W2 Bedside Ultrasonography
Use of Bedside Ultrasonography in the Intensive Care Unit
General Indications
Ultrasonography has become an invaluable tool in the management of critically ill patients. Its safety and portability allow for use at the bedside to provide rapid, detailed information regarding the cardiovascular system1 and the function and anatomy of certain internal organs. It also can be used by the clinician to assess the pleural and intraabdominal spaces and to perform some invasive procedures safely. General indications for performance of echocardiography in the ICU are listed in Box W2-1. Box W2-2 lists major indications for performance of primary TEE in the ICU. Other indications for use of bedside ultrasonography by the intensivist in critically ill patients are listed in Box W2-3.
Box W2-1
General Indications for Performance of an Echocardiographic Examination in the Intensive Care Unit
Box W2-2
Major Indications for Performance of Primary Transesophageal Echocardiography Study in the Intensive Care Unit
PEEP, positive end-expiratory pressure; TTE, transthoracic echocardiography.
Technical Aspects
Acoustic Window in a Critically Ill Patient
The practical value of bedside ultrasonography in the management of critically ill patients is now widely accepted despite the inherent limitations of the technique.2 These limitations are related mostly to suboptimal imaging conditions that commonly are encountered when performing studies of critically ill patients. The constrained physical environment of the ICU also can compromise the quality of the images obtained. For an ultrasound study to be deemed adequate, a good acoustic “window” is required to allow accurate analysis. Ultrasonography uses the physical principle that sound is reflected from tissue interfaces, allowing a two-dimensional (2D) image of the anatomic structure studied to be constructed.3 Anything hindering the reflection of this acoustic signal—air, bone, calcium, a foreign body, or another interposed structure—interferes with ultrasound transmission and diminishes the overall quality of the examination. In the ICU, many patients are mechanically ventilated. In these patients, adequate imaging can be limited by pneumothorax, pneumomediastinum, or subcutaneous emphysema.2 Other important factors limiting data acquisition in critically ill patients are related to surgical wounds and dressings, tapes, tubing, obesity, and chronic obstructive pulmonary disease. In addition, lack of patient cooperation and the impossibility of moving some patients into the optimal position for the examination contribute to a high prevalence of technically inadequate studies.2
Although ultrasonography permits evaluation of the structure and function of the heart and other important organs and structures, acquisition of data and interpretation of results are fraught with potential traps.4 Performing an ultrasound examination requires a thorough knowledge of anatomy and instrumentation, including attention to gain control, grayscale settings, Doppler velocity settings, and transducer placement.
Preparation of the Patient
An awake patient should be informed about the importance of the ultrasound investigation and should be provided with an explanation of how the clinician will perform the examination.3 These steps are especially important when the examination uses the transesophageal route.
Sedation
To optimize the ultrasound examination, the patient must be cooperative and nonagitated. Noninvasive procedures such as TTE and abdominal ultrasound usually are well tolerated by patients, and additional sedation rarely is needed to perform these procedures. When performing TEE, however, certain precautions need to be taken. Patients should fast (or have their tube feeds stopped) for at least 4 hours before the procedure. Topical anesthesia of the oropharynx also is helpful before insertion of the TEE probe, especially in patients who are not endotracheally intubated.3 Even if adequate topical anesthesia is provided, insertion of the TEE probe still can cause significant discomfort and anxiety, so providing adequate sedation and analgesia is important. Frequently used sedative or analgesic agents include intravenous (IV) midazolam, fentanyl, and propofol. Dosing should be titrated according to clinical parameters including arterial blood pressure, minute ventilation, and arterial oxygen saturation.3 Sedative-induced hypotension is a frequent problem in patients with depressed ventricular function or decreased systemic vascular resistance, and occasionally patients may require transient support with IV volume infusion or rarely a vasopressor agent. If the patient is extremely uncooperative and biting, transient paralysis accompanied by increased sedation may have to be used to perform TEE safely.
Monitoring During the Procedure
Most ICU patients are monitored continuously, at least for certain respiratory, cardiac, or hemodynamic parameters. It is essential that patients undergoing an ultrasound examination in the ICU be monitored at least with noninvasive recording of blood pressure, pulse oximetry, and electrocardiogram. Even TTE or abdominal ultrasound examinations can be associated with inadvertent pulling of tubes or drains, and anxiety can be encountered during the procedure. Because of its more invasive nature, TEE may induce complications such as increased agitation, respiratory distress, and discomfort during insertion of the probe. These effects can be associated with substantial changes in blood pressure and ventilatory status. Administration of sedatives and sometimes paralytic agents can induce further changes in hemodynamic and respiratory status.3,5
Safety
Performance of ultrasound examinations in the ICU allows procedures that previously required transport to the radiology suite to be performed at the bedside. This is an important advantage to a critically ill patient, because transport out of and back to the ICU is known to be associated with increased risk of complications.6 Performance of bedside TTE and of other noninvasive ultrasound examinations is safe and not associated with significant risks to the patient. Performance of bedside TEE also is associated with a low incidence of serious complications (<0.5% in the general population and the elderly).5 The reported mortality rate associated with TEE is 0.01% to 0.03%.7 Most patients undergoing TEE examinations in the ICU usually are receiving mechanical ventilation and have continuous monitoring of arterial blood pressure, electrocardiogram, and oxygen saturation.8 Transient hypotension, typically attributable to administration of sedative medications, usually can be treated with vasopressors or IV fluids or both. The risk of injury to the pharynx or esophagus is greater in anesthetized and endotracheally intubated critically ill patients than in awake patients, because anesthetized patients cannot assist with probe insertion by swallowing and do not resist when insertion is difficult.8 Increased difficulty in directing the TEE probe also can be encountered owing to the presence of a nasogastric tube. Coagulopathy and thrombocytopenia, common problems in critically ill patients, can increase the risk of hemorrhage due to mucosal injury during blind insertion of the TEE probe. Daniel et al.9 reported significant complications related to TEE in 18 (0.18%) of 10,218 examinations. In 11 studies reporting on 943 patients undergoing TEE, the rate of complications was 1.7%.5 Serious complications occurred in only two patients (0.2%). Colreavy et al.8 studied the safety and utility of TEE performed by ICU physicians in 255 critically ill patients and showed that TEE was associated with a complication rate of only 1.6%. It is reasonable to conclude that TEE is associated with few complications, given the high severity of illness among ICU patients.5 Close monitoring of hemodynamic and oxygenation parameters is essential. Box W2-4 lists specific contraindications to the insertion of a TEE probe.
Bedside Echocardiography in a Critically Ill Patient
Echocardiography can provide diagnostic information noninvasively regarding cardiac structure and mechanical function. The supplementary information provided by this technique can help determine the cause of hypotension refractory to inotropic support or vasopressor infusions.3 It also can help in the diagnosis of a wide spectrum of other cardiovascular abnormalities and guide therapeutic management. An adequate understanding of the proper use of echocardiography is a prerequisite for the intensivist. General indications for performance of an echocardiographic examination in the ICU are listed in Box W2-1.
Transthoracic Versus Transesophageal Echocardiography in a Critically Ill Patient
Accurate and prompt diagnosis is crucial in the ICU. The easiest and least invasive way to image cardiac structures is TTE.3 This noninvasive imaging modality is of great value in the critical care setting because of its portability, widespread availability, and rapid diagnostic capability. In the ICU, TTE in certain cases may fail to provide adequate image quality because of different factors that potentially can hinder the quality of the ultrasound signal, as was described previously. The failure rate (partial or complete) of TTE in the ICU has been reported to be 30% to 40%.10,11 Improvements have been made in transthoracic imaging (e.g., harmonics and contrast and digital technologies), however, resulting in a lower failure rate of TTE in the ICU (10%-15% in our institution).
TEE is particularly useful for evaluation of suspected aortic dissection, prosthetic heart valves (especially in the mitral position), source of cardiac emboli, valvular vegetations, possible intracardiac shunts, and unexplained hypotension. TEE allows better visualization of the heart in general and especially the posterior structures, owing to the proximity of the probe and favorable acoustic transmission.1 TTE also has limitations, however. For several areas of the heart and great vessels, TEE may provide limited images. The view of the left ventricular apex often is foreshortened with TEE, and an apical left ventricular clot can be missed. TTE usually is superior for visualization of the apex. Because of interposition of the left mainstem bronchus, the superior portion of the ascending aorta is another important area that may not be well visualized with TEE. With TEE, transducer position and angulation are constrained by the relative positions of the esophagus and heart. The relatively fixed relationship between the position of the probe and the heart often makes it impossible to align the Doppler beam parallel to the flow of interest (e.g., to evaluate the jet of blood resulting from aortic stenosis). In addition, the 2D image planes of TEE often make standard anatomic measurements more difficult to obtain.
As a result of the significantly improved technical quality of TTE, most ICU patients can be studied satisfactorily with this modality. Immediate TEE is still preferable, however, in certain specific clinical situations in which TTE is likely to fail or be suboptimal.11 The major indications for primary TEE in the ICU12,13 are listed in Box W2-2. Even when TEE is necessary, data from the TTE examination are often essential for the final clinical interpretation.
Hemodynamic Evaluation
Ventricular Function
Left Ventricular Systolic Function
The simplest quantitative approach is to measure the mid-left ventricular short-axis dimension at end diastole and end systole for determination of the percent fractional shortening. Fractional shortening is related directly to EF; normal fractional shortening is 30% to 42%.1
In the setting of regional wall motion abnormalities, fractional shortening may underestimate or overestimate global ventricular function and must be interpreted in light of what is seen in all of the 2D imaging planes of the ventricle.14
Global systolic ventricular function also can be assessed quantitatively by fractional area change (normal value is 36% to 64%)15 and EF (normal value is 55% to 75%) (Figure W2-1):
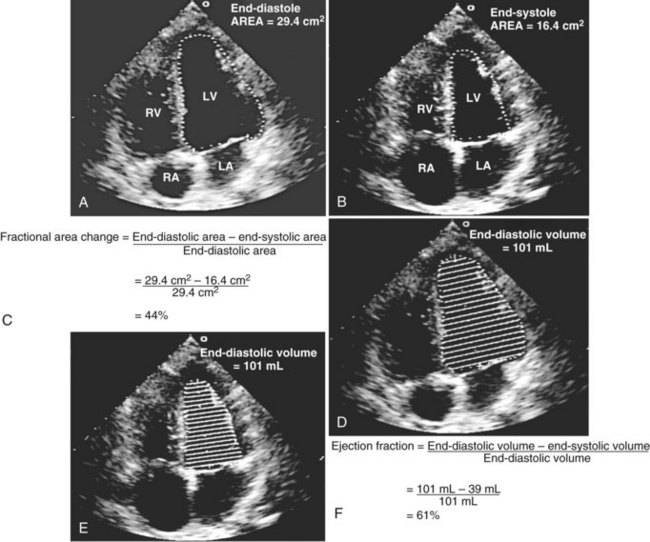
Figure W2-1 Fractional area change and ejection fraction calculation. Endocardial contour of the left ventricular cavity is traced at end diastole (A) and at end systole (B) in the transthoracic apical four-chamber view. Machine-integrated software computes the data and gives corresponding end-diastolic and end-systolic areas. Fractional area change can be calculated with these data (C). Normal values are 36% to 64%.15 Corresponding end-diastolic (D) and end-systolic (E) volumes are computed using the modified Simpson’s method. The data are used to calculate the ejection fraction (F). Normal values are 55% to 75%. LA, left atrium; LV, left ventricle; RA, right atrium; RV, right ventricle.
These measurements require good image quality, because endocardial border contours must be traced (see Figure W2-1). Machine-integrated software computes the data and provides volumes, areas, and the resultant EF (see Figure W2-1). In patients with regional wall motion abnormalities, more precise measures of stroke volume can be made by approximating ventricular volumes as a stack of elliptical discs on biplane imaging (modified Simpson’s method).1,15
In the critical care setting, endocardial border definition may be suboptimal because of poor image quality.10,16,17 In these cases, global ventricular function often is assessed qualitatively by visual inspection alone. This method has been found to be reliable when used by experienced clinicians.18 By simple visualization of the kinetics and size of the cardiac cavities in real time, an experienced intensivist with a sufficient echocardiographic background can establish a functional diagnosis immediately.
Analysis of regional wall motion includes a numerical scoring system to describe the movement of the different regions of the left and right ventricle (1 = normokinesia; 2 = hypokinesia; 3 = akinesia; 4 = dyskinesia; 5 = aneurysmal change).15 Visualized from the short-axis view of the left ventricle, a complete overview of myocardial areas perfused by the three major coronary arteries can be obtained (Figure W2-2). If the TTE examination is technically difficult and the endocardium is poorly visualized, harmonic imaging and possibly contrast, if needed, can dramatically improve endocardial border visualization and subsequent evaluation of global systolic function (as discussed further later in this chapter). For the remaining few technically challenging cases with suboptimal TTE, performance of TEE allows for a more precise evaluation of ventricular function in most critically ill patients because of the higher image quality that can be obtained with this echographic modality.
Left Ventricular Failure in the Intensive Care Unit
In a critically ill patient with unexplained hemodynamic instability, determination of cardiac function is an integral part of the medical management. Echocardiography is valuable in this setting because the clinical examination and invasive hemodynamic monitoring often fail to provide an adequate assessment of ventricular function. In a study by Fontes et al.19 that compared pulmonary artery (Swan-Ganz) catheterization and TEE, the overall predictive probability for conventional clinical and hemodynamic assessment of normal ventricular function was 98%, whereas for abnormal ventricular function (EF <40%), it was 0%. Several other studies have reported similar results.20–22 Assessment of biventricular function is one of the most important indications for performance of echocardiography in the ICU. In a study by Bruch et al.,23 115 critically ill patients were studied by TEE. The most common indication for TEE was hemodynamic instability (67% of patients). Of these hemodynamically unstable patients, 20 (26%) were found to have significant left ventricular dysfunction (EF <30%). In a study by McLean24 of the use of TEE in the ICU, the most common reason to request a TEE was assessment of left ventricular function. In most patients, left ventricular function was assessed adequately by TTE before TEE. In a study by Vignon et al.,17 TTE allowed adequate evaluation of global left ventricular function in 77% of mechanically ventilated ICU patients. Although TEE was needed for most other indications, TTE was shown to be an excellent diagnostic tool for assessment of left ventricular function in the ICU (Figure W2-3) even when positive end-expiratory pressure is present.
Sepsis-Related Cardiomyopathy
Classically, septic shock has been considered a “hyperdynamic” state characterized by normal or high cardiac output. Echocardiographic studies indicate that ventricular performance often is markedly impaired in patients with sepsis.25–27 Parker et al.28 were the first to describe left ventricular hypokinesis in septic shock. They reported that survivors manifested severely depressed left ventricular EF, but that adequate left ventricular stroke output was maintained as a result of acute left ventricular dilation.29 Jardin et al.25 studied 90 patients with septic shock and performed daily bedside assessments of left ventricular volume and left ventricular EF using TTE. They observed that left ventricular EF was significantly depressed in all patients, resulting in severe reductions in left ventricular stroke volume. Of these patients, 34 (38%) eventually were weaned from hemodynamic support and showed gradual improvement in left ventricular EF and ultimately recovered. The remaining 56 patients (62%) eventually died (of early circulatory failure or late multiple organ failure). In this subset, the degree of left ventricular dysfunction was less than in survivors but failed to improve over time. The severity of left ventricular dysfunction does not predict outcome. A paradoxical relationship between the degree of left ventricular dysfunction and the likelihood of recovery also has been described by others.25,28,30,31 Among patients who survive, left ventricular dilation and systolic dysfunction usually are reversible.
Left ventricular EF might not be a reliable index of left ventricular systolic function in patients with early septic shock because this is a state characterized by low systemic vascular resistance that unloads the left ventricle.25 Normal or supranormal EF in early sepsis might lead clinicians to make the wrong inference about cardiac reserve because left ventricular EF might decrease if afterload is increased by the administration of vasopressor agents.
Left Ventricular Diastolic Function
In the ICU, diastolic dysfunction should be suspected when ventricular filling pressure (pulmonary capillary wedge pressure) is elevated and EF is normal or supranormal.1 The diastolic properties of the ventricle often are assessed by evaluating Doppler echocardiographic mitral inflow and pulmonary venous flow patterns. Mitral inflow, as measured by pulsed wave Doppler at the tips of the mitral leaflets, is characterized by an early filling phase (E wave) followed by atrial systole, resulting in additional filling (A wave) (Figure W2-4). The transmitral Doppler pattern always should be interpreted in conjunction with pulsed wave Doppler of the pulmonary venous flow, which is characterized by a systolic phase (S), a diastolic phase (D), and an atrial phase (AR) from reversal of flow into the pulmonary veins during atrial contraction (Figure W2-5). These filling patterns are related to the intrinsic diastolic properties of the myocardium and are influenced by many different factors, particularly left atrial pressure, heart rate, ischemia, ventricular hypertrophy, and valvular pathologies. Only modest correlation has been found between Doppler indices of diastolic function and parameters measured using more invasive means.32,33 Integrated interpretation of mitral and pulmonary venous flow patterns may be useful for diagnosing abnormal myocardial relaxation (e.g., owing to hypertensive heart disease, hypertrophic cardiomyopathy, or coronary ischemia) or restrictive pathology (e.g., owing to cardiomyopathy, constrictive pericarditis, coronary artery disease, cardiac transplantation, or dilated cardiomyopathy). Nevertheless, these findings must be interpreted with caution when caring for critically ill patients, given the many different factors that can acutely influence flow patterns in this population of patients.
Right Ventricular Function and Ventricular Interaction
Abnormal right ventricular function often plays an important and sometimes underestimated role in the pathogenesis of critical illness.34–36 Based on an echocardiographic definition,37 massive pulmonary embolism and acute respiratory distress syndrome are the two main causes of acute cor pulmonale in adults.38 In the critical care setting, right ventricular function also can be altered by any other perturbations that increase right ventricular afterload, such as positive end-expiratory pressure or increased pulmonary vascular resistance (from vascular, cardiac, metabolic, or pulmonary causes). Depressed right ventricular systolic function is also often associated with right ventricular infarction, most commonly in the setting of inferior myocardial infarction. Acute sickle-cell crisis, air or fat embolism, myocardial contusion, and sepsis are other causes of acute right ventricular dysfunction.
Adequate assessment of right ventricular function is important when caring for hemodynamically unstable, critically ill patients, specifically patients with massive pulmonary embolism and acute respiratory distress syndrome, because the diagnosis of concomitant significant right ventricular dysfunction may alter therapy (e.g., fluid loading, use of vasopressors, use of thrombolytics) and provide information about prognosis.38,39 Echocardiographic examination of the right ventricle requires primarily an assessment of the size and kinetics of the cavity and septum.37,40 Normally the right ventricle appears relatively flat. As it dilates, the apical region of the right ventricle becomes more rounded (Figure W2-6). In the short-axis view, the right ventricle, which usually has a crescentic shape, becomes oval because of septal displacement and bulging of the right ventricular free wall (see Figure W2-6).1 Right ventricular size and function generally are evaluated by visual comparison with the left ventricle. Right ventricular diastolic dimensions can be obtained by measuring right ventricular end-diastolic area in the long axis, from an apical four-chamber view, using either TTE or TEE.
Because pericardial constraint necessarily results in left ventricular restriction when the right ventricle acutely dilates (i.e., there is ventricular interaction), one of the best ways to quantify right ventricular dilation is to measure the ratio between the right ventricular and left ventricular end-diastolic areas, an approach that cancels out individual variations in cardiac size.37,40 Moderate right ventricular dilation corresponds to a diastolic ventricular ratio greater than 0.6; severe right ventricular dilation corresponds to a ratio greater than or equal to 1.37,40 Right ventricular diastolic enlargement usually is associated with right atrial dilation, inferior vena caval dilation, and tricuspid regurgitation. When pressure in the right atrium exceeds pressure in the left atrium, the foramen ovale may open. Pressure and volume overload of the right ventricle can lead to distortion of left ventricular geometry and abnormal motion of the interventricular septum. With conditions of high strain imposed on the right ventricle (volume or pressure overload or both), the interventricular septum flattens, and the left ventricle appears to have a “D” shape (see Figure W2-6).4,37 This “paradoxical” septal motion also is seen at the interatrial level.
Because the two ventricles are enclosed within the relatively stiff pericardium, the sum of the diastolic ventricular dimensions has to remain constant.41 Acute right ventricular or left ventricular dilation can occur only if it is associated with an acute and proportional reduction in left ventricular or right ventricular diastolic dimension (i.e., ventricular interaction). With acute right ventricular dilation, septal displacement impairs left ventricular relaxation; the opposite occurs with acute left ventricular dilation. In these situations, the pressure-volume relationships of the left and right heart chambers are altered, and information obtained from a pulmonary artery catheter could be misleading (e.g., high filling pressures are recorded despite normal or even low circulating volume).
Pulmonary Embolism
Hemodynamic instability from acute cor pulmonale as a consequence of massive pulmonary embolism is a relatively common occurrence in critically ill patients. Until more recently, contrast pulmonary angiography generally was regarded as the gold standard for the diagnosis of pulmonary embolism. Angiography is an invasive procedure, however, and carries the risk of major complications in patients with circulatory failure.42 Contrast-enhanced helical computed tomography (CT) is an accurate and noninvasive test that has replaced angiography for the diagnosis of pulmonary embolism. Even CT requires transportation of patients to a location outside of the ICU, however, and transport alone is associated with significant risks. Echocardiography is well suited for diagnosis of pulmonary embolism because it can be done within minutes at the bedside. The diagnosis of acute cor pulmonale at the bedside with TTE has good positive predictive value for massive pulmonary embolism.43,44 This technique can detect acute right ventricular dilation and dysfunction resulting from a large pulmonary embolism. The finding of right ventricular dilation and dysfunction is not specific for pulmonary embolism, however, because these findings may be observed with a variety of other conditions associated with increased right ventricular strain. In a study by McConnell et al.,45 patients with acute pulmonary embolism were found to have a distinct regional pattern of right ventricular dysfunction with akinesia of the mid–free wall but normal motion at the apex by TTE. These findings contrasted with findings obtained in patients with primary pulmonary hypertension who had abnormal wall motion in all regions. Regional right ventricular dysfunction had a sensitivity of 77% and a specificity of 94% for the diagnosis of acute pulmonary embolism; positive predictive value was 71%, and negative predictive value was 96%. The presence of regional right ventricular dysfunction that spares the apex should raise the level of clinical suspicion for the diagnosis of acute pulmonary embolism.
Central pulmonary emboli are present in half of patients with symptoms of pulmonary embolism and acute cor pulmonale on TTE.5 Emboli lodged in the proximal pulmonary arteries usually cannot be visualized using TTE.5 Because other clinical conditions can produce acute cor pulmonale in the ICU, better visualization of the pulmonary arteries is needed to achieve high accuracy for the diagnosis of pulmonary embolism. This goal can be achieved by using TEE. TEE has good sensitivity for detecting emboli lodged in the main and right pulmonary arteries but is limited for the detection of more distal or left pulmonary emboli.5,46,47 If an embolus is visualized, the diagnosis is made. If the study is negative when the index of suspicion for pulmonary embolism is high, however, TEE must be followed up by a more definitive test such as angiography or helical CT. Also, when there is high clinical suspicion for pulmonary embolism but no emboli are visualized using TEE, the potential for nonthrombotic causes of pulmonary embolism (e.g., air or fat emboli) must be kept in mind.
The demonstration of acute cor pulmonale with echocardiography has important prognostic and therapeutic implications.48,49 The presence of cor pulmonale with massive pulmonary embolism is associated with increased mortality, whereas the absence of right ventricular dysfunction is associated with a better prognosis.39 There is no consensus on the precise indications for administration of thrombolytics in massive pulmonary embolism complicated by acute cor pulmonale.50,51 A safe and reasonable strategy for managing critically ill patients with suspected massive pulmonary embolism is as follows:
Assessment of Cardiac Output
Measurement of cardiac output remains a cornerstone in the hemodynamic assessment of critically ill patients. Thermodilution is considered the gold standard approach for determining cardiac output in most ICUs. Measurement of cardiac output using thermodilution requires placement of a pulmonary artery catheter (or at least central venous and arterial catheters); although a useful technique, it is invasive and potentially inaccurate. Unreliable values are particularly common in the presence of tricuspid regurgitation related to high pulmonary artery pressure. Several methods for determining cardiac output have been described using 2D and Doppler echocardiography. With this technique, stroke volume and cardiac output can be determined directly by combining Doppler-derived measurements of instantaneous blood flow velocity through a conduit with the cross-sectional area of the conduit. Blood flow can be calculated through various cardiac structures, including the pulmonary valve,52 mitral valve,53,54 and aortic valve.55–58 In the absence of intracardiac shunts, blood flow through these structures should be the same (continuity equation).59 Of these methods, the one using the left ventricular outflow tract and aortic valve as the conduit is probably the most reliable and most commonly used. There is excellent agreement with thermodilution in most situations.55–58 The left ventricular stroke volume is obtained by measuring the cross-sectional area of the left ventricular outflow tract (area [cm2] = (left ventricular outflow tract diameter [cm2]) × (π/4), assuming that just below the aortic annulus, the left ventricular outflow tract is circular) multiplied by the transaortic flow velocity time integral derived from a spectral Doppler tracing. The stroke volume obtained is multiplied by the heart rate to give the cardiac output: cardiac output = cross-sectional area × velocity time integral × heart rate (Figure W2-7).
With TTE, the left ventricular outflow tract diameter usually is obtained from the parasternal long-axis view, just below the insertion of the aortic valve leaflets. The Doppler interrogation is performed through the aortic valve from the apical view (see Figure W2-7). With TEE, the left ventricular outflow tract diameter usually is obtained from the five-chamber view of the left ventricle. The transgastric view usually is used to obtain an apical long-axis view of the aortic valve through which Doppler interrogation is performed.60 With either TTE or TEE, obtaining an accurate left ventricular outflow tract diameter and Doppler signal is essential to have an accurate cardiac output calculation. Because the measure of the left ventricular outflow tract diameter has a second-order relationship with the cross-sectional area (see previous formula), it is crucial that this measure be determined precisely. For the Doppler signal to be reliable, the Doppler sample must be parallel to the transaortic flow with an angle of incidence not exceeding 20 degrees to avoid underestimation of transaortic velocity. Using TTE, McLean and coworkers61 showed an excellent correlation (r = 0.94) between cardiac output determined by the left ventricular outflow tract Doppler method and the thermodilution method in critically ill patients. Other studies have shown similar results.55 In a study by Feinberg et al.,58 cardiac output determined by TEE Doppler imaging was obtainable in 88% of 33 critically ill patients, and there was good correlation (r = 0.91) with the thermodilution method. Descorps-Declere et al.60 also showed transgastric pulsed Doppler measurement across the left ventricular outflow tract with TEE to be a clinically acceptable method for cardiac output measurement in critically ill patients (r = 0.975 compared with the thermodilution method).
Another promising ultrasound-based technology to estimate cardiac output noninvasively in adults uses a small transesophageal Doppler probe to measure blood flow velocity waveforms in the descending aorta combined with a nomogram (based on height, weight, and age) for estimation of aortic cross-sectional area. This minimally invasive esophageal probe can be inserted easily in sedated patients and left in place safely for several days to provide continuous monitoring of cardiac function.62,63 Several technical problems can limit the accuracy of cardiac output measurements by esophageal Doppler monitoring,62 however, and although initial results are promising,64–66 more studies are needed to make a decision regarding the accuracy of this technique in critically ill patients.
Assessment of Filling Pressures and Volume Status
Adequate determination of preload and volume status is important for proper management of critically ill patients. Invasive pressure measurements to assess left ventricular filling are commonly used at the bedside to make inferences regarding left ventricular preload. These pressure measurements correlate only weakly with left ventricular volume, however.67 Data from invasive monitoring using pulmonary artery catheterization may be misleading because ventricular compliance is altered secondary to numerous factors.68,69 Differences in diastolic compliance among patients may account for the weak correlation between pressure and volume and may limit the ability to use pressure measurements alone to derive information concerning left ventricular preload.14 Echocardiography can be helpful for adequately assessing preload. Parameters that can be measured using 2D imaging are left ventricular end-diastolic volume and left ventricular end-diastolic area. Using Doppler interrogation, additional information—mainly transmitral diastolic filling pattern and pulmonary venous flow—can be obtained.
Two-Dimensional Imaging
Echocardiography has been validated for left ventricular volume measurements.15 Subjective assessment of left ventricular volume by estimating the size of the left ventricular cavity in the short-axis and long-axis views is often adequate to guide fluid volume therapy at the extreme ends of cardiac filling and function. More precise quantitative values are desirable, however, and can be obtained by using endocardial border tracing (as described earlier). The normal left ventricular end-diastolic volume as determined by echocardiography is 80 to 130 mL,15 and the normal left ventricular end-diastolic volume index is 55 to 65 mL/m2.15 Left ventricular end-diastolic area measured in the left parasternal short-axis view at the level of the midpapillary muscle is commonly used to estimate volume status (Figure W2-8). The normal values for left ventricular end-diastolic area in the short-axis view are 9.5 to 22 cm2.15
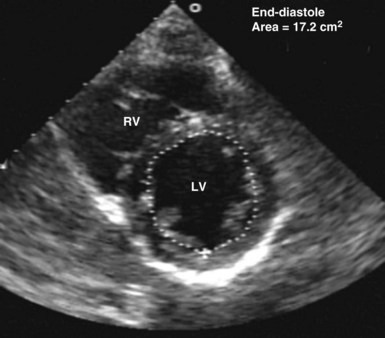
Figure W2-8 Calculation of left ventricular end-diastolic area in the transthoracic short-axis view at the level of the midpapillary muscle by endocardial contour tracing. Values of normal left ventricular end-diastolic area in the short axis range from 9.5 to 22 cm2.15 The level of the midpapillary muscle is used because of the reproducibility of the view and because changes in left ventricular volume affect the short axis of the ventricle to a greater degree than the long axis. LV, left ventricle; RV, right ventricle.
Two-dimensional TTE evaluation of ventricular dimensions has been found to be useful in assessing preload and optimizing therapy of ICU patients.25,70 Nevertheless, image quality may be suboptimal and preclude adequate visualization of the endocardial border by TTE. This potential limitation of TTE has been partly circumvented in recent years with the advent of harmonic imaging and contrast echocardiography (see later). In cases in which endocardial border visualization remains suboptimal, TEE is the modality of choice. With TEE, left ventricular volume can be estimated rapidly by subjective assessment of the left ventricular size. Quantitatively, it is estimated most often by determining left ventricular cross-sectional area at the end of diastole, most commonly using the transgastric short-axis view at the level of the midpapillary muscle. This section is used because of the reproducibility of the view and because changes in left ventricular volume affect the short axis of the ventricle to a greater degree than the long axis.14 The end-diastolic area must be measured consistently from the same reference section. End-diastolic area measured with TEE correlates with left ventricular volume determined by radionuclide studies.70
Systolic obliteration of left ventricular cross-sectional area accompanies decreased end-diastolic area and is considered to be a sign of severe hypovolemia (Figure W2-9). Although a small end-diastolic area generally indicates hypovolemia, a large end-diastolic area does not indicate adequate preload in patients with left ventricular dysfunction. Also, when systemic vascular resistance is low, as in early sepsis, left ventricular emptying is improved because of the lowered afterload. In these situations, it may be difficult to differentiate hypovolemia from low systemic vascular resistance by echocardiography alone, because both conditions are associated with decreased end-diastolic area.
Knowledge of left ventricular end-diastolic volume or absolute preload does not allow for accurate prediction of the hemodynamic response to alterations in preload.71 Tousignant et al.72 investigated the relationship between left ventricular stroke volume and left ventricular end-diastolic area in a cohort of ICU patients and found only a modest correlation (r = 0.60) between single-point estimates of left ventricular end-diastolic area and responses to fluid loading. Based on the assumption that changes in end-diastolic area occur because of changes in left ventricular volume, the determination of this area and its subsequent degree of variation after a fluid challenge could help better assess preload responsiveness. Studies have shown that changes in end-diastolic area measured by TEE using endocardial border tracing are closely related to changes in cardiac output and are superior to measurements of pulmonary artery occlusion pressure for predicting the ventricular preload associated with maximal cardiac output.73
Circulating volume status also can be assessed by 2D echocardiography by indirectly estimating right atrial pressure; this is often done by assessing the diameter and change in caliber with inspiration of the inferior vena cava (Figure W2-10). This method has been shown to discriminate reliably between right atrial pressures less than 10 mm Hg or greater than 10 mm Hg.74 A dilated vena cava (diameter >20 mm) without a normal inspiratory decrease in caliber (>50% with gentle sniffing) usually indicates elevated right atrial pressure. In mechanically ventilated patients, this measure is less specific because of a high prevalence of inferior vena cava dilation.75,76 A small vena cava reliably excludes the presence of elevated right atrial pressure in these patients.75,76
Doppler Flow Patterns
Information obtained by analysis of the Doppler signal at the level of the mitral valve and pulmonary vein offers additional information about preload.77,78 These Doppler profiles can be obtained by either TTE or TEE. Transmitral parameters that have been studied include the relation of early to late transmitral diastolic filling (E/A ratio), isovolumetric relaxation time, and the rate of deceleration of early diastolic inflow (deceleration time).1
A decrease in preload causes a significant reduction in the E wave (early filling flow wave) velocity at the mitral level in conjunction with a decrease of the S wave (systolic flow wave) in the pulmonary vein. In clinical practice, the E/A ratio is easy to assess; the normal value of this ratio is approximately 1.1,3 In conjunction with normal left ventricular contractility, a low E/A ratio is usually a characteristic sign of inadequate preload.79
Pulmonary venous flow also can be used to assess left atrial pressure. A normal pulmonary venous flow pattern showing a predominance of flow during systole (S phase) compared with early diastole (D phase) usually indicates that left atrial pressure is less than 8 mm Hg, whereas the opposite predominance of flow (in the absence of significant mitral regurgitation) usually indicates elevation of left atrial pressure.1
Hypovolemia in the Intensive Care Unit
Precise and rapid assessment of volume status is crucial when caring for hemodynamically unstable ICU patients. Hypovolemia is one of the most common causes of hypotension in the ICU. As was discussed in detail earlier, bedside echocardiography offers a quick and reliable way of estimating volume status by evaluating cardiac dynamics and left ventricular dimensions and area. The finding of end-systolic cavity obliteration is usually a reliable sign of hypovolemia. Other changes in the volume status are usually associated with subtle changes in left ventricular cavity size, so only this extreme is reliable to make the diagnosis of hypovolemia by echocardiography. In general, TTE has good sensitivity for diagnosing the presence of a small hyperdynamic left ventricle, the most typical finding in hypovolemic patients with underlying normal cardiac function, although TEE is useful in the immediate postoperative setting (Video W2-1).
When dynamic left ventricular obstruction is present, cardiac output is low, and even in the presence of marked hypovolemia, pulmonary artery occlusion pressure is high. Paradoxical worsening of hypotension after intravascular volume loading may be the first clue to dynamic left ventricular obstruction in critically ill patients. It is important that this entity be recognized early and that the pathophysiologic process be well understood, because inadequate management of this condition can lead rapidly to worsening of hemodynamic status and death. Dynamic obstruction of the left ventricle can present in different forms. One of these forms is dynamic left ventricular outflow tract obstruction. Although dynamic left ventricular outflow tract obstruction is often seen in association with asymmetrical septal hypertrophy, it also can occur in other situations.80,81 Dynamic left ventricular outflow tract obstruction is thought to be caused by the Venturi effect. This effect results when excessive acceleration of blood through a conduit produces a decrease in pressure. In the left ventricular outflow tract, such a decrease in pressure leads to a suction phenomenon that draws the anterior mitral leaflet and chordae inward toward the interventricular septum.82 This systolic anterior motion of the mitral valve leads to contact between the mitral leaflet and the septum that creates an obstructive subaortic pressure gradient and distortion of the mitral valve leaflet coaptation (Figure W2-11).82 By 2D echocardiography, the left ventricle appears to be small and hyperdynamic, and there is motion of the anterior leaflet (or chordae or both) toward the septum in systole (see Figure W2-11). With color Doppler, a “mosaic” pattern of flow is seen in the left ventricular outflow tract, owing to the high velocity and turbulence. Variable degrees of asymmetrical mitral regurgitation also may be present (see Figure W2-11). Continuous-wave Doppler shows the presence of a significant gradient in the left ventricular outflow tract. Dynamic left ventricular obstruction also can be present without systolic anterior motion. In the presence of reduced afterload, dehydration, or significant catecholaminergic stimulation, patients with a small hypertrophied left ventricle (typically seen in elderly patients with chronic hypertension) can develop midventricular obstruction due to hyperdynamic systolic obliteration of the left ventricular cavity (see Figure W2-9).83 These physiologic factors may predict development or worsening of left ventricular dynamic obstruction. Interplay of these factors with preexisting ventricular hypertrophy predisposes the patient to develop cardiogenic shock from this combined loss of preload and presence of dynamic left ventricular obstruction. Dynamic left ventricular obstruction has also been described in patients with acute myocardial infarction, mostly in association with apical infarction.81,84,85
In a study by Chenzbraun et al.85 in ICU patients, four patients with hemodynamic instability were found to have a small hyperdynamic ventricle on TEE. Of these four patients, three had pulmonary artery occlusion pressure greater than 20 mm Hg. A study by Poelaert et al.20 that evaluated the diagnostic value of TEE compared with pulmonary artery catheterization showed that pulmonary artery catheterization failed to diagnose the presence of hypovolemia in 44% of patients when TEE showed systolic obliteration of the left ventricular cavity, supporting a diagnosis of hypovolemia. TTE and TEE have been shown to play a key role in making the diagnosis of hypovolemia and left ventricular dynamic obstruction, leading to a dramatic impact on therapy.19,21,22,82–85