1 Assessment of Cardiac Risk and the Cardiology Consultation
In the early 1980s, coronary artery bypass graft surgery (CABG) was characterized by operative mortality rates in the range of 1% to 2%. Over the ensuing years, however, urgent and emergent operations and “redo” procedures became common, and greater morbidity and mortality rates were observed. Percutaneous coronary interventions (PCIs) absorbed low-risk patients from the surgery pool, with the net result being that the operative mortality rate increased to the range of 5% to 6%. The trend toward PCI has continued, with recent trials demonstrating the safety of stenting even left main coronary artery disease (CAD).1 This demographic shift has led hospital administrators to ask for justification of the observed increase in CABG mortality. This often has prompted a time-consuming and expensive chart review to identify the differences in the patient populations that led to the greater morbidity. Even with this information, it was difficult to objectively determine the impact of these new and compelling factors on mortality. The impetus for the development of a risk-adjusted outcome assessment/appropriate risk adjustment scoring system was the need to compare adult cardiac surgery results in different institutions and to benchmark the observed complication rates.2 With the passage of healthcare reform, there is increased interest in publicly reporting perioperative outcomes, which requires optimal risk adjustment.
The first risk-scoring scheme for cardiac surgery was introduced by Paiement et al3 at the Montreal Heart Institute in 1983. Since then, multiple preoperative cardiac surgery risk indices have been developed. The patient characteristics that affected the probability of specific adverse outcomes were identified and weighed, and the resultant risk indices have been used to adjust for case-mix differences among surgeons and centers where performance profiles have been compiled. In addition to comparisons among centers, the preoperative cardiac risk indices have been used to counsel patients and their families in resource planning, in high-risk group identification for special care or research, to determine cost-effectiveness, to determine effectiveness of intervention, to improve provider practice, and to assess costs related to severity of disease.4,5
Sources of Perioperative Myocardial Injury in Cardiac Surgery
Myocardial injury, manifested as transient cardiac contractile dysfunction (“stunning”) or acute myocardial infarction (AMI), or both, is the most frequent complication after cardiac surgery and is the single-most important cause of hospital complications and death. Furthermore, patients who have a perioperative myocardial infarction (MI) have poor long-term prognosis; only 51% of such patients remain free from adverse cardiac events after 2 years, compared with 96% of patients without MI.6
Myocardial necrosis is the result of progressive pathologic ischemic changes that start to occur in the myocardium within minutes after the interruption of its blood flow, as seen in cardiac surgery (Box 1-1). The duration of the interruption of blood flow, either partial or complete, determines the extent of myocardial necrosis. This is consistent with the finding that both the duration of the period of aortic cross-clamping (AXC) and the duration of cardiopulmonary bypass (CPB) consistently have been shown to be the main determinants of postoperative outcomes in virtually all studies. This was further supported in a study with an average follow-up of 10 years after complex cardiac surgery in which Khuri7 observed a direct relation between the lowest mean myocardial pH recorded both during and after the period of AXC and long-term patient survival. Patients who experienced acidosis (pH < 6.5) had decreased survival compared with those who did not. Because myocardial acidosis reflects both myocardial ischemia and poor myocardial protection during CPB, this study demonstrated the relation of the adequacy of intraoperative myocardial protection to long-term outcome (see Chapters 3, 6, 18, and 28).
Reperfusion of an Ischemic Myocardium
Surgical interventions requiring interruption of blood flow to the heart must, out of necessity, be followed by restoration of perfusion. Numerous experimental studies have provided compelling evidence that reperfusion, although essential for tissue or organ survival, or both, is not without risk because of the extension of cell damage as a result of reperfusion itself. Myocardial ischemia of limited duration (< 20 minutes), followed by reperfusion, are accompanied by functional recovery without evidence of structural injury or biochemical evidence of tissue injury.8,9
Paradoxically, reperfusion of cardiac tissue, which has been subjected to an extended period of ischemia, results in a phenomenon known as myocardial reperfusion injury.10–12 Thus, a paradox exists in that tissue viability can be maintained only if reperfusion is instituted within a reasonable time period, but only at the risk for extending the injury beyond that caused by the ischemic insult itself. This is supported by the observation that ventricular fibrillation was prominent when the regionally ischemic canine heart was subjected to reperfusion.13 Jennings et al14 reported adverse structural and electrophysiologic changes associated with reperfusion of the ischemic canine heart, and Hearse15 introduced the concept of an oxygen paradox in noting cardiac muscle enzyme release and alterations in ultrastructure when isolated hearts were reoxygenated after a period of hypoxic perfusion.
Myocardial reperfusion injury is defined as the death of myocytes, alive at the time of reperfusion, as a direct result of one or more events initiated by reperfusion. Myocardial cell damage results from the restoration of blood flow to the previously ischemic heart, thereby extending the region of irreversible injury beyond that caused by the ischemic insult alone. The cellular damage that results from reperfusion can be reversible or irreversible, depending on the length of the ischemic insult. If reperfusion is initiated within 20 minutes after the onset of ischemia, the resulting myocardial injury is reversible and is characterized functionally by depressed myocardial contractility, which eventually recovers completely. Myocardial tissue necrosis is not detectable in the previously ischemic region, although functional impairment of contractility may persist for a variable period, a phenomenon known as myocardial stunning. Initiating reperfusion after a duration of ischemia of longer than 20 minutes, however, results in irreversible myocardial injury or cellular necrosis. The extent of tissue necrosis that develops during reperfusion is directly related to the duration of the ischemic event. Tissue necrosis originates in the subendocardial regions of the ischemic myocardium and extends to the subepicardial regions of the area at risk, often referred to as the wavefront phenomenon. The cell death that occurs during reperfusion can be characterized microscopically by explosive swelling, which includes disruption of the tissue lattice, contraction bands, mitochondrial swelling, and calcium phosphate deposits within mitochondria.13
The magnitude of reperfusion injury is directly related to the magnitude of the ischemic injury that precedes it. In its most severe form, it manifests in a “no-reflow” phenomenon. In cardiac surgery, prevention of myocardial injury after the release of the AXC, including the prevention of no reflow, is directly dependent on the adequacy of myocardial protection during the period of aortic clamping. The combination of ischemic and reperfusion injury is probably the most frequent and serious type of injury that leads to poor outcomes in cardiac surgery today (see Chapters 2, 3, 6, 12 to 14, 18, and 28).
Basic science investigations (in mouse, human, and porcine hearts) have implicated acidosis as a primary trigger of apoptosis. Acidosis, reoxygenation, and reperfusion, but not hypoxia (or ischemia) alone, are strong stimuli for programmed cell death, as well as the demonstration that cardiac apoptosis can lead to heart failure.16,17 This suggests that apoptotic changes might be triggered in the course of a cardiac operation, thus effecting an injurious cascade of adverse clinical events that manifest late in the postoperative course.
Adverse Systemic Effects of Cardiopulmonary Bypass
In addition to the effects of disruption and restoration of myocardial blood flow, cardiac morbidity may result from many of the components used to perform cardiovascular operations, which lead to systemic insults that result from CPB circuit-induced contact activation. Inflammation in cardiac surgical patients is produced by complex humoral and cellular interactions, including activation, generation, or expression of thrombin, complement, cytokines, neutrophils, adhesion molecules, mast cells, and multiple inflammatory mediators.18 Because of the redundancy of the inflammatory cascades, profound amplification occurs to produce multiorgan system dysfunction that can manifest as coagulopathy, respiratory failure, myocardial dysfunction, renal insufficiency, and neurocognitive defects. Coagulation and inflammation also are linked closely through networks of both humoral and cellular components, including proteases of the clotting and fibrinolytic cascades, as well as tissue factor. Vascular endothelial cells mediate inflammation and the cross-talk between coagulation and inflammation. Surgery alone activates specific hemostatic responses, activation of immune mechanisms, and inflammatory responses mediated by the release of various cytokines and chemokines (see Chapters 8 and 28 to 31). This complex inflammatory reaction can lead to death from nonischemic causes and suggests that preoperative risk factors may not predict morbidity. The ability to risk-adjust populations is critical to study interventions that may influence these responses to CPB.
Assessment of Perioperative Myocardial Injury in Cardiac Surgery
Unfortunately, the current clinical armamentarium is devoid of a means by which perioperative cardiac injury can be reliably monitored in real time, leading to the use of indicators of AMI after the event occurs. Generally, there is a lack of consensus regarding how to measure myocardial injury in cardiac surgery because of the continuum of cardiac injury. Electrocardiographic (ECG) changes, biomarker elevations, and measures of cardiac function have all been used, but all assessment modalities are affected by the direct myocardial trauma of surgery. The American College of Cardiology/European Society of Cardiology (ACC/ESC) published a definition of AMI in 2000, which includes a characteristic rise and fall in blood concentrations of cardiac troponins or creatine kinase (CK)-MB, or both, in the context of a coronary intervention, whereas other modalities are less sensitive and specific (Figure 1-1).19 Subsequently, the Joint ESC/ACCF/American Heart Association/World Heart Federation Task Force’s Universal Definition of Myocardial Infarction published a new “Universal Definition of Myocardial Infarction” in 2007.20 Any of the following criteria meet the diagnosis for MI: Detection of rise/fall of cardiac biomarkers (preferably troponin) with at least one value above the 99th percentile of the upper reference limit (URL), together with evidence of myocardial ischemia with at least one of the following: symptoms of ischemia, ECG changes indicative of new ischemia (new ST-T changes or new left bundle branch block), development of pathologic Q waves in the ECG, or imaging evidence of new loss of viable myocardium or new regional wall motion abnormality (RWMA).
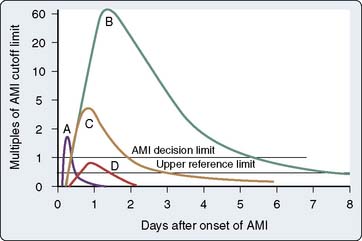
Figure 1-1 Timing of release of various biomarkers after acute ischemic myocardial infarction.
(From Apple FS, Gibler WB: National Academy of Clinical Biochemistry Standards of Laboratory Practice: Recommendations for the use of cardiac markers in coronary artery disease. Clin Chem 45:1104, 1999.)
Traditionally, AMI was determined electrocardiographically (see Chapters 15 and 18). Biochemical measures have not been widely accepted because exact thresholds for myocardial injury have not been clearly defined. Cardiac biomarkers are increased after surgery and can be used for postoperative risk stratification, in addition to being used to diagnose acute morbidity (Box 1-2).
Assessment of Cardiac Function
RWMAs follow the onset of ischemia in 10 to 15 seconds. Echocardiography can, therefore, be a sensitive and rapid monitor for cardiac ischemia/injury.21 If the RWMA is irreversible, this indicates irreversible myocardial necrosis (see Chapters 11 through 14). The importance of TEE assessment of cardiac function is further enhanced by its value as a predictor of long-term survival.22 In patients undergoing CABG, a postoperative decrease in left ventricular ejection fraction (LVEF) compared with preoperative baseline predicts decreased long-term survival.23
The use of TEE is complicated because myocardial stunning (postischemic transient ventricular dysfunction) is a common cause of new postoperative RWMAs, which are transient. However, the appearance of a new ventricular RWMA in the postoperative period, whether caused by irreversible AMI or by reversible myocardial stunning, is an indication of some form of inadequate myocardial protection during the intraoperative period and, therefore, of interest for the assessment of new interventions. Echocardiographic and Doppler systems also have the limitation of being sensitive to alterations in loading conditions, similar to the need for inotropic support and CO determinations.24 The interpretation of TEE images is also operator dependent.25 In addition, there are nonischemic causes of RWMAs, such as conduction abnormalities, ventricular pacing, and myocarditis, which confound the use of this outcome measure for the assessment of ischemic morbidity.
Electrocardiography Monitoring
The presence of new persistent Q waves of at least 0.03-second duration, broadening of preexisting Q waves, or new QS deflections on the postoperative ECG have been considered evidence of perioperative AMI.26 However, new Q waves also may be caused by unmasking of an old MI and therefore not indicative of a new AMI. Crescenzi et al27 demonstrated that the association of a new Q wave and high levels of biomarkers was strongly associated with postoperative cardiac events, whereas the isolated appearance of a new Q wave had no impact on the postoperative cardiac outcome. In addition, new Q waves may actually disappear over time.28 Signs of non–Q-wave MI, such as ST-T wave changes, are even less reliable signs of AMI after cardiac surgery in the absence of biochemical evidence. ST-segment changes are even less specific for perioperative MI because they can be caused by changes in body position, hypothermia, transient conduction abnormalities, and electrolyte imbalances (see Chapter 15).
Serum Biochemical Markers to Detect Myocardial Injury
Serum biomarkers have become the primary means of assessing the presence and extent of AMI after cardiac surgery. Serum biomarkers that are indicative of myocardial damage include the following (with post-insult peak time given in parentheses): myoglobin (4 hours), total CK (16 hours), CK-MB isoenzyme (24 hours), troponins I and T (24 hours), and lactate dehydrogenase (LDH) (76 hours). The CK-MB isoenzyme has been used most widely, but studies have suggested that troponin I is the most sensitive and specific in depicting myocardial ischemia and infarction.29–34
With respect to CK-MB, the definition of an optimal cutoff has been defined best by the correlation of multiples of the upper limit of normal (ULN) for the laboratory and medium- and long-term outcomes. For example, Klatte et al35 reported on the implications of CK-MB in 2918 high-risk CABG patients enrolled in a clinical trial of an anti-ischemic agent. The unadjusted 6-month mortality rates were 3.4%, 5.8%, 7.8%, and 20.2% for patients with a postoperative peak CK-MB ratio (peak CK-MB value/ULN for laboratory test) of less than 5, ≥5 to <10, ≥10 to < 20, and ≥20 ULN, respectively.35 The relation remained statistically significant after adjustment for ejection fraction (EF), congestive heart failure (CHF), cerebrovascular disease, peripheral vascular disease, cardiac arrhythmias, and the method of cardioplegia delivery. In the Arterial Revascularization Therapies Study (ARTS), 496 patients with multivessel CAD undergoing CABG were evaluated by CK-MB testing and followed after surgery at 30 days and 1 year.36 Patients with increased cardiac enzyme levels after CABG were at increased risk for both death and repeat AMI within the first 30 days. CK-MB increase also was independently related to late adverse outcome.
Studies suggest that postcardiac surgery monitoring of troponins can be used to assess myocardial injury and risk stratification. Increased cardiac-specific troponin I or T in patients after CABG has been associated with a cardiac cause of death and with major postoperative complications within 2 years after CABG.37,38 The ACC/ESC definition includes biomarkers but does not include specific criteria for diagnosing post-CABG AMI using cardiac biomarkers.19
There are a few new biomarkers of perioperative cardiac injury or ischemia under development. Brain natriuretic peptide (BNP) could be detected in the early stages of ischemia and decreases shortly after ischemic insult, allowing better detection of reinjury.39 BNP concentrations after CABG in the patients who had cardiac events within 2 years were significantly greater than those in the patients free of cardiac events.40 Soluble CD40 ligand (sCD40L) is another early biomarker of myocardial ischemia,41 and CPB causes an increase in the concentration of plasma sCD40L. A corresponding decrease in platelet CD40L suggests that this prothrombotic and proinflammatory protein was derived primarily from platelets and may contribute to the thrombotic and inflammatory complications associated with CPB.42 Future research will be required to determine how these biomarkers will be used to assess outcome after cardiac surgery.
Variability in Diagnosis of Perioperative Myocardial Infarction
The variability in diagnosing perioperative AMI has been studied by Jain and colleagues,43 who evaluated data from 566 patients at 20 clinical sites, collected as part of a clinical trial. The occurrence of AMI by Q-wave, CK-MB, or autopsy criteria was determined. Of the 25% of patients who met the Q-wave, CK-MB, or autopsy criteria for AMI, 19% had increased CK-MB concentrations, as well as ECG changes. Q-wave and CK-MB or autopsy criteria for AMI were met by 4% of patients. Multicenter data collection showed a substantial variation in the incidence of AMI and an overall incidence rate of up to 25%. The definition of perioperative AMI was highly variable depending on the definitions used.
Cardiac Risk Assessment and Cardiac Risk Stratification Models
In defining important risk factors and developing risk indices, each of the studies has used different primary outcomes. Postoperative mortality remains the most definitive outcome that is reflective of patient injury in the perioperative period. It is important to note that death can be cardiac and noncardiac, and if cardiac, may be ischemic or nonischemic in origin. Postoperative mortality rate is reported as either in-hospital or 30-day rate. The latter represents a more standardized definition, although more difficult to capture because of the cost-cutting push to discharge patients early after surgery. The value of developing risk-adjusted postoperative mortality models is the assessment of the comparative efficacy of various techniques in preventing myocardial damage, but it does not provide information that is useful in preventing the injury in real time.44 The postoperative mortality rate also has been used as a comparative measure of quality of cardiac surgical care.45,46
Postoperative morbidity includes AMI and reversible events such as CHF and need for inotropic support. The problems of using AMI as an outcome of interest were described earlier. Because resource utilization has become such an important financial consideration for hospitals, length of intensive care unit (ICU) stay increasingly has been used in the development of risk indices (see Chapter 33).
Predictors of Postoperative Morbidity and Mortality
Clinical and angiographic predictors of operative mortality were initially defined from the Coronary Artery Surgery Study (CASS).47,48 A total of 6630 patients underwent isolated CABG between 1975 and 1978. Women had a significantly greater mortality rate than men; mortality increased with advancing age in men, but this was not a significant factor in women. Increasing severity of angina, manifestations of heart failure, and number and extent of coronary artery stenoses all correlated with greater mortality, whereas EF was not a predictor. Urgency of surgery was a strong predictor of outcome, with those patients requiring emergency surgery in the presence of a 90% left main coronary artery stenosis sustaining a 40% mortality rate.
A risk-scoring scheme for cardiac surgery (CABG and valve) was introduced by Paiement et al3 at the Montreal Heart Institute in 1983. Eight risk factors were identified: (1) poor left ventricular (LV) function, (2) CHF, (3) unstable angina or recent (within 6 weeks) MI, (4) age greater than 65 years, (5) severe obesity (body mass index > 30 kg/m2), (6) reoperation, (7) emergency surgery, and (8) other significant or uncontrolled systemic disturbances. Three classifications were identified: patients with none of these factors (normal), those presenting with one risk factor (increased risk), and those with more than one factor (high risk). In a study of 500 consecutive cardiac surgical patients, it was found that operative mortality increased with increasing risk (confirming their scoring system).
One of the most commonly used scoring systems for CABG was developed by Parsonnet and colleagues (Table 1-1).49 Fourteen risk factors were identified for in-hospital or 30-day mortality after univariate regression analysis of 3500 consecutive operations. An additive model was constructed and prospectively evaluated in 1332 cardiac procedures. Five categories of risk were identified with increasing mortality rates, complication rates, and length of stay at the Newark Beth Israel Medical Center. The Parsonnet Index frequently is used as a benchmark for comparison among institutions. However, the Parsonnet model was created earlier than the other models and may not be representative of the current practice of CABG. During the period after publication of the Parsonnet model, numerous technical advances now in routine use have diminished CABG mortality rates.
Risk Factor | Assigned Weight |
---|---|
Female sex | 1 |
Morbid obesity (≥ 1.5 × ideal weight) | 3 |
Diabetes (unspecified type) | 3 |
Hypertension (systolic BP > 140 mm Hg) | 3 |
Ejection fraction (%): | |
Good > 50) | 0 |
Fair (30–49) | 2 |
Poor (< 30) | 4 |
Age (yr): | |
70–74 | 7 |
75–79 | 12 |
≥ 80 | 20 |
Reoperation | |
First | 5 |
Second | 10 |
Preoperative IABP | 2 |
Left ventricular aneurysm | 5 |
Emergency surgery after PTCA or catheterization complications | 10 |
Dialysis dependency (PD or Hemo) | 10 |
Catastrophic states (e.g., acute structural defect, cardiogenic shock, acute renal failure)* | 10–50† |
Other rare circumstances (e.g., paraplegia, pacemaker dependency, congenital HD in adult, severe asthma)* | 2–10† |
Valve surgery | |
Mitral | 5 |
PA pressure ≥ 60 mm Hg | 8 |
Aortic | 5 |
Pressure gradient > 120 mm Hg | 7 |
CABG at the time of valve surgery | 2 |
BP, blood pressure; CABG, coronary artery bypass graft; HD, heart disease; Hemo, hemodialysis; IABP, intra-aortic balloon pump; PA, pulmonary artery; PD, peritoneal dialysis; PTCA, percutaneous transluminal coronary angioplasty.
* On the actual worksheet, these risk factors require justification.
† Values were predictive of increased risk for operative mortality in univariate analysis.
From Parsonnet V, Dean D, Bernstein A: A method of uniform stratification of risk for evaluating the results of surgery in acquired adult heart disease. Circulation 79:I3, 1989, by permission.
Bernstein and Parsonnet50 simplified the risk-adjusted scoring system in 2000 to provide a handy tool in preoperative discussions with patients and their families, and for preoperative risk stratification calculation. The authors developed a logistic regression model in which 47 potential risk factors were considered, and a method requiring only simple addition and graphic interpretation was designed for relatively easily approximating the estimated risk. The final estimates provided by the simplified model correlated well with the observed mortality (Figure 1-2).
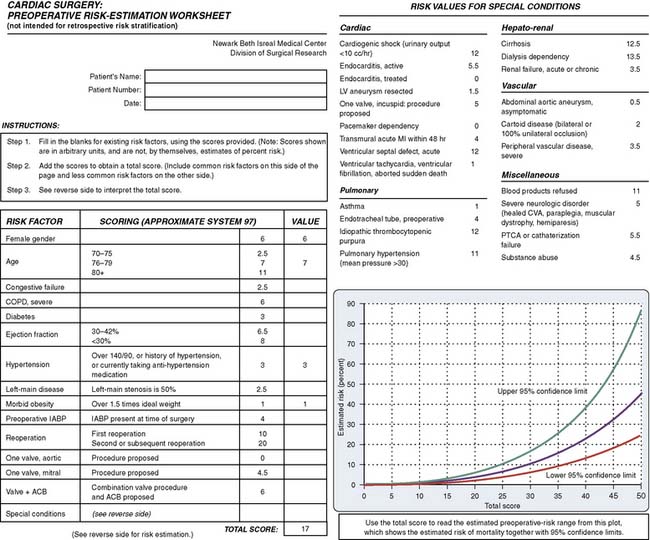
Figure 1-2 Preoperative Risk-Estimation Worksheet.
(From Bernstein AD, Parsonnet V: Bedside estimation of risk as an aid for decision-making in cardiac surgery. Ann Thorac Surg 69:823, 2000, by permission from the Society of Thoracic Surgeons.)
O’Connor et al51 used data collected from 3055 patients undergoing isolated CABG at five clinical centers between 1987 and 1989 to develop a multivariate numerical score. A regression model was developed in a training set and subsequently validated in a test set. Independent predictors of in-hospital mortality included patient age, body surface area, comorbidity score, prior CABG, EF, LV end-diastolic pressure, and priority of surgery. The validated multivariate prediction rule was robust in predicting the in-hospital mortality for an individual patient, and the authors proposed that it could be used to contrast observed and expected mortality rates for an institution or a particular clinician.
Higgins et al52 developed a Clinical Severity Score for CABG at The Cleveland Clinic. A multivariate logistic regression model to predict perioperative risk was developed in 5051 patients undergoing CABG between 1986 and 1988, and subsequently validated in a cohort of 4069 patients. Independent predictors of in-hospital and 30-day mortality were emergency procedure, preoperative serum creatinine level of greater than 168 μmol/L, severe LV dysfunction, preoperative hematocrit of less than 34%, increasing age, chronic pulmonary disease, prior vascular surgery, reoperation, and mitral valve insufficiency. Predictors of morbidity (AMI and use of the intra-aortic balloon pump [IABP], mechanical ventilation for ≥3 days, neurologic deficit, oliguric or anuric renal failure, or serious infection) included diabetes mellitus, body weight of 65 kg or less, aortic stenosis, and cerebrovascular disease. Each independent predictor was assigned a weight or score, with increasing mortality and morbidity associated with an increasing total score.