use of supplemental oxygen was reduced in four of five patients who were mechanically ventilated and whose positioning regimen included the prone abdomen-free position.99
Physiological Effects of Frequent Changes in Body Position
Table 20-2 lists some of the physiological effects of frequent changes in body position that are mediated primarily through their effects on respiratory mechanics, cardiac mechanics, airway closure, mucociliary transport, lymphatic drainage, and altered neural activation of the diaphragm. These effects, resulting from a change in position, are distinct from the benefits derived from a particular static body position. The benefits of changing position can be enhanced by moving to an extreme position (i.e., moving from supine to prone, rather than moving from supine to side-lying). Extreme body position changes simulate, but do not replace, the physiological “stir-up” and perturbations that occur with normal mobility and being upright. When the stir-up regimen was originally proposed, Dripps and Waters100 did not appreciate fully the physiological implications of regular upright standing.101 The net effect of changing body position is a stirring-up of the constituent distributions of , Q, and
matching and shifting of internal organs and their compression on the structures of the cardiovascular and pulmonary unit. Areas of dependent atelectasis, physiological dead space and shunting, and mucus distribution are dramatically shifted.102 The stir-up stimulates lymphatic drainage, surfactant production and distribution through stretching of the lung parenchyma, and the distribution and function of pulmonary immune factors.103 Frequent physical perturbations also may inhibit bacterial colonization.104 In addition, frequent body position changes shift and redistribute compression forces acting on the diaphragm, myocardium, and mediastinal structures and the compression of the lungs by the myocardium and mediastinal structures.
Frequent body position changes are used by physical therapists to stimulate the patient and increase arousal to a more alert and wakeful state (Figure 20-14). The more upright the patient, the greater the neurological arousal and the greater the stimulus to breathe; this effect is augmented by encouraging the patient to be self-supporting. Commensurate with an increase in arousal, the patient is stimulated to take deeper breaths and, hence, to increase . When body positioning is coupled with mobilization, vasodilation and recruitment of the pulmonary capillaries are stimulated, and this, in turn, improves the homogeneity of the distributions of
and Q, hence augmenting
matching.
Prescription of Therapeutic Body Positions and Body Position Changes
Prescription of body positioning is based on an analysis of the factors that contribute to impaired oxygen transport for each patient.105 Specific positions are selected to simulate as closely as possible the physiological function of the normal, healthy, upright cardiovascular and pulmonary unit and oxygen transport, and the perturbations and stirring-up that occurs hemodynamically during normal mobility and being upright.101 A hierarchy of body positioning alternatives based on the physiological justification of the various positions appears in Table 20-3. These positions range from the most to the least physiological. The hierarchy is based on the premise that oxygen transport is optimal when upright and moving. Mobilization in the upright position increases tidal volume and respiratory rate, hence increasing minute ventilation, flow rates, and mucociliary transport and clearance and enhances the efficiency and effectiveness of airway clearance and coughing. Thus incorporating active movement into the body position change is optimal.
Table 20-3
Physiological Hierarchy of Body Positions
The upright erect sitting position implies that the back, head, and neck are vertical and aligned, with flexion only at the hips; the patient is not slumped or recumbent.
The less able the patient is to assist in positioning, the greater the need for more extreme positions and greater turning frequency.
If the patient is totally unable to move (i.e., in a coma or paralyzed), extreme body positions are indicated if they are not contraindicated because of hemodynamic instability or increased intracerebral pressure. The upright position is used as much as possible, provided the patient is physically supported for safety and monitored in terms of treatment response. Passive standing on a tilt table is hemodynamically questionable; it is preferable that patients be placed in a high Fowler position, with legs positioned dependently, using the knee breaks in the bed.
Regimens of 360-degree horizontal turning and 180-degree vertical turning (ranging from 20 degrees head-down to 20 degrees lean-forward) are used unless contraindicated. Positioning through a maximal arc simulates as closely as possible the three-dimensional movement of the chest wall during normal respiration.
Body position should first be exploited when coupled with movement followed by the erect sitting positions with legs dependent. Figure 20-15 illustrates several variants of the sitting position. Each of these positions has distinct effects on oxygen transport, so the specific upright position has to be prescribed specifically, because the supported and propped-up positions in bed do not substitute for upright, erect sitting. These variants have a role only when the patient deteriorates while being positioned upright or when in that position. Sitting with the feet down and supported is preferable to the propped up long sit because of the gravitationally induced hemodynamic benefits associated with this position. Sitting up in bed fails to position the patient in a perfectly vertical upright position. The head-up position in bed can be maximized, and the use of knee breaks in the bed provides a gravitational stimulus to the circulating blood volume.
A common practice is to progressively turn patients in one-quarter turns, such as from supine to side-lying and back to supine and so forth. However, the use of extreme positions and extreme position changes may yield greater physiological benefit with respect to the degree of perturbation and stir-up elicited.106 Extreme position changes result in significant alterations in the distributions of , Q, and
matching. Mucociliary transport is stimulated, and secretion accumulation and stagnation is minimized. In addition, the more extreme the body position, the greater the degree of arousal stimulated, particularly in the upright positions, and arousal is essential in patients who are critically ill.
Mechanical Body Positioning
Kinetic or mechanically turning beds have long been known to benefit oxygen transport in patients who are severely ill.107–111 These beds are indicated for those patients who are moderately hemodynamically unstable and on neuromuscular blockers and thus tolerate manual turns poorly. Such turning frames are contraindicated, however, for patients who are less ill. Even for patients who require multiple assistants and extra caution and time to turn and position effectively, these issues should never deny a patient a much-needed treatment with proven effectiveness.
Practical Considerations in Positioning Patients
Prescriptive body positioning can require a significant amount of the physical therapist’s time, as well as that of other team members. Such prescriptive positioning is based on clear indications and well-defined parameters; it is not to be confused with “routine” positioning. For any hospitalized patient who is recumbent and inactive, body positioning is a 24-hour concern, both during and between treatments. Such patients are at risk for impaired oxygen transport. The team as a whole has a responsibility to ensure that complications related to static positioning and restricted mobility are prevented.112
Despite the time and labor involved in turning a patient to the prone position, particularly if the patient is mechanically ventilated, the benefits of this position (even for short periods) outweigh the time and effort required. Given the benefits that can be derived from the prone position, justification has to be made for not doing so. Frequently, therapeutic body positioning can be effectively coordinated with nursing interventions and other procedures. With all body positions, but especially with the prone position in patients who are severely ill, potential inclusion and exclusion criteria should be reviewed, along with preturn considerations, the turning technique, assistants, monitoring the effectiveness of the prone position, passive movements, and limb positioning.91 Finally, any problems or adverse responses to the prone, as to other positions, are documented.
Monitoring Responses to a Body Position or Position Changes
The duration and frequency of the positions and the frequency of body position changes are response-dependent rather than time-dependent. Monitoring is the basis for defining and modifying the body positioning prescription. The physiological variables to be monitored depend on the patient’s specific presentation and on cardiovascular and pulmonary dysfunction and its severity and distribution. Monitoring the patient who is not critically ill includes subjective and objective evaluation of indexes of the adequacy of oxygen transport (Part II). Among the most important are oxygen delivery, oxygen consumption, oxygen extraction, and gas exchange indicators such as the A/AO2 difference and the PAO2/PaO2. Subjectively, the patient’s facial expression, respiratory distress, dyspnea, anxiety, peripheral edema, discomfort, and pain are assessed. Objectively, heart rate, blood pressure, respiratory rate, SaO2, flow rates, and bedside spirometry can be readily assessed. The appropriate standardization and procedures need to be used to ensure that the measures are both valid and reliable (see Chapter 7). Because physiological variables change from moment to moment, serial measures over a period of time should be taken to establish an average value, rather than using peak or discrete measures, which may misrepresent a treatment effect.
To interpret and compare various measures, the FIO2 and any change in FIO2 must be recorded. The use of the ratio PaO2:FIO2 enables comparison of gas exchange within and between patients when FIO2 levels differ or change.113 Similarly, for patients who are mechanically ventilated, any changes in the ventilatory parameters must be noted, in addition to other interventions that have a direct effect on oxygen transport. Only in this way will the clinician be able to reasonably conclude that the position or position change was instrumental in enhancing oxygen transport.