Chapter 14 Basal Ganglia
The term basal ganglia denotes a number of subcortical nuclear masses that lie in the inferior part of the cerebral hemisphere, lateral to the thalamus (Figs 14.1, 14.2). They have traditionally been regarded as including the corpus striatum, the claustrum and the amygdaloid complex. More recently, however, the working definition has been narrowed to cover only the corpus striatum and its associated structures in the diencephalon and midbrain. The reasoning behind this change is that these structures form a functional complex involved in the control of movement and motivational aspects of behaviour, whereas the claustrum is of unknown function and the amygdala is more closely related to the limbic system (Ch. 16).
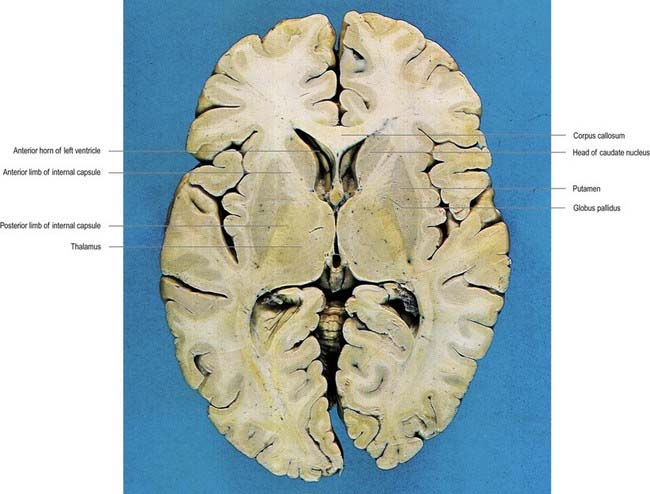
Fig. 14.1 Horizontal section of the brain.
(Dissection by E. L. Rees; photograph by Kevin Fitzpatrick on behalf of GKT School of Medicine, London.)
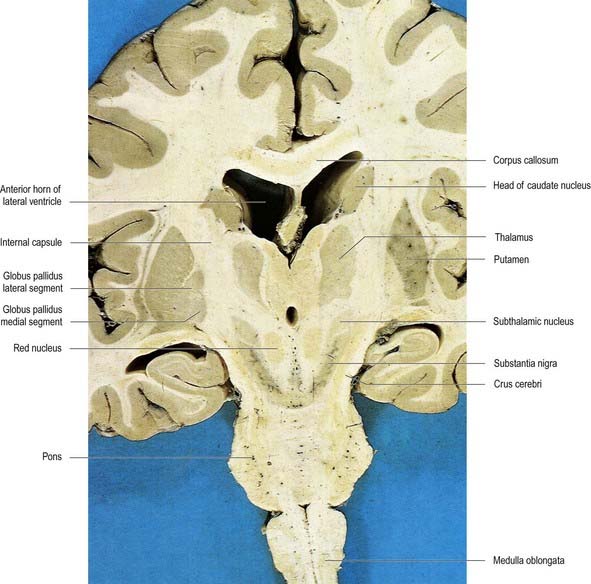
Fig. 14.2 Oblique coronal section of the brain.
(Dissection by E. L. Rees; photograph by Kevin Fitzpatrick on behalf of GKT School of Medicine, London.)
The corpus striatum consists of the caudate nucleus, putamen and globus pallidus (Fig. 14.3). Because of their close proximity, the putamen and globus pallidus were historically considered a single entity, termed the lentiform complex or nucleus. With increasing knowledge of their structure and function, however, it has become clear that the putamen is more correctly considered to be in unity with the caudate nucleus, with which it shares common chemocytoarchitecture and connections. The putamen and caudate nucleus are together referred to as the neostriatum or simply the striatum.
The striatum is considered the principal ‘input’ structure of the basal ganglia because it receives the majority of afferents from other parts of the neuraxis. Its principal efferent connections are to the globus pallidus and pars reticulata of the substantia nigra. The globus pallidus—in particular, its medial segment—together with the pars reticulata of the substantia nigra (Ch. 10) is regarded as the main ‘output’ structure because it is the source of massive efferent fibre projections, mostly directed to the thalamus.
The caudate nucleus is a curved, tadpole-shaped mass. It has a large anterior head, which tapers to a body, and a down-curving tail (Fig. 14.4). The head is covered with ependyma and lies in the floor and lateral wall of the anterior horn of the lateral ventricle, in front of the interventricular foramen. The tapering body is in the floor of the body of the ventricle, and the narrow tail follows the curve of the inferior horn, lying in the ventricular roof in the temporal lobe. Medially, the greater part of the caudate nucleus abuts the thalamus, along a junction marked by a groove, the sulcus terminalis. The sulcus contains the stria terminalis, lying deep to the ependyma (see Fig. 5.3; Fig. 14.5). The stria terminalis forms one margin of the choroid fissure of the lateral ventricle; the hippocampal fimbria and fornix form the other margin. The sulcus terminalis is especially prominent anterosuperiorly (because of the large head and body of the caudate nucleus relative to the tail), where it is accompanied by the thalamostriate vein.
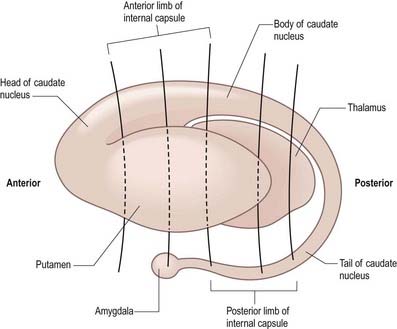
Fig. 14.4 Striatum within the left cerebral hemisphere.
(By permission from Crossman, A.R., Neary, D., 2000. Neuroanatomy, 2nd ed. Churchill Livingstone, Edinburgh.)
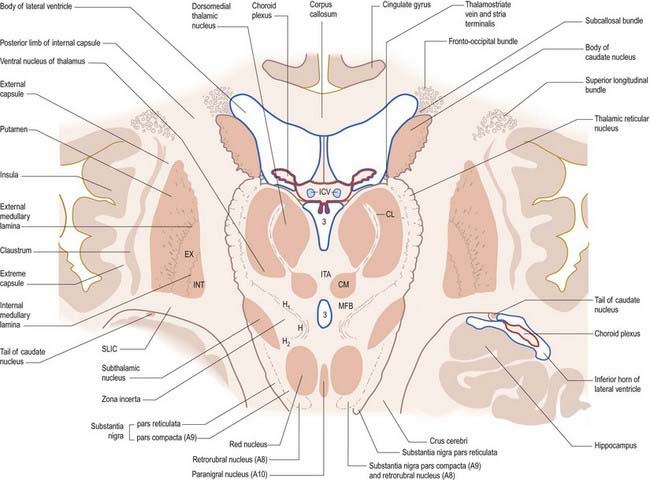
Fig. 14.5 Oblique section through the diencephalon and basal ganglia. A8, A9, A10, dopaminergic cell groups; CL, centrolateral nucleus of thalamus; CM, centromedian nucleus of thalamus; EX, external pallidal segment; H, H1, H2, subthalamic fields of Forel; ICV, internal cerebral veins in the transverse fissure; INT, internal pallidal segment; ITA, interthalamic adhesion; MFB, median forebrain bundle; SLIC, sublentiform internal capsule; 3, third ventricle.
The corpus callosum lies above the head and body of the caudate nucleus. The two are separated laterally by the fronto-occipital bundle and medially by the subcallosal fasciculus, a bundle of axons that caps the nucleus (see Fig. 14.5; Figs 14.6, 14.7). The caudate nucleus is largely separated from the lentiform complex by the anterior limb of the internal capsule (Figs 14.1, 14.6, 14.7). However, the inferior part of the head of the caudate becomes continuous with the most inferior part of the putamen immediately above the anterior perforated substance. This junctional region is sometimes known as the fundus striati (see Fig. 14.6). Variable bridges of cells connect the putamen to the caudate nucleus for most of its length. They are most prominent anteriorly, in the region of the fundus striati and the head and body of the caudate nucleus, where they break up the anterior limb of the internal capsule (see Figs 14.6, 14.7). In the temporal lobe, the anterior part of the tail of the caudate nucleus becomes continuous with the posteroinferior part of the putamen. The vast bulk of the caudate nucleus and putamen are often referred to as the dorsal striatum. A smaller inferomedial part of the rostral striatum is referred to as the ventral striatum and includes the nucleus accumbens.
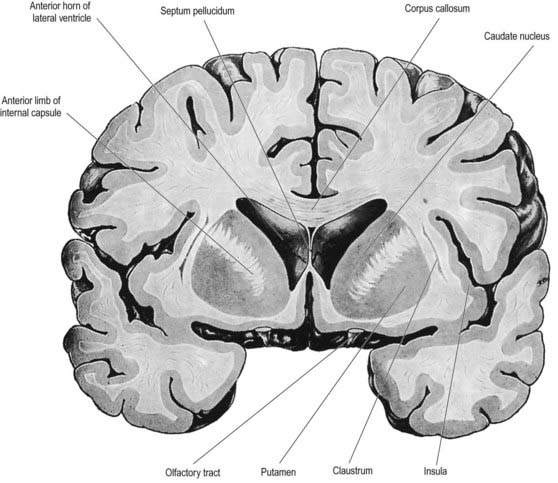
Fig. 14.7 Posterior aspect of a coronal section through the anterior horn of the lateral ventricles.
The lentiform complex lies deep to the insular cortex, with which it is roughly coextensive, although the two are separated by a thin layer of white matter and the claustrum (see Fig. 14.2; Fig. 14.8). The claustrum splits the insular subcortical white matter to create the extreme and external capsules. The latter separates the claustrum from the putamen (see Figs 16.40, 14.1, 14.2, 14.8). The lentiform complex is separated from the caudate nucleus by the internal capsule.
Inferiorly, a little behind the fundus striati, the lentiform complex is grooved by the anterior commissure, which connects inferior parts of the temporal lobes and the anterior olfactory cortex of the two sides (see Fig. 14.6). The area above the commissure is referred to as the dorsal pallidum, and that below it is the ventral pallidum.
Striatum
Neuroactive chemicals, whether intrinsic or derived from afferents, are not distributed uniformly in the striatum. For example, serotonin and glutamic acid decarboxylase concentrations are highest caudally, whereas substance P, acetylcholine and dopamine are highest rostrally. However, there is a finer-grain neurochemical organization that informs the view of the striatum as a mosaic of islands or striosomes (sometimes referred to as patches), each 0.5 to 1.5 mm across, packed into a background matrix. Striosomes contain substance P and enkephalin. During development, the first dopamine terminals from the substantia nigra are found in striosomes. Although this exclusivity does not persist after birth, striosomes in the caudate nucleus still contain a higher concentration of dopamine than the matrix does. The latter contains acetylcholine and somatostatin and is the target of thalamostriate axons. Receptors for at least some neurotransmitters are also differentially distributed. For example, opiate receptors are found almost exclusively within striosomes, and muscarinic receptors predominantly so. Moreover, the distribution of neuroactive substances within the striosomes is not uniform. In humans, the striosome–matrix patchwork is less evident in the putamen, where it appears to consist predominantly of matrix, than it is in the caudate nucleus.
The major connections of the striatum are summarized in Figure 14.9. Although the connections of the dorsal and ventral divisions overlap, a generalization can be made: the dorsal striatum is predominantly connected with motor and associative areas of the cerebral cortex, whereas the ventral striatum is connected with the limbic system and orbitofrontal and temporal cortices. For both dorsal and ventral striata, the pallidum and substantia nigra pars reticulata are key efferent structures. The fundamental arrangement is the same for both divisions. The cerebral cortex projects to the striatum, which in turn projects to the pallidum and substantia nigra pars reticulata. From these, efferents leave to influence the cerebral cortex (either the supplementary motor area or the prefrontal and cingulate cortices via the thalamus) and the superior colliculus.
The striatum also receives afferents, which are more crudely spatially organized, from the polysensory intralaminar thalamus. The cerebelloreceptive nucleus centralis lateralis projects to the anterior striatum (especially the caudate nucleus), and the cerebello- and pallidoreceptive centromedian nucleus projects to the putamen.
The aminergic inputs to the caudate and putamen are derived from the substantia nigra pars compacta (dopaminergic group A9; Figs 14.10, 14.11), the retrorubral nucleus (dopaminergic group A8; see Fig. 14.5), the dorsal raphe nucleus (serotoninergic group B7; see Fig. 14.10) and the locus coeruleus (noradrenergic group A6). The nigrostriatal input is sometimes referred to as the ‘mesostriatal’ dopamine pathway. It reaches the striatum by traversing the H fields of the subthalamus. These aminergic inputs appear to modulate the responses of the striatum to cortical and thalamic afferent influences.
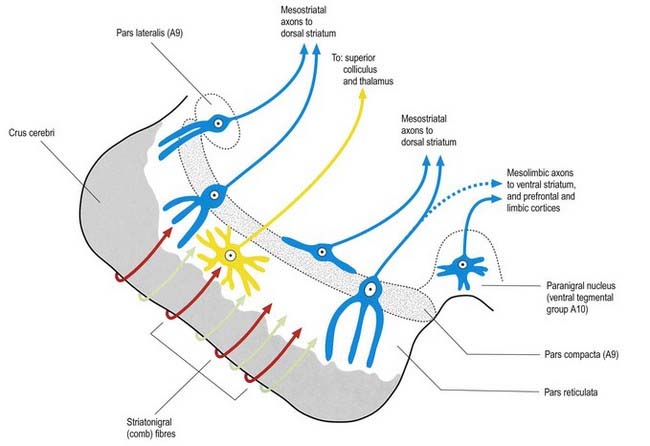
Fig. 14.11 Scheme of the organization of the substantia nigra in transverse section. Compare with Figure 14.10. Medially, there is no sharp distinction between dopaminergic cells projecting to the dorsal striatum (pars compacta, A9) and those projecting to the ventral striatum and limbic system (paranigral nucleus, A10). Dendrites of dopaminergic neurones intrude into the pars reticulata. Note the distinctive projection systems from the pars reticulata.
(From Paxinos (Ed.), 1990. The Human Nervous System, vol. 1, Webster. pp. 889–944. Modified with permission from Elsevier.)
Efferents from the striatum pass to both segments of the globus pallidus and to the substantia nigra pars reticulata, where they end in a topically ordered fashion. Fibres ending in either the lateral or medial pallidal segment originate from different striatal cells (Figs 14.9, 14.12). Those to the lateral pallidum come from neurones that co-localize GABA and enkephalin and give rise to the so-called indirect pathway. This name refers to the fact that these striatal neurones influence the activity of basal ganglia output neurones in the medial pallidum via the intermediary of the subthalamic nucleus. Other striatal neurones, which co-localize GABA and substance P/dynorphin, project directly to the medial pallidum and are therefore described as the direct pathway.