Chapter 13 Assessment of Right Ventricular Function
The right ventricle (RV) is often overlooked because most cardiologists focus their attention on the left ventricle (LV). Early work characterizing ventricular anatomy and physiology was performed on the LV and then assumed to be similar in the RV. Methods for measuring ventricular volume and function from the various imaging modalities were similarly developed for the LV and then applied to the RV. However, more recent studies have gradually revealed the differences between the two ventricles, and interest in the RV has risen because of recognition of the impact of RV dysfunction on patient prognosis, the increasing survival of patients with congenital heart disease to adulthood, and the rising incidence of pulmonary hypertension. Although the complex shape of the RV has long been recognized, it could previously only be glimpsed from two-dimensional (2D) images; the recent advent of three-dimensional (3D) surface reconstruction techniques for the RV has enabled new appreciation for the variety of phenotypes in which the RV can present in various conditions (Figure 13-1).
Visualization of the Right Ventricle
The sector width in current ultrasound equipment is too narrow to contain both the LV and RV. Therefore, when acquiring the ultrasound study, it is necessary to ensure that the RV is completely visualized. This is easily accomplished by centering the view on the RV in both parasternal (Figure 13-2) and apical views (Figure 13-3).1 Additional nonstandard views may be needed in patients with RV dilation. The four-chamber view can visualize the cardiac apex in normal hearts. However, centering of the RV is needed to visualize the apical bulging that may occur in hemodynamic overload states (see Figure 13-3).2
Volume Measurement
Two-Dimensional Approaches
The RV is notorious for its complex shape, which defies comparison with a geometric reference figure. In contrast, LV volume can be accurately measured from single or biplane views by comparison with an ellipsoid of revolution using the area-length method.3 Early attempts to measure RV volume from angiograms used the formula V = kA1A2/L, where A1 and A2 are the areas of the RV in the two views, L is the length of the RV long axis, and k is a constant. Depending on the value of k and how L is defined, the RV was compared with a parallelepiped, ellipsoid of revolution (area-length method), triangular prism, or pyramid.4–6 When these methods were compared with in vitro hearts or models, the disk summation method proved to be the most accurate.7,8 The area-length method also performed well from the projection views of angiograms but proved inaccurate on 2D echocardiograms.9
Models that take advantage of ultrasound’s tomographic, rather than projection, imaging were also developed. Levine and colleagues wrote: “A geometric structure can be constructed resembling the right ventricle with respect to its overall form and body segment, and such a structure can have a volume equal to 2AL/3 without unreasonable restriction of its dimensions,” where A is the area in one view and L spans the RV in the other, roughly orthogonal view (Figure 13-4).10,11 However, these models require subcostal views that may be obtainable in only 52% of patients older than 5 years.9
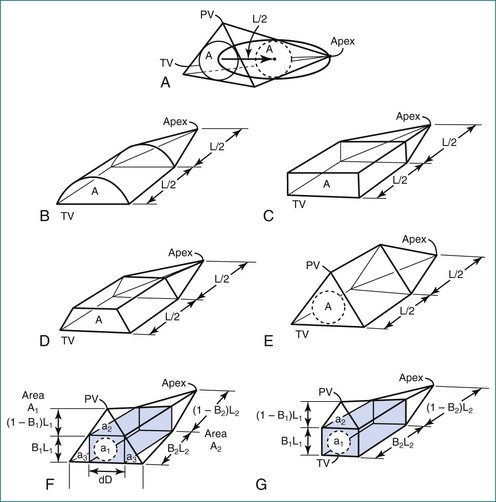
Figure 13-4 Geometric models with volume = 2AL/3. PV, pulmonary valve; TV, tricuspid valve.
(From Levine RA, Gibson TC, Aretz T, et al: Echocardiographic measurement of right ventricular volume. Circulation 69:497–505, 1984.)
Because of the inaccuracy in volume measurement, assessment of right ventricular ejection fraction (RVEF) based on 2D echocardiography (2DE) is not recommended.1,9,12 Instead, visual assessment is performed to gauge RV size relative to that of the LV (Figure 13-5; Video 13-1).13 Normally, the RV is only two thirds the size of the LV in the apical four-chamber view; the LV forms the apex of the heart and is round in short-axis views throughout the cardiac cycle. Deviations from this pattern may indicate RV dilation, but careful examination of multiple views is recommended for confirmation of the diagnosis because the size of the RV varies with the angle of the plane (Figure 13-6).1
Three-Dimensional Approaches
3D analysis entails delineating the RV from multiple views, a time-consuming task that is rendered more difficult by heavy trabeculations, particularly in hypertrophied RVs. For analysis of magnetic resonance imaging (MRI) scans, a recommendation has been made to trace the endocardial contour outside the trabeculations to maximize reproducibility (Figure 13-7).14 Another issue is the definition of end systole. Because of the asynchronous contraction of the sinus and infundibulum, the timing of minimum chamber area will vary from region to region and from slice to slice.15 However, frame-by-frame analysis has shown that selection of this time point from the four-chamber view and application of its systolic interval to the entire RV provide a highly accurate measurement of end-systolic volume.16
The disk summation method avoids assumptions concerning the shape of the RV because it does not compare the RV to a geometric figure, although it does assume that the cross-sectional contour is elliptical or hemielliptical.17–19 Consequently, even pathologically misshapen ventricles, which are commonly found in congenital heart defects, can be accurately measured. The contours of the RV endocardium are traced from parallel views, the area of each contour is multiplied by the interplanar distance to compute the volume of each “slice,” and the slice volumes are summed to compute the volume of the RV. With echocardiography, the RV is sliced into parallel short-axis views. For MRI, alternate-slice prescriptions have been advocated to more easily visualize the tricuspid annulus and define the basal limits of the RV.20 A disadvantage of 3DE performed with a matrix array transducer is that the RV cannot be imaged in its entirety in a significant number of adult patients within the single apical scan that is most commonly used to acquire the image data. This disadvantage is particularly present when the RV is enlarged, the exact situation in which quantification of RV function is important. For both echocardiography and MRI, it is advisable to trace additional views orthogonal to the short-axis “stack” for assistance in delineating the apex, the structures in the basal slice, and the basal bulge (Figure 13-8).21 Although the RV might be visualized in RV-centered images alone, this should only be done when it is certain that the LV does not need to be visualized.
An alternative to volumetric 3DE is acquisition of multiple 2D views while tracking the spatial location and orientation of the view planes for offline processing. The RV endocardial surface is reconstructed after tracing the images, and volume is computed from the 3D surface. An advantage of this approach is the use of freehand scanning so that views providing optimal image quality are acquired. As for analysis of volumetric datasets using disk summation, multiple views must be traced. Three methods have been validated for 3D reconstruction of the RV from manually traced borders. The method of Jiang and associates22 was based on deforming a spherical template to fit traced borders (Figure 13-9). The method was highly accurate on in vivo testing (r = 0.99 for end-diastolic volume; r = 0.98 for end-systolic volume; r = 0.98 for ejection fraction (EF); and r = 0.985 for RV free wall mass).
Buckey and colleagues23 swept the RV from a fixed transducer location in five-degree increments to define a series of wedges whose volumes were computed and summed to determine the RV volume. Accuracy in vitro was excellent (r = 0.95 and r = 0.96, respectively, for short-axis and apical scanning), but the entire RV had to be visualized from a single transducer position.
In the piecewise smooth subdivision surface (PSSS) method, a triangulated control mesh is designed as a model that is fit to traced borders. Parts of the control mesh can be marked as sharp around valve orifices and along the RV free wall to the septal edge (Figure 13-10). The PSSS method’s accuracy for RV volume and mass has been validated (r = 0.99 and r = 0.93, respectively), and it is the only method shown to reproduce the 3D shape of the LV and the RV with anatomic accuracy.24,25
Although these three techniques are accurate for measuring RV volume, the requirement for tracing the RV border in multiple images has precluded their clinical application. One approach for reducing the human workload is to reduce the number of borders that need be traced. However, after comparing the results obtained with disk summation over 2 to 16 slices, Chen and associates26 still found eight slices to be the “optimal choice for accurate and convenient measurement” of mass as well as volume.
Automated border detection has been stymied by the inherently noisy nature of the images, the frequent signal dropout, and the heavy trabeculation of the RV. However, TomTec Imaging Systems (Munich, Germany) markets a semiautomated method applicable to 3DE (Figure 13-11) that has been extensively validated.27–29
An alternative approach to reducing the workload of manual tracing is to use knowledge of the expected shape of the RV and the range of shapes that it can adopt in disease processes. The method marketed by VentriPoint, Inc. (Seattle, WA) is semiautomated like TomTec’s, but the user traces points at anatomic landmarks (Figure 13-12) rather than whole borders.30
Function Measurement
The American Society of Echocardiography’s guidelines state that “in selected patients with RV dilation or dysfunction, 3DE using the disk summation method may be used to report RVEF.”1 However, 3DE is not universally available, so surrogate parameters based on a single 2D view have been proposed for estimation of global RV function. The analysis usually is performed on the apical four-chamber view because of the predominantly longitudinal contractile pattern of the RV, which lacks the LV’s middle layer of circumferential fibers.31,32 The most commonly used parameters are fractional area change and tricuspid annular plane systolic descent (TAPSE).33
A major disadvantage of the single-view approach to RV function assessment is that it provides a limited perspective. The relationship between TAPSE and EF, for example, is weak if there is tricuspid regurgitation or if longitudinal shortening of the RV differs from the function of regions not visualized in the four-chamber view.34,35 In both volume and pressure overload, analysis of short-axis contours has shown dilation and dysfunction, whereas longitudinal analysis showed poor correlation between TAPSE and EF.36,37