27 Assessment and monitoring of the perianesthesia patient
Alveolar Artery Carbon Dioxide Differences: The difference between the PaCO2 and the ETCO2 level is referred to as the alveolar-arterial CO2 difference (a-ADCO2).
Alveolar Dead Space: Alveoli that do not participate in gas exchange because of lack of blood flow.
Anatomic Dead Space: Areas of the tracheobronchial tree not involved in gas exchange.
Capnography: Measurement of end-tidal carbon dioxide at the patient’s airway that allows continuous assessment of the adequacy of alveolar ventilation.
Dead Space Ventilation: Includes anatomic, alveolar, and physiologic (total) dead space.
End-Tidal Carbon Dioxide (ETCO2): At the end of exhalation, the peak carbon dioxide occurs, which in the normal lung is the best approximation of alveolar carbon dioxide levels.
Flow-Directed Pulmonary Artery Catheter (FDPAC): Pulmonary artery thermodilution catheter used in hemodynamic monitoring.
Hemodynamic Monitoring (Invasive Monitoring): The monitoring of blood flow through the use of invasive catheters to provide pressure measurements in the systemic and pulmonary circulations, central veins, pulmonary capillary bed, and the right or left atrium, as well as cardiac output.
Hyperthermia: A core temperature greater than 38° C (100.4° F).
Hypothermia: A core temperature less than 36° C (96.8° F).
Left Atrial Pressure: Measured with a catheter placed directly in the left atrium. Usually monitored only in open heart cases when direct access to the left atrium can be reached. In the absence of mitral valve disease or left atrial tumor, left atrial pressure reflects left ventricular end-diastolic pressure and left ventricle preload.
Obstructive Sleep Apnea: Repeated episodes of obstructive apnea during sleep together with daytime sleepiness, mood changes, and altered function.1
Physiologic Dead Space: The sum of anatomic and alveolar dead space.
Pulmonary Artery Pressure: Pressure in the pulmonary artery.
Pulmonary Capillary Wedge Pressure (PCWP): Also known as the pulmonary capillary occlusive pressure; reflects the pressure in the left atrium.
Pulmonary Vascular Resistance: The resistance, impedance, or pressure that the right ventricle must overcome to eject the blood into the pulmonary artery.
Pulse Oximetry: Pulse oximetry (SpO2) is used for noninvasive measurement of arterial oxygen saturation (SaO2) in the blood.
Right Atrial Pressure: Reflects venous return to the right side of the heart and right ventricular end diastolic pressure (preload).
Systemic Vascular Resistance: The resistance, impedance, or pressure the left ventricle must overcome to eject the blood from the left ventricle.
Temporal Artery Temperatures: Scanning of the forehead over the temporal artery with a noninvasive thermometer.
The primary purpose of the postanesthesia care unit (PACU) is the critical evaluation and stabilization of patients after procedures, with an emphasis on the anticipation and prevention of complications that result from anesthesia or the operative or interventional procedure. A knowledgeable, skillful perianesthesia nurse must fully assess the condition of each patient not only at admission and at discharge but also at frequent intervals throughout the postanesthesia period. Assessment must be a continuous and complete process that leads to sound nursing judgment and the implementation of therapeutic care. Assessment includes the gathering of information from direct observation of the patient (the primary source), from the physician, other health care personnel, and from the medical record and the care plan. Traditionally perianesthesia nurses have, with only limited information, performed the role of caring for the surgical and interventional patients in the vulnerable postanesthesia state. However, for assessment of the perianesthesia patient and plan and implementation of appropriate care, preoperative information must be available as a basis for comparison with postoperative data. The perianesthesia nurse has a professional obligation to consider the patient’s history, clinical status, and psychosocial state. The necessary data may be gathered with chart review, personal preoperative visit, and consultation with other health care members who provide care to the patient. The collection of such information should be a coordinated effort with all involved members of the health care team. This chapter discusses the assessment of postprocedure patients and their common needs. Specific assessments related to patient age, the type of procedure, and problems that result from complicated diagnoses are addressed in following chapters. The assessment and management of postoperative pain is presented in Chapter 31.
Preoperative assessments
The preoperative evaluation of both the physical and the emotional status of the surgical patient is extremely important, and nursing brings a unique perspective to this assessment. The scope of perianesthesia nursing practice involves the age-specific assessment, diagnosis, intervention, and evaluation of patients within the perianesthesia continuum. The scope identifies risks for problems that can result from the administration of sedation/analgesia or anesthetic agents for surgical, diagnostic, or therapeutic procedures.2 Nurses in a number of subspecialties, including perianesthesia nurses, perioperative nurses, and general unit nurses, have advocated this assessment. A preoperative visit from each nurse who will care for the patient seems redundant and can be overwhelming for the patient. More appropriately, nurses should treat each other as colleagues who communicate needs for specific information, coordinate the collection of such information, and document data to be used for planning care. Multidisciplinary communications are instrumental in the education of all those who care for the patient and in the development of communication patterns.
Admission observations
Physical assessment of the perianesthesia patient must begin immediately on admission to the PACU. The patient is accompanied from the procedure room to the PACU by the anesthesia provider or monitoring nurse, who reports to the receiving nurse on the patient’s general condition, the procedure performed, and the type of anesthesia or sedation used. In addition, the nurse should be informed of any problems or complications encountered during the procedure and anesthesia or sedation (see Chapter 26). Because all anesthetics are depressants, postoperative assessment and care generally are the same, regardless of the specific agent used. For special precautions required for certain agents, review the chapters on anesthesia (see Chapters 19 through 25).
Respiratory function
Respiratory assessment is coupled with the related responses of the cardiovascular and neurologic systems for total evaluation of the adequacy of gas exchange and ventilatory efficiency. Respiratory function is evaluated with clinical assessment. Pulse oximetry is used for assessment of arterial oxygenation, and capnography is used in evaluation of the adequacy of alveolar ventilation. Arterial blood gas measurements may be a part of the respiratory assessment (see Chapters 12, 29, and 30).
Clinical assessment
Inspection
The resting respiratory rate of a normal adult is approximately 12 to 20 beats/min. Infants and children have a higher respiratory rate and a lower tidal volume than adults (see Chapter 49). Respirations should be quiet and easy and have a regular rate and rhythm. The chest should move freely as a unit, and expansion should be equal bilaterally. Alterations in symmetry can be caused by many factors, including pain, that may cause splinting at the incision site, consolidation, and pneumothorax. The nurse should note the character of the respirations; intercostal retractions, bulging, nasal flaring, or use of the accessory respiratory muscles, which are signs of respiratory distress. The depth of respiration is as important as the rate. Shallow respirations are the cardinal sign of continuing depression from anesthesia or preoperative medications, but can be caused by many other factors, including incisional pain, obesity, tight binders, and dressings that restrict movements of the thoracic cage or abdomen. Shallow respirations and use of the neck and diaphragmatic muscles may also indicate reparalyzation from the use of skeletal muscle relaxants such as succinylcholine, atracurium, pancuronium, and vecuronium. The presence of chest movements alone does not provide evidence that adequate gas exchange is occurring.
Listening and auscultation
First, the perianesthesia nurse should listen unaided to the patient’s respirations. Normal respiration should be quiet; noisy breathing indicates a problem. Extraneous sounds always indicate some kind of obstruction; however, quiet breathing does not always indicate the absence of problems. An accumulation of mucus or other secretions, evidenced by gurgling in any of the respiratory passages, can cause airway obstruction and should be removed immediately. Purposeful coughing with good expiratory airflow is the most effective method of clearing secretions. If the patient is not yet reactive enough to do this alone, the secretions must be suctioned orally and nasally. Nasotracheal suctioning may be useful to clear secretions and to stimulate cough, but the catheter is ineffective for reaching secretions distal to the carina. Obstruction can also occur from poor oropharyngeal muscle tone caused by the muscle-relaxant effect of general anesthesia plus the rolling back of the tongue. Patients with obstructive sleep apnea are prone to airway obstruction and should not undergo extubation until they are fully awake. Tracheal extubation should be performed only when the patient is breathing spontaneously with adequate tidal volumes, oxygenation, and ventilation.1,3 To relieve airway obstruction, use the jaw thrust maneuver by providing anterior pressure support on the angle of the jaw to open the air passages.
Monitoring of oxygenation with pulse oximetry
A pulse oximeter is used for noninvasive measurement of arterial oxygen saturation (SaO2) in the blood (SpO2 when measured with pulse oximetry) and is a valuable adjunct to the clinical assessment of oxygenation. Many clinical indicators, such as the patient’s color and the characteristics of the respirations, are subjective, and the physical signs of cyanosis are not evident until hypoxia is severe. Pulse oximetry monitoring is objective and continuous and provides an early warning of developing hypoxemia, thus allowing intervention before signs of hypoxia appear. Consequently, pulse oximetry has been widely adopted in the PACU as a tool for both safety monitoring and patient management. As a confirmation of the importance of pulse oximetry, the American Society of PeriAnesthesia Nurses (ASPAN) PeriAnesthesia Nursing Standards and Practice Recommendations 2010-2012 recommends evaluation of all PACU patients with pulse oximetry at admission and discharge, and ASPAN recommends a pulse oximeter for every patient in all phases of perianesthesia nursing levels of care.2
A pulse oximeter consists of a microprocessor-based monitor and a sensor (Fig. 27-1). In addition to a SpO2 display, most oximeters display the pulse rate and have an adjustable alarm system that sounds when values register outside a designated range. A variety of sensors is available, each intended for application to specific sites and for use on patients of various sizes (the manufacturer’s instructions describe these requirements). The sensor is applied to a site with a good arterial supply. The most common application site is a finger or toe (hand or foot in neonates); other sites include the nose, the forehead, the earlobe, or the temple. Both reusable sensors and disposable adhesive sensors are available, and disposable sensors allow for patient-dedicated monitoring when infection control concerns are present.
Technology overview
Interpretation of pulse oximetry measurements
Consideration of the mechanisms of oxygen transport is essential for adequate interpretation of SpO2. Approximately 98% of the oxygen in blood is bound to hemoglobin; SaO2 and SpO2 reflect this blood oxygen. The remaining blood oxygen is dissolved in plasma; blood gas analysis measures the partial pressure exerted by this oxygen dissolved in plasma (PaO2 = 80 – 100 mm Hg at sea level). The dissolved oxygen is used to meet immediate metabolic needs. The oxygen bound to hemoglobin serves as the reservoir that replenishes the pool of dissolved oxygen (see Chapter 12).
The rate at which oxygen binds to hemoglobin is primarily controlled by two factors: the PaO2 and the affinity of hemoglobin for oxygen. This relationship between SaO2 and PaO2 is represented by the oxyhemoglobin dissociation curve. The curve is sigmoid in shape, and its position is affected by a number of physiologic variables that change the affinity of hemoglobin for oxygen (Fig. 27-2).
Clinical issues
As with any technology, important clinical issues must be considered for appropriate use of pulse oximetry. Shifts in the oxyhemoglobin dissociation curve that are caused by abnormal values of pH, temperature, partial pressure of carbon dioxide (PCO2), and 2,3-diphosphoglycerate must be considered. Consideration of the patient’s hemoglobin level is also important because a pulse oximeter cannot detect depletion in the total amount of hemoglobin. When pulse oximetry is used on a postoperative patient with a low hemoglobin level, a high SpO2 value might not reflect adequate oxygenation. The amount of hemoglobin, although it is well saturated with oxygen, may be inadequate to meet tissue needs because fewer carriers are available to transport oxygen.
Patient movement can produce false signals that interfere with the ability of the pulse oximeters to identify the true pulse, thus leading to unreliable SpO2 and pulse rate readings. The sensor should be properly and securely applied; a sensor that is loosely attached or incorrectly positioned can magnify the effect of motion. If the problem persists, consideration should be given to moving the sensor to a less active site. Pulse oximeters that use the electrocardiographic signal as an aid in identification of the pulse can have an enhanced ability to distinguish between the true pulse and artifacts produced by motion. The result is more reliable SpO2 readings.
Monitoring of ventilation with capnography
Technology overview
Sidestream capnographs incorporate moisture-control features that are designed to minimize clogging of the sample tube, protect the measurement chamber from moisture-induced damage, and minimize the risk of cross contamination. The design of these moisture-control systems significantly affects a monitor’s ease of use. Most systems rely on water traps, which must be emptied routinely. A new technology uses a special system of filters and tubing to dehumidify the sample and thus eliminate the need for water traps.
The normal capnogram
For effective use of capnography, it is important to understand the components of the normal CO2 waveform (capnogram)—a square wave pattern with a plateau (Fig. 27-3). Early in exhalation, air from the anatomic dead space, which is virtually CO2 free, is measured with the instrument. As exhalation continues, alveolar gas reaches the sampling site, and the CO2 level increases rapidly. The CO2 concentration continues to increase throughout exhalation and reaches the alveolar plateau because alveolar gas dominates the sample. At the end of exhalation, the ETCO2 occurs, which in the normal lung is the best approximation of alveolar CO2 levels. The CO2 concentration then drops rapidly as the next inhalation of CO2-free gas begins.
End-tidal versus arterial carbon dioxide
In normal conditions, when ventilation and perfusion are well matched, ETCO2 closely approximates arterial CO2 (PaCO2). The difference between the PaCO2 and the ETCO2 level is the alveolar-arterial CO2 difference (a-ADCO2). ETCO2 is usually as much as 5 mm Hg lower than PaCO2. When the two measurements differ significantly, an anomaly in the patient’s physiology, the breathing circuit, or the capnograph is usually present. Significant divergence between ETCO2 and PaCO2 is often attributable to increased alveolar dead space. CO2-free gas from nonperfused alveoli mixes with gas from perfused regions, thus decreasing the ETCO2 measurement. Clinical conditions that cause increased dead space, such as pulmonary hypoperfusion, cardiac arrest, and embolic conditions (e.g., air, fat thrombus, amniotic fluid), can increase the a-ADCO2. Changes in the a-ADCO2 can be used in assessing the efficacy of the treatment; as the patient’s dead space improves, the partial pressure of the alveolar carbon dioxide less the partial pressure of arterial carbon dioxide (PACO2 – PaCO2) narrows. Increases in dead space ventilation lower ETCO2 and therefore increase the PaCO2 – ETCO2 gradient. Widened PaCO2 – ETCO2 examples include embolic phenomena, hypoperfusion, and chronic obstructive pulmonary disease. Alternatively, a significant PACO2 – PaCO2 value can indicate incomplete alveolar emptying (e.g., with reactive airway disease), a leak in the gas-sampling system that allows loss of respiratory gas, and contamination of respiratory gas with fresh gas.4
Interpretation of changes in the capnogram
A sudden decrease in ETCO2 to a near-zero level indicates that the monitor is no longer detecting CO2 in exhaled gases (Fig. 27-4). Immediate action is crucial for detection and correction of the cause of this loss of ventilation. Possible causes include a completely blocked endotracheal tube, esophageal intubation, a disconnection in the breathing circuit, and inadvertent extubation. The latter three possibilities are particularly likely if the decrease in ETCO2 coincides with movement of the patient’s head. First, after elimination of possible clinical causes for this decrease in ETCO2, a clogged sampling tube or instrument malfunction is investigated as the cause of the problem.
An exponential decrease in ETCO2 over a small number of breaths usually signals a life-threatening cardiopulmonary event that has dramatically increased dead space ventilation (Fig. 27-5). Sudden hypotension, pulmonary embolism, and circulatory arrest with continued ventilation must be considered.
A gradual increase in the ETCO2 level while the capnogram retains its normal shape usually indicates that ventilation is inadequate to eliminate the CO2 that is produced (Fig. 27-6). This situation can be the result of a small ventilator leak or a partial airway obstruction that reduces minute ventilation. It can also reflect increased CO2 production associated with increased body temperature, the onset of sepsis, or shivering. Of particular importance, a large increase in ETCO2 can be one of the earliest signs of malignant hyperthermia, which may not begin until after emergence from anesthesia.
A gradual decrease in the ETCO2 level commonly occurs in the patient who is anesthetized, narcotized, hyperventilated, or hypothermic (Fig. 27-7).
Assessment of the capnogram can reveal information about the quality of alveolar emptying. For example, the patient with bronchospasm is unable to completely empty the alveoli, and the resulting capnogram does not have an alveolar plateau (Fig. 27-8). The ETCO2 reported by the capnograph in this instance is not a good estimate of alveolar CO2. Effective administration of bronchodilator therapy commonly improves alveolar emptying and results in a more normal capnogram.
Clinical issues
In addition to the diagnostic usefulness of changes in the capnogram, some specific applications of capnography are particularly valuable in the PACU. Of primary importance is its ability to provide early warning of hypoventilation that, in the PACU, may be the result of anesthesia, sedation, analgesia, or pain. A falling ETCO2 value may indicate pulmonary hypoperfusion from blood loss or hypotension. During rewarming, ETCO2 values are likely to increase as metabolic activity increases.
Importantly in the PACU, capnography can provide critical information about the patient’s ventilatory status, including an early warning of apnea that results in overall patient safety.5 Deterioration of a patient is discernable 2 to 3 minutes earlier in a patient with an ETCO2 reading than with oxygen saturation. Therefore capnography is a helpful assessment for patients who are extremely sedated, who require high doses of opioids, or who have obstructive sleep apnea.6
The ASPAN does not currently have a practice recommendation requiring continuous monitoring of ETCO2 in the phase I PACU. Practice Recommendation 2, Components of Initial, Ongoing, and Discharge Assessment and Management, recommends that vital signs are monitored, to include “end-tidal CO2 (capnography) monitoring if available and indicated.”2,6
Cardiovascular function and perfusion
Blood pressure monitoring
Noninvasive measurement
Manual method
The cuff is inflated, and when cuff pressure exceeds the arterial pressure, arterial blood flow ceases and the pulse is no longer palpated. As pressure is released with turning the valve of the inflation bulb, blood flow resumes and audible (Korotkoff) sounds are noted with the stethoscope. These sounds change in quality and intensity throughout further cuff deflation and generally disappear. Systolic pressure is noted as the first audible sound in the cuff-deflating process.7 The diastolic pressure is marked by the disappearance of sounds in the adult patient and the muffling of sounds in the pediatric patient.
Automatic method
One of the most commonly used automatic noninvasive blood pressure methods is based on oscillometric technology. The cuff is chosen and applied according to conventional technique. Oscillations of the arterial wall are occluded as the cuff is inflated and are detected during cuff deflation. Systolic pressure is indicated at the onset of oscillations. As cuff pressure decreases, oscillations increase in amplitude and peak at the mean arterial pressure. The point at which oscillations disappear is the diastolic pressure (Fig. 27-9). All three pressures are normally reported on oscillometric monitoring devices.
Invasive measurement
Arterial blood pressure measurements are continuous and are indicated for patients at high risk hemodynamically. Changes in patient pressures can be observed on an ongoing basis. This technology may also be chosen for patients in whom indirect measurements fail because of diminished or absent Korotkoff sounds (i.e., patients who are obese or edematous) and for those with high peripheral vascular resistance. The direct arterial access is also beneficial if the patient needs frequent blood samples for laboratory analysis.
Damping of the arterial waveform with subsequent unreliable readings may occur for a variety of reasons, including clotting and kinking of the arterial catheter, positioning of the catheter against the arterial wall, and the presence of air bubbles within the arterial line system. Loose connections, calibration error, and equipment failure can also contribute to unreliable readings. A square-wave test evaluates the dynamic response of the pressure monitoring system. To perform a square-wave test, activate the fast flush device for 1 to 2 seconds and note the monitor configuration. The patient wave form will be replaced with a square wave (Fig. 27-10).
An Allen test should be performed before radial artery cannulation for minimization of the risk of hand ischemia (see Chapter 11). If arterial lines are discontinued in the PACU, constant pressure should be applied to the site for 5 to 10 minutes or until bleeding has ceased. A pressure dressing should be applied, and the site should be checked frequently for any bleeding and radial pulse palpation.
Complications and risks of invasive arterial blood pressure monitoring include infection, thrombosis, emboli, tissue ischemia, hemorrhage, and vessel perforation. Arterial blood pressure monitoring is generally contraindicated in patients with septicemia, coagulopathies, irradiated arterial sites, anatomic anomalies, inadequate collateral blood flow, or thrombosis. No intravenous solution or medications should be administered through the arterial pressure monitoring system at any time. If the catheter patency is in question, blood and fluid are aspirated from the blood drawing port or stopcock and the system is flushed with the fast flush device, not a syringe.8
Electrocardiographic monitoring
The perianesthesia nurse must have a basic understanding of cardiac monitoring and should be able to interpret the basic cardiac rhythms and dysrhythmias and correlate them with expected cardiac output and its effects on the patient’s condition. According to the most recent ASPAN standards, ECG monitoring should be performed for each patient during phase I level of care, and an ECG monitor should be readily available for patients in preanesthesia and phase II level of care areas.2 Dysrhythmias of any type can occur at any time and in any patient during the postoperative period; therefore accurate ECG monitoring and interpretation are mandatory skills for the perianesthesia nurse. This section is designed to provide an introduction to specific problems of cardiac monitoring in the PACU.
Lead placement
Site selection on the chest is based on a triangular arrangement of positive, negative, and ground electrodes. Placement of electrodes directly over the diaphragm, areas of auscultation, heavy bones, or large muscles is avoided. Adequate space for application of defibrillator paddles is allowed in the event that defibrillation should become necessary. Fig. 27-11 depicts the most commonly used electrode leads. Lead II is the most commonly used in the PACU because it is the most versatile; it is useful in assessing P waves, P-R intervals, and atrial dysrhythmias. The modified chest lead I is useful for assessing of bundle branch block and differentiation between ventricular dysrhythmias and aberrations. This lead is useful when the patient is known to have preexisting cardiac disease. The Lewis lead is useful when P waves are difficult to distinguish with other leads and is used to detect atrial flutter when it is suspected clinically but not demonstrated on the ECG.
Sinus dysrhythmias
Sinus bradycardia
Fig. 27-12 shows a slow heart rate, less than 60 beats/min. Sinus bradycardia is a rhythm with impulses that originate at the sinus node at a rate of less than 60 beats/min. Its rhythm may be irregular because of accompanying sinus dysrhythmias. All other complex features are normal.
Sinus bradycardia is commonly encountered in the PACU because of the depressant effects of anesthesia. It can occur normally during sleep, and young healthy adults, especially those who are normally physically active, often have bradycardia. Usually no treatment is necessary except continuation of the stir-up regimen. Excessive parasympathetic stimulation from pain may cause bradycardia, in which case appropriate analgesics and other pain-relieving measures should be initiated. If the patient shows symptoms of low cardiac output, notify the physician; treatment may be initiated with atropine to block vagal effects or epinephrine and dopamine to stimulate the cardiac pacemaker. If temporary pacing wires are available, either atrial or ventricular pacing can be attempted. Bradycardia in conjunction with hypotension is considered an ominous sign for a pediatric trauma patient in shock, because the major component of cardiac output in small children is the heart rate.9
Sinus tachycardia
Fig. 27-13 shows a fast heart rate (greater than 100 beats/min). The rhythm may be slightly irregular; all other complex features are normal.
Supraventricular dysrhythmias
Premature atrial contraction
Premature atrial contraction, or atrial premature beat, occurs earlier than expected as a result of an irritable focus in the atrium (Fig. 27-14). Cardiac rate and rhythm are normal except for the prematurity. The P wave configuration of the premature beat usually differs from that of the normal beat. The PAC is followed by a pause that is not fully compensatory. This dysrhythmia results from anxiety and is commonly encountered in the PACU. No treatment is necessary unless the PACs become frequent or the patient becomes symptomatic. Pharmacologic therapy may become necessary with agents such as digitalis, beta blockers, or verapamil if hemodynamic function is impaired.
Atrial tachycardia
Atrial tachycardia is a rhythm disturbance that is a rapid regular supraventricular heart rate that results from an irritable focus of five or more PACs in succession (Fig. 27-15). The rate is 150 to 200 beats/min with a regular rhythm.
Atrial flutter
Atrial flutter consists of rapid supraventricular contractions that result from an ectopic focus with varying degrees of ventricular blocking (Fig. 27-16). Its cause is the same as that of PAC and atrial tachycardia. The rhythm is usually regular; the atrial rate is 250 to 350 beats/min. Treatment is the same as for atrial tachycardia.
Atrial fibrillation
In atrial fibrillation, one or more irritable atrial foci discharge at an extremely rapid rate that lacks coordinated activity (Fig. 27-17). Atrial fibrillation occurs commonly in patients with atrial enlargement from mitral valve disease or from long-standing coronary artery disease and is often preceded by PACs, tachycardia, or flutter. Clinically, the patient has an irregular heartbeat, pulse rate, and usually, a noticeable pulse deficit. Cardiac output decreases in varying degrees. Normally, atrial filling and contraction account for 30% of ventricular filling. Without this atrial filling, or “atrial kick,” of volume into the ventricle, stroke volumes and thus cardiac outputs are diminished. Treatment involves digitalis, quinidine, verapamil, atrial pacing, ablation, or cardioversion.
Ventricular dysrhythmias
Premature ventricular contraction
Premature ventricular contraction is a rhythm disturbance that involves an earlier-than-expected ventricular contraction from an irritable focus in the ventricle (Fig. 27-18). The rhythm is regular except for the premature beat, and the rate is normal.
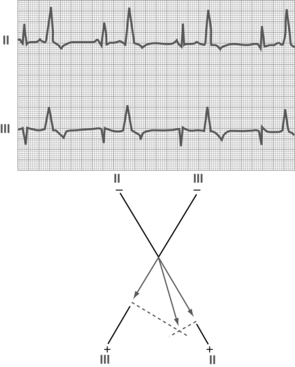
FIG. 27-18 Premature ventricular contractions (leads II and III).
(From Hall J: Guyton and Hall textbook of medical physiology, ed 12, Philadelphia, 2011, Saunders.)
The PVC is followed by a pause that is fully compensatory (i.e., the time of the PVC plus the pause time equals the time of two normal beats). PVCs are commonly encountered in the PACU and can occur in any patient. Occasional PVCs occur normally and need no treatment. Multiple PVCs may indicate inadequate oxygenation; when they occur, the patient’s respiratory status should be assessed thoroughly. Other causative factors of PVCs include electrolyte disturbances, acid-base imbalance, drug toxicity, and hypoxemia of the myocardium.
Ventricular tachycardia
Three or more consecutive PVCs constitute ventricular tachycardia (Fig. 27-19). The rhythm is fairly regular, and P waves are not seen. Occasionally, patients may have ventricular tachycardia and be asymptomatic, but usually they have anxiety, palpitations, fluttering, pounding in the chest, dizziness, faintness, and precordial pain. If ventricular tachycardia is prolonged, cyanosis, mental confusion, convulsions, and unconsciousness develop as a result of decreased blood and oxygen supply to the brain.
Ventricular fibrillation
Ventricular fibrillation is characterized by a rapid irregular quivering of the ventricles that is uncoordinated and incapable of pumping blood (Fig. 27-20). This rhythm disturbance is the major death-producing cardiac dysrhythmia. The immediate initial treatment is external direct current countershock (Fig. 27-21). Ventricular fibrillation may occur spontaneously without any forewarning, or it may be preceded by evidence of ventricular irritability. Patients in whom ventricular fibrillation is likely to develop include those with underlying heart disease, those with ventricular irritability in the operating room during surgery, and those with symptoms of shock. All these patients should be monitored continuously throughout the recovery period. If ventricular fibrillation is not immediately terminated with countershock, CPR and a cardiac code must be initiated without delay (see Chapter 57).
Hemodynamic monitoring
Although more prominent in cardiac surgery, hemodynamic monitoring using pulmonary artery catheters are commonly used with patients of higher acuity who receive care in many PACUs. Minimally invasive monitoring techniques include use of the esophageal Doppler, arterial pressure-based cardiac output, impedance cardiography, or ultrasound cardiac output monitoring. These minimally invasive techniques reflect cardiac output and assessment of fluid requirements by volumetric means.
Hemodynamic monitoring can be accomplished via the following invasive lines: a flow-directed pulmonary artery catheter, a central venous pressure catheter, a left-atrial or right-atrial catheter, a pulmonary artery thermistor catheter, or a peripheral arterial catheter (A-line). The parameters obtained from these various lines, the catheter insertion sites, and the placement and monitoring methods are presented in Table 27-1 and depicted in Fig. 27-22. Problems associated with maintenance of these lines are summarized in Table 27-2.
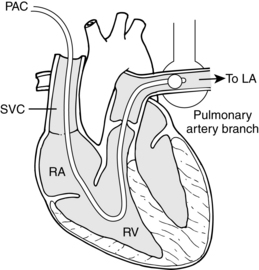
FIG. 27-22 Schematic view of anatomy of pulmonary catheterization.
(From Papadakos P, Szalados J: Critical care: the requisites in anesthesiology, St. Louis, 2005, Mosby.)
Table 27-2 Potential Problems Associated with Invasive Hemodynamic Monitoring
POTENTIAL PROBLEMS | ETIOLOGY | PRECAUTIONS AND TREATMENT |
---|---|---|
Alterations in Pressure Wave Configurations | ||
Dampened tracings | Technical | |
Air in system | Check system for bubbles; flush bubbles out of system. | |
Disconnection in system | Inspect and tighten all connections. Check that stopcocks are positioned correctly and covered with dead-end stopcock port covers (no holes). | |
Kinked catheter | Remove dressing to ascertain whether catheter is kinked externally. | |
Catheter tip against wall | Turn patient’s head or reposition extremity in which that catheter is inserted; watch for improvement in tracing. Gently aspirate catheter from various angles to determine at which angle best flow is achieved; tape and redress catheter at angle at which best flow is achieved. Gently flush catheter in an attempt to push tip away from vessel wall. Never flush catheter in which clotting is suspected. | |
Physiologic | ||
Clot on catheter tip | Attempt to aspirate blood from catheter. If possible, keep aspirating until clot is retrieved or blood no longer seems thickened, then flush system until line is cleared and readable tracing reappears. If blood cannot be aspirated, do not flush system and notify physician. | |
With FDPAC, this may also indicate that catheter has advanced forward and is in wedged position* | Ensure balloon is deflated. Recheck system and line. If no improvement, obtain chest radiograph and notify physician. | |
Technical | ||
Abrupt exaggeration of pressure tracings | Loss of calibration of level of transducer | Re-zero and re-level transducer. Check that balloon has not accidentally inflated. Check for dysrhythmia. |
Physiologic | ||
Slippage of catheter out of chamber or vessel | Avoid traction on intravascular lines; tape catheter to skin or secure with suture. | |
FDPAC slipping from pulmonary artery to right ventricle; characterized by systolic pressure that remains same while diastolic pressure falls into range of right-ventricular end-diastolic pressure | Inflate balloon in an attempt to let catheter float back into pulmonary artery. If catheter does not migrate back into pulmonary artery, obtain chest radiograph and notify physician. | |
RAC, PAC, or PATC has slipped out of vessel wall into thoracic cavity | Attempt to aspirate to see whether catheter is still in vessel. If blood returns, flush system and attempt to obtain readable pressure tracings. If no blood return is achieved, notify physician and remove catheter, per protocol. | |
Alterations in Vascular Integrity | ||
Venous and arterial spasms | Trauma to vessels during prolonged insertion attempts; artery irritated by catheter | Apply local anesthetic to catheter surface or administer anesthetic via intravenous route. Use guidewire to facilitate insertion. Cool catheter to make it less flexible and easier to insert. |
Thrombophlebitis | Irritation to vessels from prolonged insertion attempts or from constant motion of catheter against vessel | See Venous and Arterial Spasms, discussed previously. Secure catheter in place with either tape or suture. Avoid prolonged infusions of chemically irritating medications. Maintain adequate dilutions and observe for signs and symptoms of phlebitis and change flush solution bag before it empties. Notify physician for possible withdrawal of catheter. Distal placement of stopcocks, connecting catheters, and tubing permits atraumatic blood sampling and flushing. |
Embolization | Clot embolization from thrombophlebitis or from clot on catheter tip | Always aspirate catheter first if clot is suspected. Never flush. |
Pulmonary embolism with infarct from FDPAC | Observe for changes in chest radiograph that indicate pulmonary embolization. | |
Cerebral embolization from LAC | Observe for neurologic changes that may indicate embolization from LAC. | |
Peripheral embolization with extremity ischemia from peripheral arterial lines | Observe for ischemic changes of extremity in which catheter is located. | |
Air embolization | Loose connections | Secure and tighten all connections. Vigilantly observe LAC, because even minute amounts of air in this system can lead to serious neurologic complications. |
Rupture of balloon on FDPAC caused by overinflation | Inflate balloon slowly, and do not overinflate. Limit inflations. Allow balloon to empty air passively back into syringe. Avoid aspirating air back because this weakens integrity of balloon. Aspirate only if air fails to return passively. If air does not return and rupture is questioned, sterile saline solution can be injected into balloon and attempts made to aspirate it back. Failure to aspirate fluid back indicates leak; physician should be notified. | |
Vessel erosion or hemorrhage | Inadequate hemostasis after insertion | Apply firm pressure for 15 to 20 minutes. |
Bleeding from insertion site: Rupture of branch of pulmonary artery |
Do not attempt to inflate balloon if tracing already appears wedged. Inject only prescribed amount of air into balloon.
Inject air slowly, and stop injecting if resistance is felt. Inject only amount of air necessary to obtain wedge tracing.
Limit wedge intervals in patients at high risk, such as patients with pulmonary hypertension or long-standing mitral valve disease.
RAC, Right-atrial catheter; PATC, pulmonary artery thermistor catheter; LAC, left-atrial catheter. FDPAC, flow-directed pulmonary artery catheter; CVP, central venous pressure.
* Wedging of catheter in postoperative patient may be common occurrence for two reasons: (1) catheter may have advanced forward during operative procedure when chest was open and lungs were not fully inflated, because less resistance to forward advancement was found; and (2) as hypothermia is reversed and patient and catheter rewarm, its increased flexibility may allow it to float forward.
Adapted from Cole D, Schlunt M: Adult perioperative anesthesia: the requisites in anesthesiology, Philadelphia, 2004, Mosby; and Dennison R: Pass CCRN! ed 3, St. Louis, 2007, Mosby.
Right-atrial pressure
The normal right-atrial pressure ranges from 2 to 6 mm Hg. Pressures that exceed that level can be the result of fluid overload, right-ventricular failure, tricuspid valve abnormalities, pulmonary hypertension, constrictive pericarditis, or cardiac tamponade. Values in the lower range are usually indicative of hypovolemia.
Pulmonary capillary wedge pressure
Normal pulmonary capillary wedge pressure (PCWP) recordings are between 8 and 12 mm Hg. Values in this range can be caused by an increased volume load, as is seen in left-ventricular failure, or they can be created by an obstruction to forward flow. Such obstructions may be caused by mitral stenosis, mitral regurgitation, or pulmonary embolism. Lower values may result from hypovolemia or indicate an obstruction to left-ventricular filling, which could occur with a pulmonary embolism, pulmonary stenosis, or right-ventricular failure.
Cardiac output and cardiac index
in which SV is stroke volume, HR is heart rate, and CO is cardiac output.
Cardiac index is calculated with the following formula:
in which BSA is body surface area in square meters and CI is cardiac index. Normal CI ranges from 2.5 to 4 Lmin/m2. Because CI takes body size into consideration, it is a better indicator of the patient’s perfusion status.1,6,8,9
Systemic vascular resistance
in which MAP is mean arterial pressure and CVP is central venous pressure.1,8,10,11
Central nervous system function
Assessment of the CNS in the PACU generally involves only gross evaluation of behavior, level of consciousness, intellectual performance, and emotional status. A more detailed assessment of CNS function is necessary for patients who have undergone CNS surgery; that discussion occurs in Chapters 10 and 38.
Emergence from anesthesia
Patients arrive in the PACU at all levels of consciousness, from fully awake to completely anesthetized. With modern anesthesia techniques, however, most patients respond appropriately by the time they are established in the PACU and become oriented quickly when the stir-up regimen is begun (see Chapter 28). With the use of fluorinated and opioid anesthetics, emergence is generally quiet and uneventful. Occasionally, a patient becomes agitated and thrashes about; this situation seems to occur more often in adolescents and young adults than in patients of other age groups. Emergence delirium also tends to occur more frequently in patients who have undergone intraabdominal and intrathoracic procedures (see Emergence Excitement in Chapter 29).
Thermal balance
The measurement of the patient’s body temperature in the PACU is particularly important. The most recent ASPAN standards state that, at minimum, the preoperative assessment, initial postoperative physical assessment, and discharge evaluation of the patient in phases I and II Level of care should include documentation of temperature.2 Normal body temperature may vary from 36° to 38° C (96.8° to 100.4° F). In the healthy adult, body temperature remains fairly constant because of the balance between heat production and heat loss. Alterations in body temperature occur often in the postoperative patient. Factors that affect the body temperature in the PACU patient are listed in Box 27-1.
BOX 27-1 Factors that Influence Body Temperature of the PACU Patient
Premedications, anesthesia, and the stress of surgery all interact in a complex fashion to disrupt normal thermoregulation. Both hypothermia (temperature less than 36° C) and hyperthermia (temperature greater than 38° C) are associated with physiologic alterations that may interfere with recovery (Box 27-2). Patients at the age extremes and those with extreme debilitation are at even greater risk for postoperative development of temperature abnormalities.
BOX 27-2 Physiologic Alterations Associated with Hypothermia and Hyperthermia
Hypothermia | Hyperthermia |
---|---|
Bluish tint to skin (cyanosis) | Pale skin (mottled) |
Increased metabolic rate with shivering, then decreased metabolic rate | Increased metabolic rate |
Decreased oxygen consumption | Increased oxygen consumption |
Decreased muscle tone | Decreased muscle tone |
Decreased heart rate | Increased heart rate (rapid and bounding) |
Dysrhythmias | Dysrhythmias |
Decreased level of consciousness | Alterations in central nervous system (patient may be agitated) |
Core temperature (approximate value of temperature of blood perfusing the major metabolically active organs) is measured via the pulmonary artery, distal esophagus, nasopharynx, or tympanic membrane (using infrared thermistor). Monitoring the true core temperature is unrealistic and not clinically feasible for use on all patients in the PACU. Therefore, other measurement techniques (axillary, rectal, tympanic, temporal artery, oral) are required in the PACU even while their correlation to core temperature is debated. Consistency in use of the same method of measurement is required so that trends can be spotted and management of hypothermia begun.
Source: Hooper VD et al: ASPAN’s evidence-based clinical practice guideline for the promotion of perioperative normothermia, ed 2, J Perianesth Nurs 25:346-365, 2010.
Management of the patient with hypothermia is directed toward the restoration of normothermia and the avoidance of shivering. Warm blankets can be placed over the patient as specific hospital protocol allows. Forced air warming systems provide a safe and effective means of gradually rewarming the patient. Hypothermia and hyperthermia are discussed in greater detail in Chapter 53.
Fluid and electrolyte balance
Evaluation of a patient’s fluid and electrolyte status involves total body assessment. Imbalances readily occur in the postoperative patient for a number of reasons, including the restriction of food and fluids before surgery, fluid loss during surgery, patient’s physical status, and stress. The normal body response to stress of surgery is renal retention of water and sodium. In addition, patients often have abnormal avenues of postoperative fluid loss (see Chapter 14).
Fluid intake
Intravenous fluids
After ensuring that the intravenous fluids are infusing correctly, check to see what fluids, if any, are to follow or if the infusion is to be discontinued. If the patient is receiving total parenteral nutrition and intralipids, only feeding solutions should go through this line; another intravenous pathway must be secured for other uses. Multilumen catheters allow for the administration of multiple fluids and medications, and central line catheters can be connected to a transducer to provide continuous hemodynamic monitoring, if indicated.
Oral fluids
Oral intake must be prohibited after anesthesia until the laryngeal and pharyngeal reflexes are fully regained, as evidenced by the patient’s ability to gag and swallow effectively. If the patient is permitted oral intake, starting with small amounts of ice chips is best because they are less likely to cause nausea and vomiting. Some PACUs use isotonic ice chips that are made from a balanced electrolyte solution, such as Lytren, Pedialyte, and Ricelyte. If ice chips are well tolerated, the patient can progressively increase oral intake to include water and other clear liquids. Kool-Aid and fruit-flavored popsicles are well tolerated and accepted by children and adults. In addition, carbonated beverages may be soothing to a patient who feels slightly nauseated. The management of postoperative nausea and vomiting is discussed in Chapters 16 and 29.
Fluid output
The surgical site should be noted on admission to the PACU, and the dressing should be checked for drainage. The perianesthesia nurse must be aware of the presence of any drains and the expected amount of drainage. Drainage tubes should be checked to ensure patency, and the amount, color, and odor of any drainage should be observed and documented. All tubes should be secure and either clamped shut or connected to drainage apparatus as ordered by the physician. A summary of imbalances that may occur with abnormal avenues of output is presented in Table 27-3. Any deviations from the normally expected drainage in a specific route should be reported promptly to the surgeon.
Obviously, the accurate measurement and recording of all intake and output is vital to the assessment of each patient’s fluid and electrolyte status. A running total kept on the postanesthesia flow sheet or online documentation system is essential for quick assessment of fluid status. In addition to observation and assessment of avenues of intake and output, the perianesthesia nurse should be alert to symptoms of fluid and electrolyte imbalance, which are summarized in Table 27-4.
Table 27-4 Signs and Symptoms of Acute Fluid and Electrolyte Imbalance
IMBALANCE | SYMPTOMS AND FINDINGS |
---|---|
Hyperosmolarity | |
Water excess, sodium deficit | Polyuria (if kidneys are healthy), twitching, hyperirritability, disorientation, nausea, vomiting, weakness, serum Na < 120 mEq/L |
Isotonic Disturbances | |
Dehydration | Weakness, nausea, vomiting, oliguria, postural drop in systolic BP, elevated hematocrit, normal serum Na+ |
Circulatory collapse | Shock |
Volume excess | Dyspnea, cough, sweating, edema |
Hydrogen Ion Imbalances | |
Metabolic acidosis |
Bicarbonate loss: nausea, vomiting, abdominal discomfort, Weakness, tremors, malaise, headache, Kussmaul respiration, hypotension, tachycardia and other dysrhythmias, confusion, drowsiness, lethargy leading to coma Symptoms of K+ excess, ABG pH < 7.35, HCO−3 < 25, acid urine with pH < 6.0; increased rate and depth of breathing |
Hyperventilation: tachycardia, palpitations, dry mouth, anxiety, profuse perspiration, paresthesia of mouth and extremities, dizziness, vertigo, increased muscle twitching, tetany, inability to concentrate, seizures, coma
Increased irritability, disorientation, shallow slow respirations, periods of apnea, irregular pulse, muscle twitch, ABG pH > 7.45, HCO−3 > 29, alkaline urine with pH > 7.0
Weakness, mental confusion, shallow respirations, hypotension, dysrhythmias, serum K+ < 3.5 (measurement of extracellular K+ and only gives vague reflection of intracellular balance)
Four common causes of hypokalemia: reduced intake, GI losses, excessive renal loss of K+, K+ shifts from extracellular to intracellular, metabolic alkalosis
BP, Blood pressure; ABG, arterial blood gas; GI, gastrointestinal; PCO2, partial pressure of carbon dioxide.
Musculoskeletal assessment
An increase of orthopedic surgical procedures for both ambulatory and hospitalized patients provides a challenge to the perianesthesia nurse. Primary risk factors for complications in those with musculoskeletal injuries include the patient’s multiple comorbid conditions, age, and the multiple types of drugs used, including anticoagulants, steroids, estrogens, and opioids. Assessment and care of these patients include consideration for circulation, reflexes, positioning, transfer, and ambulation. See Chapter 37 for more specifics.
Integumentary system
Chapters 5 and 17 address aspects of the integumentary system. The integumentary system can be injured by the physical forces used in positioning and maintaining the patient position during and after surgery. Pressure, shear, friction, and maceration can cause damage to tissue integrity. The perianesthesia nurse must assess the patient’s skin and positioning to prevent integumentary complications from occurring.
Psychosocial assessment
Almost all surgical patients have a degree of anxiety about anesthesia and the surgical procedure and a fear of postoperative pain. The physical signs and symptoms of anxiety are the same as those produced by any stressor. Reactions are mediated by the sympathetic nervous system and are listed in Box 27-3.
Symptoms of anxiety must be carefully differentiated from those of other causes. Differentiation is particularly difficult while the effects of anesthesia are still present. A quiet and calm environment is important to the postanesthesia recovery of the surgical patient. A calm and confident nurse can do much to allay anxiety for the postoperative patient through both verbal reassurance and touch. Hearing is the first sense to return after anesthesia. Speaking loudly to patients is not necessary; they may not respond even if they can hear. In fact, yelling at patients may increase anxiety early in the PACU period, because the patients may believe they are not recovering as quickly as they should.
Attention to the psychosocial ramifications of specific surgical interventions is provided in each of the following chapters on postanesthesia care. These comments are incorporated into the overall text whenever deemed appropriate. For further discussion of the relationship between pain and anxiety, see Chapter 31.
Summary
Obviously, the perianesthesia nurse must be an expert in assessment. The perianesthesia nurse must not only understand the normal physiologic functioning of the human body but also be able to differentiate and evaluate the variety of pathologic symptoms that may arise in the postprocedure patient. The perianesthesia nurse must be aware of the interrelationships between mind and body and must be sensitive to the psychosocial factors that influence the patient’s reactions. Knowledgeable assessment of the postprocedure patient is essential for the provision of safe and effective treatment and nursing care.
1. Cole D, Schlunt M. Adult perioperative anesthesia: the requisites in anesthesiology. Philadelphia: Mosby; 2004.
2. American Society of PeriAnesthesia Nurses: Perianesthesia nursing: standards and practice recommendations 2010-2012. Cherry Hill, NJ: ASPAN; 2010.
3. Atlee J. Complications in anesthesia, ed 2. Philadelphia: Saunders; 2007.
4. Miller R, et al. Miller’s anesthesia, ed 7. Philadelphia: Churchill Livingstone; 2009.
5. Odom-Forren J. Capnography and sedation: A global initiative. J Perianesth Nurs.2011;26:221–224.
6. Godden B. Where does capnography fit into the PACU. J Perianesth Nurs. 2011;26(6):408–410.
7. Stoelting R, Miller R. Basics of anesthesia, ed 6. Philadelphia: Churchill Livingstone; 2011.
8. Urden L, et al. Priorities in critical care nursing, ed 5. St. Louis: Mosby; 2009.
9. Litman RS. Pediatric anesthesia: the requisites in anesthesiology. Philadelphia: Mosby; 2004.
10. Morton P, et al. Critical care nursing: a holistic approach. ed 9. Philadelphia: Lippincott Williams & Wilkins; 2009.
11. Diehl T, ed. Hemodynamic monitoring made incredibly visual, ed 2, Philadelphia: Lippincott Williams & Wilkins, 2011.
Alspach J. Core curriculum for critical care nursing, ed 6. Philadelphia: Saunders; 2006.
Arbour R. Impact of bispectral index monitoring on sedation and outcomes in critically ill adults: a case series. Crit Care Nurs Clin North Am.2006;18:227–241.
Barash P, et al. Clinical anesthesia, ed 6. Philadelphia: Lippincott Williams & Wilkins; 2009.
Bickley L, Hoekelman R. Bates’ guide to physical examination and history taking, ed 10. Philadelphia: Lippincott Williams & Wilkins; 2009.
Black J, Hokanson-Hawks J. Medical-surgical nursing: clinical management for positive outcomes. ed 8. St. Louis: Saunders; 2009.
Brunton L, et al. Goodman and Gilman’s the pharmacological basis of therapeutics, ed 12. New York: McGraw-Hill Professional; 2010.
Dennison R. Pass CCRN, ed 3. St. Louis: Mosby; 2007.
Fleisher L. Evidence-based practice of anesthesiology, ed 2. Philadelphia: Saunders; 2009.
Hall J. Guyton and Hall textbook of medical physiology, ed 12. Philadelphia: Saunders; 2011.
Headley JB, et al. Implementing nurse driven protocols: using arterial based cardiac output technologies AACN. available at: http://www.aacn.org/DM/CETests/Overview.aspx?TestID=33&mid=2864&ItemID=26, April, 25, 2011. Accessed
Heitz U, Horne M. Fluid, electrolyte, and acid-base balance, ed 5. St. Louis: Mosby; 2005.
Hickey J. The clinical practice of neurological and neurosurgical nursing, ed 6. Philadelphia: Lippincott Williams & Wilkins; 2008.
Katzung BG. Basic and clinical pharmacology, ed 11. Los Altos, Calif: Appleton & Lange; 2009.
Mason RJ, et al. Murry and Nadel’s textbook of respiratory medicine, ed 5. Philadelphia: Saunders; 2010.
McCance K, Huether S. Pathophysiology: the biologic basis for disease in adults and children. ed 6. St. Louis: Mosby; 2010.
Nagelhout J, Plaus K. Nurse anesthesia, ed 4. Philadelphia: Saunders; 2009.
Rathmell J, et al. Regional anesthesia: the requisites in anesthesiology. Philadelphia: Saunders; 2004.
Schick L, Windle PE. Perianesthesia nursing core curriculum: preoperative phase I and phase II PACU nursing. ed 2. St. Louis: Mosby; 2010.
Schumacker L, Chernecky C. Critical care & emergency nursing, ed 2. St. Louis: Saunders; 2009.
Springhouse. Critical care nursing made incredibly easy, ed 2. Ambler, Pa: Lippincott Williams & Wilkins; 2007.
Springhouse. IV therapy made incredibly easy, ed 4. Springhouse, Pa: Lippincott Williams & Wilkins; 2009.
Swearingen P. Manual of medical-surgical nursing care, ed 7. St. Louis: Mosby; 2010.