Chapter 42 Atrial Fibrillation
Epidemiology
Incidence and Prevalence
Atrial fibrillation (AF) is the most common sustained arrhythmia, with the incidence of 3.1 cases in men and 1.9 cases in women per 1000 person-years in the population younger than 64 years, rising to 19.2 per 1000 person-years in those 65 to 74 years, and as high as 31.4 to 38 in octogenarians.1 A rising proportion of the older population, markedly improved survival from previously fatal cardiovascular conditions, and a recently observed trend toward a continuous increase in the incidence of AF among younger age groups will result in a considerable increase in the number of patients with AF over the next 4 decades. The Framingham Study in the United States and the Rotterdam Study in Europe have estimated lifetime risk for development of AF to be 1 in 4 for men and women aged 40 years and older.2,3 Projected data from national databases have predicted that the number of patients with AF in the United States is expected to reach 5.6 to 15.9 million by 2050, particularly if the incidence of AF continues to rise (Figure 42-1).4–6 A similar increase in the proportion of population with AF is likely to be seen in Western Europe.3,7 However, even these projections may represent conservative estimates because of silent and short transient episodes of AF, which are likely to be diagnosed more frequently because of increased awareness of the arrhythmia, improved diagnostic tools, and a wider use of implantable rhythm-control or rhythm-monitoring devices.8
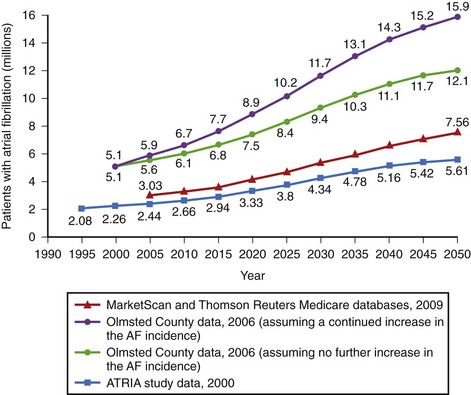
FIGURE 42-1 Estimates of the number of individuals with atrial fibrillation in the United States by 2050.
(Data from Go AS, Hylek EM, Phillips KA, et al: Prevalence of diagnosed atrial fibrillation in adults: National implications for rhythm management and stroke prevention. The Anticoagulation and Risk Factors in Atrial Fibrillation (ATRIA) Study, JAMA 285:2370–2375, 2001; Miyasaka Y, Barnes ME, Gersh BJ, et al: Secular trends in incidence of atrial fibrillation in Olmsted County, Minnesota, 1980 to 2000, and implications on the projections for future prevalence, Circulation 114:119–125, 2006; and Naccarelli GV, Varker H, Lin J, Schulman KL: Increasing prevalence of atrial fibrillation and flutter in the United States, Am J Cardiol 104:1534–1539, 2009.)
Age, Gender, and Ethnicity
A series of epidemiologic studies have identified age to be one of the major determinants of risk of developing AF, with odds of 1.1 to 5.9 depending on the initial age and morbidity of a cohort studied and the duration of follow-up.9–12 In the Framingham Heart Study, the risk of AF was increased approximately twofold per decade of age in both men and women.11 Increased rates of AF related to age are attributed to inherent changes in the atria occurring with aging (e.g., fatty metamorphosis, myocyte degeneration, fibrosis), accumulation of risk factors, and progression of the underlying disease.
Men are affected almost twice as often and are diagnosed with AF at a younger age compared with women, but recent analyses have suggested that women with AF have more comorbidities, higher mortality rate, and higher risk of stroke and treatment-related complications (e.g., proarrhythmia or bleeding) compared with their male counterparts.11–15
The prevalence of AF is higher in white populations.16–19 In the Atherosclerosis Risk in Communities (ARIC) Study, age-adjusted incidence rates of AF were highest in white men and lowest in black women (7.45 and 3.67 per 1000 person-years, respectively).17 In the health survey of 664,754 U.S. veterans, white men were significantly more likely to have AF compared with all other races except Pacific Islanders (odds ratio [OR] compared with blacks, 1.84; vs. Hispanics, 1.77; vs. Asians, 1.41; vs. Native Americans, 1.15; P < .001).18 The prevalence and distribution of associated risk factors also depended on ethnicity. Thus white men were more likely to have valvular heart disease, coronary artery disease (CAD), and congestive heart failure (CHF); blacks had the highest prevalence of hypertension; and Hispanics had the highest prevalence of diabetes. Racial differences remained after adjustment for age, body mass index, and underlying pathology. In a meta-analysis of seven randomized clinical trials in patients with acute coronary syndrome (ACS), the prospectively collected information on the development of AF indicated that the rates of AF were lower in Asian patients than in white patients (4.7% vs. 7.6%; OR, 0.65; 95% confidence interval [CI], 0.50 to 0.84; P = .001).19 This contradicts the finding of a greater prevalence of unfavorable risk factors for AF in nonwhite populations.20 Nonwhite races have also been reported to be at higher risk of intracranial hemorrhage as a complication of anticoagulation therapy (hazard ratio [HR], with whites as referent, 4.06 for Asians, 2.06 for Hispanics, and 2.04 for blacks).21 Thus the link between ethnicity and risk of AF as well as the therapy for AF remains to be further investigated.
Associated Disease and Risk Factors
AF is commonly associated with underlying cardiovascular or endocrine pathology (Figure 42-2). The primary pathologies underlying or promoting the occurrence of AF vary more than for any other cardiac arrhythmia, ranging from autonomic imbalance through organic heart disease to metabolic disorders (Table 42-1). Hypertension, heart failure, and ischemic and valvular heart disease, particularly mitral stenosis, are the most common associations and risk factors for the development and progression of AF. Valvular heart disease of rheumatic etiology, one of the main causes of AF, with an odds of 2 to 3 and greater in early studies, no longer holds its leading role but is still important in the developing countries or in the very old.9–11
Table 42-1 Risk Factors and Associations of Atrial Fibrillation
CARDIAC | NONCARDIAC | INTERVENTIONS |
---|---|---|
STRUCTURAL HEART DISEASE | PATHOLOGIC | CARDIAC |
Valvular heart disease, particularly mitral | Diabetes mellitus | Coronary artery bypass grafting |
Hypertension or increased pulse pressure | Thyrotoxicosis or subclinical hyperthyroidism | Valvular repair or replacement |
Congestive heart failure and diastolic dysfunction | Chronic obstructive pulmonary disease | Surgical correction for congenital heart disease |
Myocardial infarction | Lung cancer | NONCARDIAC |
Ischemic heart disease | Chronic renal disease | Thoracic surgery, particularly pulmonary |
Hypertrophic cardiomyopathy | Obesity (BMI) | Surgical procedures requiring general anesthesia |
Dilated cardiomyopathy | Metabolic syndrome | SUBSTANCE USE AND ABUSE |
Atrial septal defect | Sleep apnea | Alcohol excess |
Myocarditis or pericarditis | Pheochromocytoma | Caffeine (trigger) |
ARRHYTHMIAS | Electrolyte disturbances | Smoking |
Sick sinus syndrome | PHYSIOLOGICAL | Thyroxine |
Atrial tachycardia and atrial flutter | Male gender | Cytotoxic agents |
WPW syndrome (accessory pathway) | Aging | Recreational drugs (cannabis, MDMA) |
Long P-R interval | Tall stature | Anabolic steroids |
GENETIC | Endurance training | |
Familial lone AF | BIOMARKERS | |
Short QT syndrome | Atrial natriuretic peptide, brain natriuretic peptide | |
Brugada syndrome | Cross-reactive protein, tumor necrosis factor-α, interleukin-6 | |
Long QT syndrome (rare) |
AF, Atrial fibrillation; BMI, body mass index; MDMA, 3,4-methylenedioxymethamphetamine (“ecstasy”); WPW, Wolff-Parkinson-White.
Heart Failure
An increasing number of surviving patients with left ventricular impairment has led to CHF emerging as one of the leading risk factors for AF associated with a threefold to almost sixfold increase in the risk of AF.10,12,13 The prevalence of AF associated with CHF varies from 4% to 50%, depending on New York Heart Association (NYHA) class (Figure 42-3).22 The occurrence of CHF in middle age confers an 8% risk of developing AF over a 10-year period if the patient’s age at time of CHF diagnosis was 55 to 64 years, which rises to more than 30% if CHF was diagnosed at age 45 to 54 years.13
Diastolic left ventricular dysfunction with subsequent increases in filling pressures, which is common in older adults, mediates atrial remodeling and is associated with a 5.26-fold increased risk of AF.23 The incidence of AF in patients with CHF and preserved left ventricular systolic function is 20% to 30%.24
Hypertension
Hypertension is the most prevalent condition associated with AF, with a rate of approximately 70% (see Figure 42-2). It is a firmly established risk factor for the development of AF, as shown by many epidemiologic surveys, and increases the risk of AF by a factor of 1.5 to 2.10–13 The risk has been shown to rise proportionally to the level of blood pressure, although iatrogenic hypotension has been linked to AF, suggesting a J-shaped relationship between blood pressure and AF.12,25,26
Myocardial Infarction
AF is a common complication of ACS, occurring de novo in 6% to 15% of patients with myocardial infarction (MI); the incidence is higher (10% to 20%) if patients with a history of atrial tachyarrhythmias are included.27 Patients with ST-segment elevation MI (STEMI) are more likely to develop AF compared with patients with other types of ACS (8% vs. 6.4%), and the presence of early left ventricular systolic dysfunction and CHF has been consistently shown to increase the likelihood of AF by a factor of 1.58 to 3.28.27,28
Previous MI was associated with an increased risk of subsequent AF by a factor of 1.4 to 3.62, but this association is less well established for CAD without MI because these two entities have not been adequately distinguished in epidemiologic studies.9,10,12 It is reasonable to consider CAD, particularly with evidence of reversible ischemia, a risk factor because it may promote formation of the substrate for AF (e.g., due to atrial ischemia).
Diabetes
Diabetes mellitus has been identified as a risk factor for AF. In the Framingham Heart Study, diabetes mellitus was associated with a 1.4-fold increased risk of AF in men and 1.6-fold increase in women.10 Its relevance as a risk factor has been confirmed in recent population-based surveys and meta-analyses of randomized controlled studies. A report from the Veterans Health Administration Hospitals database with 845,748 participants has shown that type 2 diabetes increases the risk of AF by a factor of 2.13.29 A systematic review of 11 studies involving 108,703 cases of AF in more than 1.6 million individuals has revealed that patients with diabetes have a 40% greater risk of AF compared with their nondiabetic counterparts.30
The cause-and-effect association between diabetes and AF has not been fully elucidated as, for example, no substantial evidence that type 1 diabetes increases risk of AF exists. Patients with type 2 diabetes mellitus often have other comorbidities and risk factors that may predispose to AF, such as older age, hypertension, CHF, CAD, obesity, and sleep apnea, and the effect of confounding is significant. Although hyperglycemia may directly affect the electrophysiological properties of the atria, causing intra-atrial conduction delay and promoting structural remodeling by activation of the AGE-RAGE (advanced glycation end product–receptor for AGE) system and upregulation of circulating tissue growth factors, insulin resistance associated with type 2 diabetes may also be an important contributor.31,32
Cardiomyopathies
AF occurs in association with hypertrophic cardiomyopathy in 18% to 28% of patients, with an annual incidence of 2%.33,34 The prevalence of AF is higher in patients older than 50 years with evidence of left ventricular systolic dysfunction, and its occurrence is associated with limiting symptoms and increased morbidity from CHF (a threefold increase) and stroke (an 8- to10-fold increase) and an almost quadrupled mortality rate. A genetic predisposition to AF is seen in some forms of hypertrophic cardiomyopathy: an Arg663His mutation in the β-cardiac myosin heavy chain gene has been identified in patients with a specific phenotype of familial hypertrophic cardiomyopathy presenting with moderate septal left ventricular hypertrophy, predominantly localized in the proximal segment of the interventricular septum, and a 47% prevalence of AF.35
Dilated cardiomyopathy (DCM) can promote AF via the same mechanisms as CHF of ischemic etiology; that is, atrial overload, stretch, and fibrosis. Some types of mutations in the lamin A/C gene linked to DCM have also been shown to be associated with increased risk of progressive conduction system disease, atrial myopathy, and AF.36
Pre-excitation Syndrome
AF is found in 30% of patients with Wolff-Parkinson-White (WPW) syndrome and is associated with increased risk of sudden cardiac death (SCD).37 Unlike the atrioventricular (AV) node, accessory pathways usually exhibit rapid, nondecremental conduction, and if the effective refractory period of an accessory pathway is short (<250 ms), this may result in a rapid ventricular response during AF, with subsequent degeneration to ventricular fibrillation (VF). In some patients with an accessory pathway or a dual AV node, AV re-entrant tachycardia (AVRT) and AV node re-entrant tachycardia (AVNRT) can trigger AF.
Thyrotoxicosis
Thyrotoxicosis has a classic, but relatively minor, association with AF, whereas latent hyperthyroidism, which has been shown to be associated with an almost threefold increase in the age-adjusted incidence of AF in the Framingham Heart Study, is likely to have a greater impact in the general population.38 In the prospective population-based Rotterdam Study in older individuals, free thyroxine levels within the normal range showed a graded association with risk of AF (HR for highest vs. lowest quartile, 1.62).39 Convincing evidence that hypothyroidism may increase risk of AF does not exist.6
Smoking
Smoking as a risk factor has been debated, but recent analyses from the Atherosclerosis Risk in Communities (ARIC) and the Rotterdam studies have provided evidence linking smoking to the risk of AF in the general population, with a hazard ratio of 1.51 to 2.05 in current smokers and 1.32 to 1.49 in former smokers.40,41 In the highest tertile of accumulated smoking amount (>675 cigarette-years), the incidence of AF was 2.1 times greater than in those who never smoked.
Alcohol
High alcohol intake has been consistently associated with risk of AF, but the effects of light or moderate consumption are less clear, and some have suggested a neutral or even protective effect. A recent systematic review has reported a monotonic dose-response causal relationship between alcohol and onset of AF.42 Compared with nondrinkers, individuals consuming 24, 60, and 120 g of alcohol daily had relative risk of 1.08, 1.44, and 2.09, respectively (men), and 1.07, 1.42, and 2.02, respectively (women). Recent meta-analysis of 14 studies has shown that for each 10-mg increase per day in alcohol intake, the risk of AF increased by 1.08 (95% CI, 1.05 to 1.10; P < .001).43
Caffeine
In addition to a series of case reports, recent analyses from the Framingham Heart Study, the Danish Diet, Cancer, and Health Study, and the Women’s Health Study found no association between caffeine intake and the incidence of AF.44–46 In a canine model with enhanced AF inducibility caused by the stimulation of the ganglionated plexi, the presence of caffeine reduced the propensity for AF.47 It is possible that excessive caffeine consumption may trigger AF in individuals with a predominantly adrenergically mediated mechanism of the arrhythmia, but this association could not be detected in the epidemiologic studies.
Postoperative Atrial Fibrillation
AF may occur in about 30% of patients after isolated coronary artery bypass grafting (CABG), 40% of patients after valve surgery, and 50% of patients after combined coronary artery and valvular surgery.48 Most cases of AF occur during the first 3 to 4 days, and more than 90% have a paroxysmal form. Postoperative AF is associated with increased morbidity and mortality rates, largely because of stroke and circulatory failure and longer hospital stay. Atrial flutter (AFL) and atrial tachycardias (ATs), including multi-focal AT, are also common.
Risk factors include conventional clinical variables such as age, hypertension, left ventricular dysfunction, chronic obstructive pulmonary disease (COPD), renal impairment, and specific surgical aspects such as inadequate cardioprotection and hypothermia, right coronary artery grafting, and a longer bypass time.49 The incidence of postoperative atrial tachyarrhythmias is lower with minimally invasive techniques.
Other Risk Factors
Pneumonia is a one of the reversible causes of AF, whereas COPD, which is observed in 10% to 15% of patients with AF, increases the propensity to AF, such as by inflammatory mechanisms; therefore it has been identified as a risk factor for AF, although its exact impact is unknown.50 The presence of COPD can contribute to progression of AF to a more sustained form.51
Chronic renal dysfunction has recently been linked to increased risk of AF. In the ARIC study, reduced glomerular filtration rate (GFR), particularly in association with macroalbuminuria, increased risk of developing AF over 1 decade by 13-fold.52 In a population-based cohort of African-American and white American adults 45 years or older enrolled in the Reasons for Geographic and Racial Differences in Stroke (REGARDS) study, individuals with chronic renal failure stage 1 to 2, 3, and 4 to 5 had the age-, race-, and gender-adjusted odds ratios for AF of 2.67, 1.68, and 3.52, respectively.53
Emerging Risk Factors
New risk factors such as obesity (relative risk [RR], 1.2 to 2.3), metabolic syndrome, a prolonged P-R interval on an electrocardiogram (ECG) (RR, 1.2 to 2.7), P-wave duration (1.15 per standard deviation increase), sleep apnea (RR, 2.2 to 3), psychological stress and negative emotions, endurance athletic training (RR, 5.3), visceral adipose tissue (pericardial fat) detected by computer tomography (CT) (RR, 1.11 to 1.18), and tall stature (RR, 1.7 for height >180 cm) have all been reported in association with AF.54 A series of biomarkers of inflammation and oxidative stress (cross-reactive protein, interleukin [IL]-6, intercellular adhesion molecule (ICAM)-1, tumor necrosis factor [TNF]-α), hemodynamic stress (atrial natriuretic and brain natriuretic peptides [ANP and BNP]), and cardiac damage (troponin) have emerged as predictors of incident AF.54
Genetic Factors
Among many risk factors for AF, genetic predisposition to AF or specific genetically predetermined forms of the arrhythmia have also been identified. Among the participants in the Framingham Heart Study, a parental history of AF increased the risk of AF in the offspring by a factor of 1.4 after adjustment for AF risk factors and AF-related genetic variants (Figure 42-4).55 Genetic variation may play even a greater role in the pathogenesis of lone AF (see below). AF in association with known genetic conditions such as Brugada syndrome and short QT interval syndrome is highly prevalent. The genetic substrate for AF ranges from a large kindred with monogenic forms of AF with rare genetic mutations and high penetrance to common genetic polymorphisms in a variety of different genes in general populations.
Monogenic mutations associated with AF have been described in the number of genes encoding several potassium channels (e.g., KCNQ1 and KCNE2, which encode α- and β-subunits, respectively, of the IKs channel; KCNA5 and KCNJ2, encoding Kv1.5 and Kir 2.1 channels underlying the IKur and IK1 currents, respectively), and α- and β-subunits of the sodium channels (SCN5A and SCN1/2B) as well as a number of non–ion channel mutations in the nucleoporin gene (NUP155), connexin-40 gene (GJA5), and ANP gene.56 However, monogenic forms of AF are rare and, at the population level, AF heritability is likely to be determined by aggregate effects of multiple common genetic variants interacting with environmental factors.
Population-based genome-wide association studies (GWASs) have identified a variety of single-nucleotide polymorphisms near the PITX2 (paired-like homeodomain 2) gene on chromosome 4q25 that almost double the susceptibility to AF in general populations of European and Asian descents.57,58 Other common variants include ZFHX3 (zinc finger homeobox 3) on chromosome 16q22, and KCNN3 (a calcium-activated, small conductance potassium current, which is also involved in atrial repolarization) on chromosome 1p21.
Lone Atrial Fibrillation
Lone AF poses a higher risk of heritability. Relatives of patients with lone AF are at a substantially increased risk of developing AF compared with the general population, suggesting a Mendelian genetic contribution to the etiology of this common trait. Analysis of the Danish Twin Study has revealed an increased risk of AF among monozygotic twins compared with dizygotic twins (22% vs. 12%), with the heritability of AF estimated at 62% (compared with 80% heritability of height and 35% heritability of the QT interval).58,59
GWASs have linked an SNP in KCNN3 to a greater risk of lone AF with an odds ratio of 1.52.60 The angiotensin-converting enzyme (ACE) insertion/deletion (I/D) polymorphism was associated with electrical remodeling in patients with lone AF.61
Vagally Mediated and Adrenergically Mediated Atrial Fibrillation
The balance between sympathetic and vagal influences plays an important role both in the initiation and prediction of AF. Two types of autonomic-induced AF exist. Adrenergically mediated episodes of AF are typically triggered by exercise and emotional stress (Figure 42-5), commonly associated with polyuria, and occur mainly during the day. Vagally mediated AF is characterized by male predominance, younger age, minimal tendency to progress to permanent AF, and onset at rest or at night. Episodes of vagal AF are typically preceded by progressive bradycardia. β-Blockers are treatment of choice in AF with a predominant adrenergic component but may worsen symptoms in patients with vagally mediated AF.
However, patients who can be classified in terms of sympathetic or adrenergic forms of AF typically represent the extreme of either sympathetic or parasympathetic influence, and the overall prevalence of typical vagally or adrenergically mediated AF is relatively low. In 1517 patients with paroxysmal AF included in the Euro Heart Survey, vagally mediated AF was found in 6% and adrenergically mediated AF was found in 15%.62
Classification
The 2010 European Society of Cardiology (ESC) guidelines introduced a longstanding persistent category for AF, which typically lasts for 1 year or more before a rhythm control intervention is attempted (Figure 42-6).63 This allows for a permanent form to be redesignated as longstanding persistent AF, that is, amenable to restoration and maintenance of sinus rhythm, mainly because of the increased success of ablation therapies.
Progression of Atrial Fibrillation
AF is a progressive disease because of the continuous remodeling of the atria caused by the AF itself, changes associated with aging, progression of underlying heart disease, and genetic and environmental factors. Progression from first-diagnosed or recurrent paroxysmal AF to persistent or permanent AF occurs, on average, at the rate of 5% to 15% per year, depending on a number of factors such as age at presentation and the presence of underlying heart disease (Table 42-2).64,65
Table 42-2 Rates of Progression of Paroxysmal Atrial Fibrillation to Persistent or Permanent Atrial Fibrillation
Factors, independently associated with the progression of AF in the European Survey on AF, formed a HATCH score to predict progression of AF (hypertension, age >75 years, transient ischemic attack [TIA] or stroke, COPD, history of stroke or heart failure). CHF and stroke were assigned 2 points each and the remaining factors 1 point each. Almost half the patients with a high HATCH score of 6 to 7 had AF progression after 1 year compared with only 6% of those with a HATCH score of 1. Rates of progression are higher in patients who presented with first-onset persistent AF, with 35% to 40% developing permanent arrhythmia by the end of the first year since diagnosis.66 Pursuing the aggressive rhythm control strategy with antiarrhythmic drugs, pulmonary vein ablation or both and, when necessary, cardioversion can alter the natural course and prevent or delay progression of AF.
Progression of AF is linked to structural atrial remodeling, accumulation of electrically silent fibrotic tissue, and transformation of AF from a primary electrical disorder requiring specific triggers such as atrial premature beats (APBs), pulmonary vein tachycardia, and neurohumoral stimuli (trigger-dependent AF) into a substrate-dependent variety (Figure 42-7). Patients with lone AF have been reported to have the lowest progression rates of approximately 1% to 2% per year.67 Atrial remodeling occurs in both lone AF and AF associated with cardiovascular disease, but it is thought to be more pronounced in the latter. However, recent studies employing delayed enhancement magnetic resonance imaging (MRI) techniques have demonstrated the presence of extensive fibrosis primarily accumulated in the left atrial posterior wall in patients with lone AF compared with healthy individuals without AF.68 Patients with lone AF were found to have a similar amount of fibrosis of the left atrium as those with associated cardiovascular and metabolic disease.
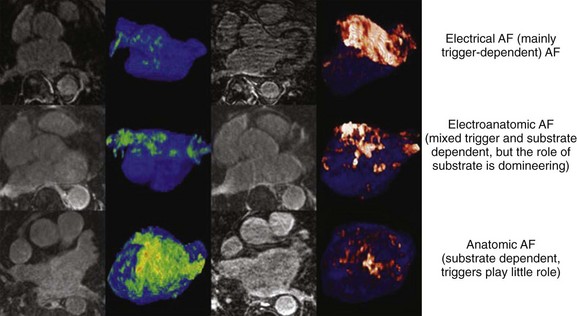
FIGURE 42-7 Stages of progression of atrial fibrillation assessed by delayed-enhancement magnetic resonance imaging.
(From Mahnkopf C, Badger TJ, Burgon NS, et al: Evaluation of the left atrial substrate in patients with lone atrial fibrillation using delayed-enhanced MRI: Implications for disease progression and response to catheter ablation, Heart Rhythm 7:1475–1481, 2010.)
Consequences of Atrial Fibrillation
The clinical significance of AF lies predominantly in high risk of ischemic stroke and heart failure and increased subsequent death. The occurrence of AF is commonly associated with worsening of pre-existing cardiovascular disease. New-onset AF in CHF has been linked to clinical deterioration and poor prognosis.22 Patients with concomitant AF and hypertension had a two- to threefold greater risk of developing stroke and CHF or dying.69 AF is associated with increased in-hospital and long-term mortality rates in patients with MI.27,70 AF leads to more hospital admissions than any other arrhythmia and presently costs approximately 1% of the health care budget in the Western countries.
Stroke
The presence of AF has been estimated to increase the risk of stroke by about fivefold.71 Whereas the effects of hypertension, CAD, and CHF on the risk of stroke become progressively weaker with advancing age, the impact of AF remains significant. In the Framingham Study, the annual risk of stroke attributable to AF among patients aged 50 to 59 years is 1.5% and increases to 23.5% in those older than 80 years.71 Approximately 15% of neurologically asymptomatic patients enrolled in the Veterans Affairs Stroke Prevention in Nonrheumatic Atrial Fibrillation (SPINAF) study had evidence of one or more previously undiagnosed cerebral infarcts on CT images of the brain.72 The risk is considerably higher in patients with previous stroke or TIA.
Strokes associated with AF are usually more severe and confer a high risk of subsequent morbidity, mortality, and poor functional outcome independent of the underlying heart disease. Risk of recurrent stroke is high, particularly within the first year, because of hemostatic abnormalities following the index event. The report from the Framingham Study has revealed that stroke associated with AF was nearly twice as likely to be fatal, and functional deficits were more likely to be severe among survivors.73 Nearly three quarters of stroke victims with AF were severely dependent in activities of daily living (ADLs) compared with about one third of their counterparts with sinus rhythm.
Dementia
Dementia as a consequence of AF has been only recently recognized. Evidence from the large ongoing prospective Intermountain Heart Collaborative Study database, AF was independently associated with all dementia types, and the risk was the highest in the younger group (<70 years), with an HR of 2.2 for vascular dementia, 3.34 for senile dementia, and 2.3 for Alzheimer disease after adjustment for age and comorbidities.74 According to data from the Olmsted County, Minnesota, in patients with a mean age of 71 years, the rate of dementia associated with AF was 2.7% at 1 year and 10.5% at 5 years.75 The diagnosis of dementia in the presence of AF was associated with a marked increased risk of subsequent mortality by factor of 3.49 in men and 3 in women.
Tachycardia-Induced Cardiomyopathy
AF may compromise left ventricular systolic function and worsen CHF because of fast, uncontrolled ventricular rates, irregularity of ventricular response, and loss of atrial systolic input. Loss of AV synchrony is associated with impaired diastolic filling, reduced stroke volume, and elevated diastolic atrial pressure, resulting in an approximately 20% reduction in cardiac output.22 An irregular ventricular response further decreases cardiac output and increases right atrial pressure and pulmonary capillary wedge pressure independent of rate. Increased adrenergic stimulation to maintain adequate cardiac output in the setting of heart failure will facilitate AV conduction and favor the progression of cardiomyopathy. AF has been reported to confer a threefold risk of worsening CHF.76 In patients with CHF secondary to diastolic dysfunction, the occurrence of AF may be particularly hazardous.
Less commonly, AF with fast, uncontrolled ventricular rates may cause symptomatic left ventricular dysfunction in patients with no or minimal structural heart disease. Such ventricular dysfunction associated with significant heart dilatation and symptoms of heart failure is termed tachycardia-induced cardiomyopathy. It is usually applied to persistent forms of the arrhythmia, and it is generally accepted that sustained ventricular rates of above 120 beats/min may pose a risk but may also occur in individuals with frequent paroxysms, in whom rate control is difficult. Tachycardia-induced cardiomyopathy may reverse completely after sinus rhythm is restored or adequate ventricular rate control is achieved either by pharmacologic or nonpharmacologic means (Figures 42-8 and 42-9).
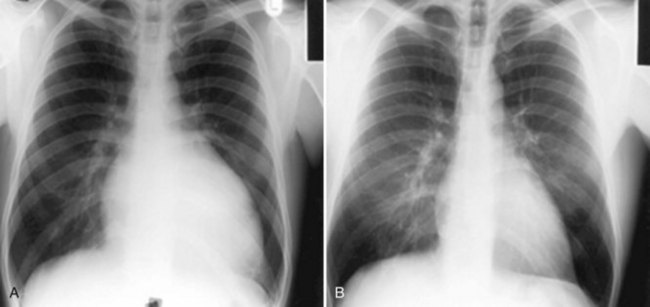
FIGURE 42-8 Reversal of cardiac enlargement on chest radiographs 3 months after restoration of sinus rhythm.
Hospitalizations
According to some surveys, the number of AF-related hospitalizations almost tripled in 2000 compared with the previous 2 decades and continues to grow, probably because of an increasing proportion of the population surviving to very old age with greatly improved health care. Patients with AF can be admitted for treatment of AF (e.g., cardioversion, initiation of antiarrhythmic drug therapy, or ablation) and cardiovascular adverse events (e.g., proarrhythmia, progression of heart failure, stroke, or MI) or noncardiac causes. Analysis from the Atrial Fibrillation Follow up Investigation of Rhythm Management (AFFIRM) trial has linked cardiovascular hospitalization with increased risk of subsequent death.77 The association between cardiovascular hospitalization and adverse outcome has also been reported in the Stockholm Cohort Study of Atrial Fibrillation, which showed that individuals who spent more than 2% of their follow-up period in the hospital with a cardiovascular diagnosis had a significantly higher mortality rate than those who had spent less time in the hospital (36 vs. 8.2 deaths per 100 patient-years).78 Time in-hospital greater than 2% increased the risk of subsequent death by a factor of 2.5, and rehospitalization for cardiovascular reason within 3 months increased the risk of death by a factor of 1.4 in the early cohort and 2.43 in the later cohort, for which a better account of therapy was available.
Quality of Life
Patients with AF generally have impaired quality of life compared with healthy control subjects—matched samples from the general population or patients with stable CAD—and is similar on most scales to health impairment seen in patients with MI or CHF and more significant structural heart disease.79 The degree of reported reductions in quality of life is determined by the form of AF and the presence of other diseases that may affect the individual perception of health. Thus patients with multiple comorbidities are more likely to report poorer quality of life compared with patients with established AF and those with less significant underlying heart disease. Paroxysmal AF is usually symptomatic and is associated with impaired quality of life. Patients with this variety report better quality of life and had significantly higher scores in physical functioning, vitality, mental health, and emotional health when they perceive themselves to be in sinus rhythm.80 Women are more likely to report poor quality of life compared with men, despite comparable severity of underlying pathology. Even patients with asymptomatic AF report a significantly poorer perception of general health and decreased global life satisfaction compared with their healthy counterparts, despite similar scores on all other scales.81
Both antiarrhythmic drug therapy and ablation-based therapies have been shown to render a significant proportion of AF asymptomatic, which may result in improvement in quality of life. Of interest, when quality of life was compared in patients with AF who were randomly assigned to AV node ablation or AV node modification, ablation was shown to result in a greater improvement in quality of life and reduction of symptoms compared with modification, probably because of better control of the rate and regularity of ventricular response after abolishing fibrillatory conduction to the ventricles.82 Recently, several AF-specific quality of life questionnaires have been proposed, but all require validation.83,84
Silent Atrial Fibrillation
AF is typically associated with a variety of symptoms: palpitations, dyspnea, chest discomfort, fatigue, dizziness, and syncope (Figure 42-10).85 The paroxysmal forms are more likely to be symptomatic and frequently present with specific symptoms, whereas the permanent forms are usually associated with less-specific symptoms. However, a substantial proportion of patients may not experience any symptoms or these symptoms may be subtle and may not be reported.
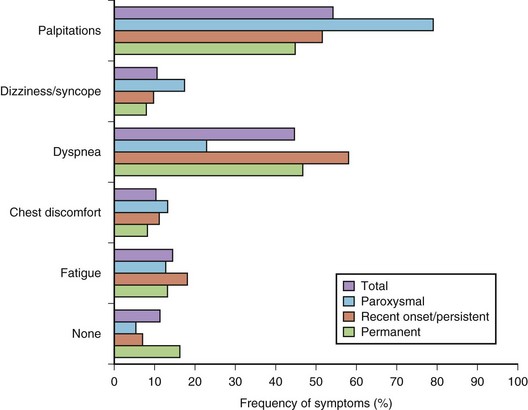
FIGURE 42-10 Distribution of symptoms in different forms of atrial fibrillation.
(Modified from Lévy S, Maarek M, Coumel P, et al, on behalf of the College of French Cardiologists: Characterization of different subsets of atrial fibrillation in general practice in France: The ALFA Study, Circulation 99:3028–3035, 1999.)
The prevalence of sustained silent AF found incidentally during routine physical examinations, preoperative assessments, occupation assessments, or population surveys is believed to be 25% to 30%, but modern implantable rhythm control devices such as pacemakers and implantable cardioverter defibrillators (ICDs) have revealed that up to 50% to 60% may have unsuspected episodes of the arrhythmia, with almost half of these lasting more than 48 hours.8,86 Patients with unrecognized AF do not receive appropriate preventive therapy and are at greater risk of stroke or, in the case of persistent rapid ventricular rates, tachycardia-induced cardiomyopathy. For example, AF may be found incidentally in about 20% to 25% of admissions for stroke.
Studies that used regular ECG monitoring have demonstrated that up to 90% of recurrences may go unreported by patients but can only be detected by daily transtelephonic ECG transmission (Figure 42-11).87 The clinical significance of very short paroxysms of AF, which are difficult to detect by conventional methods of rhythm monitoring but can be logged by implantable device diagnostics, has recently been demonstrated in the The Relationship Between Daily Atrial Tachyarrhythmia Burden from Implantable Device Diagnostics and Stroke Risk (TRENDS) study in patients treated with a pacemaker for sinus node dysfunction.88,89 AF burden of 5.5 hours or greater on any of 30 prior days doubled the risk of a thromboembolic event.
Furthermore, an exact definition of “asymptomatic” AF does not exist. A patient may claim to have no symptoms but report feeling much better after cardioversion. Studies have also shown that quality of life is reduced even when a patient is classified as asymptomatic.81 Level of acceptable symptoms may also vary according to age and comorbidities.
Mortality
Association of AF with death has been demonstrated in epidemiologic studies. Data from the Framingham Study suggest that the risk of death conferred by AF is nearly doubled even in the absence of identifiable structural heart disease.90 The risk of death associated with AF is significantly higher in women than in men (OR, 1.9 vs. 1.5). Data from other epidemiologic studies are convincing (Table 42-3). AF is an independent predictor of death in patients with a range of cardiovascular pathologies: CHF, hypertension, MI with left ventricular dysfunction, and ACS. AF increases cause-specific mortality: SCD, death from heart failure, and fatal strokes. A mechanistic basis for the link between AF and each of the components of total mortality does exist (Figure 42-12). Although the exact mechanism by which AF can increase the risk of SCD in the general AF population (apart from rare cases of SCD in WPW syndrome) has not been well established, proarrhythmia from antiarrhythmic drugs and ischemia-induced VT and VF, particularly in patients with severe underlying disease, are plausible explanations.
Current data do not offer a definitive answer whether AF truly is an independent risk factor for death or whether it is a marker indicating a sicker patient. However, even lone AF has been reported to entail 4.2-fold increase in all-cause mortality and an almost twofold increase in cardiovascular mortality, including SCD, in the Paris Prospective Study.91
Costs
The high lifetime risk for AF and increased longevity underscore the important public health burden imposed by AF across the world. The majority of AF expenditure constitutes hospital charges for frequent and often prolonged admissions for AF and particularly its costly complications such as stroke and heart failure. In addition, the cost of caring for patients with other cardiovascular pathologies is significantly higher in the presence of AF. The cost of caring for patients with AF also continues to be significantly higher during the 2 to 3 years after initial hospitalization. The arrhythmia costs of the health care budget in the United Kingdom and has almost doubled (0.9% to 2.4%) over the past 5 years.92