Chapter 9 Apoptosis Assessment
Introduction
A landmark of cellular self-destruction by apoptosis is the activation of nucleases and proteases that degrade the higher order chromatin structure of the DNA into fragments of 50 to 300 kilobases and subsequently into smaller DNA pieces of about 200 base-pairs in length. Activation of proteases, notably aspartate-specific cysteinyl proteases, referred to as caspases, is of primary relevance to apoptosis. Caspase-3 is considered to be the key mediator of apoptosis of mammalian cells, and its expression may be measured with immunohistochemical staining. Using fluorescent-labeled reagents, it is possible to tag the DNA break and identify the percentage of apoptotic cells with a high degree of accuracy.1–6
Measurable Features of Apoptosis
Apoptosis can also be characterized by changes in cell membrane structure. During apoptosis, the cell membrane’s phospholipid asymmetry changes—phosphatidylserine is exposed on the outer membrane, whereas membrane integrity is maintained. Annexin V specifically binds phosphatidylserine, whereas propidium iodide is a DNA-binding fluorochrome. When a cell population is exposed to both reagents, apoptotic cells stain positive for annexin V and negative for propidium iodide; necrotic cells stain positive for both, and live cells stain negative for both.3
This process of apoptosis and its analysis by flow cytometry are shown in Figures 9-1 and 9-2.
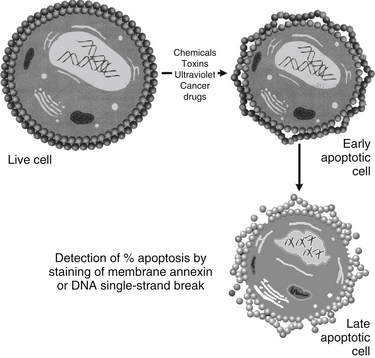
FIGURE 9-1 Detection of apoptosis using damaged membrane or DNA single-strand break and flow cytometry.
Another assessment of apoptosis involves ex vivo cell analysis. Specifically, the expression of active caspase-3 along with the Bcl-2/Bax ratio as markers of apoptosis can be measured. Immunohistochemical staining will reveal the expression of these apoptotic-related proteins, caspase-3 and cleaved caspase-3; the latter is indicative of apoptosis.7 Bcl-2 is anti-apoptotic gene product that exists in ratio to Bax and Bak, which are pro-apoptotic gene products. This ratio is indicative of the degree of apoptosis, with a decreased Bcl-2:Bax ratio indicative of apoptosis. Cells from Bax (−/−) and Bak(−/−) knockout animals do not respond to apoptosis inducers. In these cells, cytochrome C is not released from the mitochondrial membrane to initiate the caspase cascade.8 Thus, Bax and Bak are critical to apoptosis, and their expression in relation to Bcl-2 is highly correlative to apoptosis.
Different Stages of Apoptosis
The process of apoptosis is divided into three different stages:
These stages of apoptosis are depicted in Figure 9-3. Induction represents the initial events that signal a cell so that apoptosis may begin. This induction phase may be induced by various physical agents, such as toxic chemicals, hypoxia, radiation, chemotherapy agents, hormones, and CD95 or Fas ligation. It has been proposed that the induction stage of apoptosis is prevented by many antioxidants (vitamin C, β-carotene, and vitamin E) and also by various biological response modifiers, including lentinan, thymic hormones, viral antigens, and cytokines.
Apoptosis is Induced by Chemicals to Control Malignancy
Many chemicals have the capacity to bind to DNA, form DNA adducts, or cause DNA single-strand breaks, possibly leading to cancer. However, the body is equipped with many factors, enzymes, suppressor genes, and cellular sensors, all with the capacity to prevent the consequences of this DNA damage by activating apoptosis-inducing signals.
An important prediction of the relevance of apoptosis to malignancy is that the rate of apoptosis versus mitosis should influence the behavior of a tumor. Recently, the relationship between the apoptotic and mitotic indexes in a tumor was demonstrated as predictive of outcome: a higher ratio of apoptosis to mitosis within the tumor correlated with positive prognosis. Further, it was found that this was not simply a function of cell death per se. Tumors with a high incidence of necrosis rather than apoptosis were correlated with poor prognosis. It therefore follows that treatments or conditions that favor apoptosis should have desirable effects, and that defects in the pathways leading to apoptosis are likely to play important roles in the process of oncogenesis.4,5
Many reactive chemicals and drugs such as acetaminophen, diquat, carbon tetrachloride, quinones, cyanide, polyhydroxyl polyether, methyl mercury, and organotin have been implicated in apoptosis (programmed cell death) and necrosis (toxic cell death).9–16