Chapter 37 Assessment of Myocardial Viability with Thallium-201 and Technetium-Based Agents
INTRODUCTION (See Chapter 36)
In the current era of revascularization surgery and interventional cardiology, the assessment of myocardial viability has become an integral component of the diagnostic evaluation of patients with coronary artery disease (CAD) and depressed left ventricular (LV) function. It is now well established that LV dysfunction is not always an irreversible process related to previous infarction, as once was widely believed. Regional and global ventricular function may improve substantially and even normalize after reperfusion therapy for acute myocardial infarction1–5 and after myocardial revascularization procedures in patients with chronic CAD.6–9 This potentially reversible form of LV dysfunction is known as myocardial hibernation, indicating a condition in which myocardial contractility has been reduced in the setting of a sustained reduction in myocardial blood supply.9–12 The conceptual framework of hibernation has been challenged in recent years, and an alternative concept has been proposed of persistent LV dysfunction caused by repeated episodes of myocardial ischemia leading to repetitive stunning.13,14 This latter mechanism for reversible LV dysfunction in chronic CAD has gathered support from excellent experimental models of repetitive ischemia in the setting of a chronic coronary artery stenosis.15–18 Independent of the mechanism, which is difficult to determine clinically in individual patients, the important clinical issue is that viable but dysfunctional myocardium in patients with chronic CAD will improve in function only if identified and revascularized. Imaging to evaluate the presence and extent of viable but dysfunctional myocardium has become an important component of the clinical assessment of patients with CAD and impaired LV function,19,20 particularly among patients who are possible candidates for myocardial revascularization. These procedures are often accompanied by high operative morbidity and mortality rates in this subset of patients. On the other hand, the large subgroup of patients with moderate to severe LV dysfunction is at considerable risk for death during the course of medical therapy, and these patients potentially have the most to gain from successful revascularization in terms of survival (Fig. 37-1).21
Although the percentage of patients showing an important reversal of LV dysfunction after revascularization varies among reported series (probably as a result of patient selection factors and revascularization techniques), it is not inconsequential. It is estimated that 25% to 40% of patients with chronic CAD and LV dysfunction have the potential for significant improvement in LV function after revascularization (Fig. 37-2).6–8,22,23 These findings have several implications. First, given the important relationship between LV function and survival (see Fig. 37-1), the improvement in LV function after revascularization may translate into an improvement in survival. Although definitive data tying improved function to improved survival are lacking, many recent retrospective studies have begun to establish this point, as discussed later. Second, the decision to proceed with revascularization in patients with moderate to severe LV dysfunction is often difficult. These patients undergo coronary artery bypass surgery or percutaneous coronary intervention with considerable risk of procedure-related morbidity and mortality. The perioperative mortality rate associated with surgical revascularization of patients with severe LV dysfunction is as high as 10%. Hence, accurate methods to detect viable myocardium distal to a coronary stenosis, with the potential for reversal of LV dysfunction, are essential to select prospective patients in whom these risks are justified.
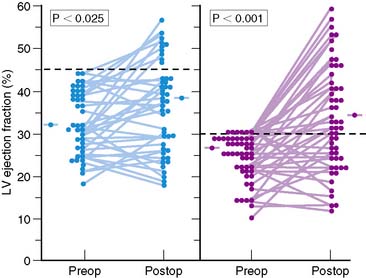
Figure 37-2 Left ventricular (LV) ejection fraction at rest shown by radionuclide ventriculography before (Preop) and after (Postop) coronary artery bypass surgery in patients with preoperative LV dysfunction in two surgical series.8,20 Although surgery resulted in only a small increase in mean ejection fraction, substantial increases were observed in a substantial subset of patients, with normalization of ejection fraction occurring in many patients.
(From Elefteriades JA, Tolis G Jr, Levi E, et al: Coronary artery bypass grafting in severe left ventricular dysfunction: Excellent survival with improved ejection fraction and functional state, J Am Coll Cardiol 22:1411–1417, 1993; and Bonow RO, Dilsizian V: Thallium-201 for assessment of myocardial viability, Semin Nucl Med 21:230–241, 1991.)
Several clinically reliable physiologic markers can be used to assess myocardial viability. Indexes of regional coronary blood flow, regional wall motion, and regional systolic wall thickening are accurate markers of viability if they are normal or near normal. However, these indexes have major limitations in identifying viable myocardium when they are reduced or absent. By definition, regional perfusion and systolic function (regional wall motion and wall thickening) are severely reduced or absent in patients with hibernating myocardium,7,9–11,24 despite maintenance of tissue viability. Other patients have preserved blood flow at rest but recurrent ischemic episodes during stress that lead to persistent contractile dysfunction from repetitive stunning.1,25–29 In these latter patients, indexes of wall motion and wall thickening are also imprecise markers of viability. More recently, cardiac magnetic resonance imaging (MRI) has become a valuable tool for the assessment of myocardial viability. In addition to functional information, areas of hyperenhancement on delayed imaging after gadolinium injection correlate well with areas of scarring.30–32
THALLIUM-201 IMAGING TO ASSESS MYOCARDIAL VIABILITY
Cellular viability requires intact sarcolemmal function to maintain electrochemical gradients across the cell membrane and preserved metabolic activity to generate high-energy phosphates. These processes require adequate myocardial blood flow to deliver substrates and washout metabolites. The retention of 201Tl is an active process that is a function of cell viability and cell membrane activity as well as blood flow. Therefore, in theory, 201Tl should be taken up and retained by viable myocardium regardless of whether systolic function is preserved. Thus, regional thallium activity, even in asynergic regions, should be an accurate marker of viability and should predict improvement of regional contraction after revascularization.19,27,33–37
Stress-Redistribution Imaging
Several recent studies of patients with chronic CAD showed that blood flow under basal conditions may be normal or near normal in myocardial regions, despite severe segmental dysfunction, and that this regional dysfunction improves after myocardial revascularization.1,25,26,28,29 In these patients with perfusion-contraction mismatch, the segmental dysfunction is believed to arise from repeated episodes of myocardial ischemia that lead to repetitive stunning, rather than true myocardial hibernation, which, by definition, requires reduced blood flow under basal conditions. Although the relative prevalence of stunning versus hibernation as a causative mechanism for reversible contractile dysfunction in patients with chronic CAD is uncertain, it appears that both processes occur, but most patients have a form of repetitive stunning. In these patients, the finding of reversible ischemia may be the key to determining the viability of dysfunctional segments, which is readily accomplished with stress thallium imaging. Thus, thallium redistribution in an asynergic region with a defect shown on stress imaging predicts improvement of regional contraction after revascularization (Fig. 37-3).37,38 However, many regions of severely ischemic or hibernating myocardium appear to have irreversible thallium defects on standard exercise-redistribution imaging; hence, the negative predictive value of an irreversible 201Tl defect is relatively poor. Up to 50% of regions with “irreversible” thallium defects improve in function after revascularization.38–43 Thus, standard stress-redistribution thallium imaging has an excellent positive predictive value, but a suboptimal negative predictive value. It is generally accepted that stress 4-hour redistribution imaging does not provide satisfactory precision in differentiating between LV dysfunction arising from infarcted versus hibernating myocardium. This technique often underestimates the presence of viable myocardium and hence the potential for recovery after revascularization.
This concept is supported by studies directly comparing the results of PET imaging and exercise-redistribution thallium scintigraphy. In four studies, between 38% and 47% of apparently irreversible thallium defects (that would have been identified as scar by stress-redistribution thallium imaging) were identified as viable on the basis of regional uptake of fluorine-18 (18F)-fluorodeoxyglucose (FDG).44–47 Although these data suggest that metabolic imaging with PET is superior to thallium imaging in the detection of viable myocardium, two limitations of these studies must be emphasized. First, the severity of the reduction in thallium activity within the irreversible thallium defects was not assessed. The importance of quantitative analysis of regional thallium activity is discussed later. Second, the previous comparative studies of thallium scintigraphy and PET all used postexercise thallium imaging followed by a single redistribution study 3 to 4 hours later. As noted earlier, the limitations of 4-hour redistribution imaging are well established.
Modifications in imaging protocols with 201Tl considerably enhance the ability of 201Tl imaging to detect viable myocardium.12,20,35 These include late redistribution imaging and 201Tl reinjection techniques.
Late Thallium-Redistribution Imaging
In many patients, late imaging at 24 to 72 hours elicits 201Tl redistribution in many defects that appear to be irreversible at 3 to 4 hours. This late 201Tl redistribution is evidence of viable myocardium.39,40,48,49 As many as 54% of defects that appear irreversible at 3 to 4 hours show reversibility at 24 hours, although this number is as low as 22% in some studies. 201Tl redistribution is a continual process,50 and a truly irreversible defect shown on early redistribution images will not reverse later. However, in many viable regions, defect reversal at 3 to 4 hours may be minimal and poorly detected. Hence, the defect may not reverse appreciably on qualitative interpretation, and the increase in relative tracer activity also may not exceed the reproducibility limit on quantitative analysis. In these regions, late redistribution imaging may confirm that defect reversibility has occurred.
Several studies show that late imaging at 8 to 72 hours shows substantial thallium redistribution in many defects that appear to be irreversible at 3 to 4 hours,39,40,48,49 and that this late thallium redistribution is consistent with viable myocardium.40,49 Kiat et al.40 reported in 21 patients undergoing myocardial revascularization procedures that 61% of apparently irreversible defects seen at 4 hours reversed at the time of late (18- to 72-hour) redistribution imaging. The revascularization results in this latter study, as assessed by postintervention repeat thallium imaging, provided additional important insights. Of all regions that appeared irreversible at 4 hours, 72% showed improvement after revascularization, confirming earlier studies that found that standard 3- to 4-hour thallium redistribution imaging overestimates the prevalence and severity of irreversible myocardial damage. However, when these 4-hour irreversible defects were further analyzed with the results of late imaging, 95% of regions with late redistribution improved after revascularization, compared with only 37% of regions that remained irreversible on late imaging.40 These findings indicate that myocardial segments that show late thallium redistribution represent viable myocardium and that late imaging may considerably improve the identification of viable myocardium in thallium defects that appear irreversible at 3 to 4 hours. The implications of late thallium redistribution for myocardial viability are similar to those of 3- to 4-hour redistribution. Many persistent defects seen at 3 to 4 hours show late redistribution. The positive predictive value of late redistribution is excellent, but the negative predictive value remains poor. The positive predictive value of late redistribution is more than 90%40 in predicting improvement after revascularization; thus, defect reversibility with late imaging is an excellent marker of viable myocardium. The negative predictive value of an irreversible defect at 24 hours appears to be only marginally better than that of an irreversible defect at 3 to 4 hours. For example, 37% of irreversible defects seen at 24 hours improve after revascularization,40 and 39% of irreversible defects seen at 24 hours show improvement by quantitative analysis when 201Tl is reinjected at rest.51 Moreover, nearly 50% of these defects are metabolically active on PET imaging.52 This finding suggests that some ischemic regions may never redistribute, even on late imaging, no matter how long the redistribution period, unless serum thallium levels are augmented.
Thallium-Reinjection Techniques
The reinjection of thallium at rest immediately after the standard 4-hour redistribution image may overcome several of these limitations and may be used to assess myocardial viability in apparently irreversible thallium defects on standard early or late-redistribution images (Fig. 37-4).53–55 Up to 49% of apparently irreversible defects on 3- to 4-hour redistribution images53 and 39% of these defects on 24-hour redistribution images51,56,57 show improved or normal uptake after thallium reinjection.53 Additional redistribution imaging obtained hours after the reinjected thallium dose appears to provide no information beyond that obtained by imaging immediately after reinjection.58 Fewer than 5% of myocardial regions with persistent defects on 3- to 4-hour redistribution plus reinjection images show evidence of late redistribution. However, late redistribution imaging is important when reinjection is performed at 4 hours without an intervening redistribution study. A simple exercise-reinjection protocol without redistribution imaging may miss unique and important viability information provided by redistribution. Hence, if reinjection protocols are used, it is essential to perform routine 3- to 4-hour redistribution imaging before the reinjection or to perform late-redistribution imaging in patients with persistent defects on stress-reinjection images.59
The observation that thallium uptake after reinjection represents viable myocardium is substantiated in three subgroups of patients. First, in nine studies43,53,60–66 reporting on 295 patients with LV dysfunction who were evaluated again 3 to 6 months after revascularization, improved wall motion occurred in 69% of segments identified as viable by thallium reinjection before revascularization. Such improvement occurred in only 11% of segments considered nonviable by redistribution or reinjection images (Fig. 37-5). Second, in comparative studies with thallium reinjection and PET imaging with FDG, most myocardial segments that were identified as viable by reinjection had metabolic evidence of myocardial viability.67–69 The concordance between data on thallium reinjection and FDG uptake was excellent, with 51% of regions with severe irreversible thallium defects on 4-hour redistribution studies identified as viable by both thallium reinjection and PET.67 Third, in patients who underwent gated MRI to assess regional systolic function, excellent correlation was observed between regional thallium activity and FDG activity in myocardial regions with severely reduced or absent wall thickening.68
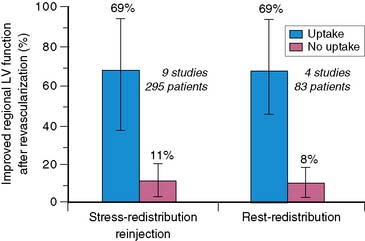
Figure 37-5 Likelihood of improved regional left ventricular (LV) function after revascularization based on thallium-201 single-photon emission tomography imaging. Data are summarized from nine studies done with stress-redistribution-reinjection imaging43,53,60–66 and four studies done with rest-redistribution imaging.71,73–75 The range of values reported in the individual studies is shown by the horizontal bars connected by vertical lines. The blue bars represent the positive predictive value, and the red bars represent the inverse of the negative predictive value.
These PET data are in keeping with previous studies indicating that up to 50% of regions with apparently irreversible thallium defects have metabolic activity by PET and thus evidence of viability.44–47 These data also indicate that thallium reinjection is a convenient, clinically accurate, and relatively inexpensive method with which to identify viable myocardium in patients with chronic CAD and LV dysfunction. Thus, thallium reinjection at rest after 3- to 4-hour redistribution imaging provides most of the clinically relevant information on myocardial viability in regions with apparently irreversible thallium defects. Thallium reinjection may be used instead of 24-hour imaging in most patients with a persistent thallium defect on conventional redistribution images.
Rest-Redistribution Thallium Imaging
The finding of exercise-induced ischemia in a patient with LV dysfunction has important prognostic implications that usually identify the patient as a candidate for revascularization therapy. Thus, exercise-redistribution-reinjection thallium protocols are attractive because they provide important information about both jeopardized myocardium and viable myocardium. However, in many patients, the sole clinical issue is the viability of one or more regions of dysfunctional LV myocardium, not whether there is also inducible ischemia. In others, the coronary anatomy is known to be at high risk, and stress testing is contraindicated, although the determination of viability may establish the potential benefit of revascularization. In these patients, rest-redistribution thallium imaging is a practical approach that can yield accurate viability data. It is essential to obtain both initial images (indicating regional perfusion) and subsequent redistribution images. Although early thallium studies yielded mixed results on the predictive accuracy of rest-redistribution imaging,33,36 subsequent studies show that a quantitative analysis of regional thallium activity in rest-redistribution studies predicts recovery of regional LV function with accuracy that is virtually identical to that achieved with thallium exercise-redistribution-reinjection imaging (see Fig. 37-5).56,70–75 These results are also comparable to those with PET imaging with FDG. PET achieves a higher positive predictive value, and thallium single-photon emission tomography (SPECT) imaging achieves a higher negative predictive value.
The available data comparing rest-redistribution imaging and stress-redistribution-reinjection imaging indicate comparable positive and negative predictive values for recovery of regional function after revascularization. Both techniques have high sensitivity, yielding a negative predictive value of approximately 90% (see Fig. 37-5). However, specificity is much lower, yielding a positive predictive value of less than 70%. A limitation of this analysis is that thallium data are considered binomially, rather than as a continuum.
In laboratories using dual-isotope imaging for stress/rest imaging, with a technetium-99m (99mTc) tracer for stress imaging and 201Tl for rest imaging, the rest-redistribution thallium technique for viability assessment can be easily incorporated into the evaluation of patients with LV dysfunction, as discussed subsequently in this chapter. This can be done by performing rest-redistribution thallium imaging before the 99mTc stress test (Fig. 37-6). Alternatively, if irreversible defects are detected after routine stress/rest imaging, late imaging of thallium redistribution at a time when the technetium has decayed provides additional information regarding viability of myocardium with reduced flow under resting conditions.
Quantitative Analysis of Thallium Data
In 12 of the 13 SPECT studies summarized in Figure 37-5, regional thallium activity was analyzed quantitatively rather than with a visual scoring system. It is unclear whether a subjective interpretation of images can replicate these results. However, in most cases, the criteria for viability in these quantitative studies were based on a threshold level of thallium activity such that a thallium level greater than 50% or 60% of the activity in normal myocardial segments was considered viable. This black-and-white approach to the thallium data, classifying myocardial areas simply as viable or nonviable, achieves reasonable results. However, it does not take advantage of one of the greatest strengths of perfusion imaging: the ability to view regional tracer activity as a continuum rather than a simple binary function. Several studies show the nearly linear relationship (Fig. 37-7) between regional thallium activity and the likelihood of recovery of regional function after revascularization.73–75 This continuous relationship between thallium activity and myocardial viability is confirmed by histologic studies of myocardial biopsy specimens obtained at surgery76 that show a significant inverse relationship between thallium uptake and the degree of myocardial interstitial fibrosis (Fig. 37-8). For example, all myocardial segments with thallium activity greater than 50% of normal were considered viable in the study of Perrone-Filardi et al.73 However, 56% of segments with thallium activity of 50% to 60% improved after revascularization, whereas 88% of segments with thallium activity greater than 80% showed functional improvement after revascularization (see Fig. 37-7). This important factor may explain the wide range of positive predictive values of thallium imaging reported in the individual studies summarized in Figure 37-5; there may have been considerable differences in relative thallium activity in regions considered viable in these studies. A corollary to this argument is that relative regional thallium activity provides a high degree of certainty at either end of the thallium-activity spectrum (see Fig. 37-7), but important uncertainty exists for intermediate thallium levels. Recent data suggest that late redistribution imaging (using a rest-redistribution protocol) may provide greater confidence about the potential for recovery of function in a myocardial region with intermediate thallium activity at 3 to 4 hours; 21% of such regions show increased relative thallium activity at 24 hours.74