Chapter 7 Anterior Cruciate Ligament Primary and Revision Reconstruction
Diagnosis, Operative Techniques, and Clinical Outcomes
INDICATIONS
Anterior Cruciate Ligament Primary Reconstruction in Acute and Chronic Ruptures
Patients presenting with acute complete ruptures of the anterior cruciate ligament (ACL; >5 mm of increased anterior tibial translation and positive pivot shift test) are treated with rehabilitation until pain and swelling subside and joint motion and muscle function are restored. This delay markedly reduces the incidence of postoperative complications of knee motion limitations and muscle weakness. All patients with acute ruptures are profiled with regard to their desired future activity level to determine whether ACL reconstruction is warranted.139 Those who wish to return to high-risk activities, including strenuous athletics or occupations involving pivoting, cutting, twisting, and turning, are considered for reconstruction. In patients with acute ACL ruptures and a concomitant displaced bucket-handle meniscus tear, surgery within 2 weeks is required to reduce the meniscus to a normal location and repair the tear. The ACL reconstruction may be performed at the same setting; however, knees with excessive swelling and pain undergo meniscus repair first. After an appropriate period of rehabilitation, ACL reconstruction is performed.
Patients involved in low-risk activities or who are willing to avoid strenuous athletic and occupational activities that place the knee at increased risk for giving-way episodes may not require ACL reconstruction. The patient is placed into a conservative treatment program that includes rehabilitation to regain muscle strength and neuromuscular function and counseling on the risk of future giving-way reinjuries and potential damage to the joint. Even with surgical reconstruction, patients are informed that an ACL rupture is a serious injury and it is unlikely that they will ever have a truly normal knee joint. The injury may also involve a bone bruise and chondral damage, with sequelae for future joint symptoms. The goal of conservative management is to prevent recurrent giving-way reinjuries, which are deleterious to the joint because they frequently result in meniscus tears and subsequent meniscectomy. It is important to categorize the knee joint as to the injury that has occurred. Repairable meniscus tears almost always indicate a concurrent ACL reconstruction. Otherwise, the success of the meniscus repair may be compromised.40,108,157 DeHaven and coworkers40 documented higher failure rates of meniscus repairs in knees with ACL deficiency than in knees with normal ACL function (46% and 5%, respectively) in a 10-year follow-up study. A grade III pivot shift and grossly positive Lachman test (increased ≥ 10 mm anterior tibial translation) indicate involvement of the secondary ligamentous restraints and, in the authors’ experience, an increased risk of giving-way reinjuries with recreational activities. Patients with physiologic laxity of other ligaments or partial tears (second-degree) of medial or lateral ligaments frequently have a grossly positive pivot shift test, as discussed in Chapter 3, The Scientific Basis for Examination and Classification of Knee Ligament Injuries.
The rationale for the indications for ACL reconstruction is based on a study of 103 patients with chronic ACL ruptures.141 These knees had no other ligament injuries and had not undergone reconstruction. The patients were evaluated a mean of 5.5 years after the initial knee injury; a subgroup of 39 patients presented an average of 11 years postinjury. This study was not a natural history study, because all patients sought treatment owing to symptoms. After the initial injury, 82% of the patients returned to sports activities, which gave an initial false impression of the seriousness of the ACL disruption. After 5 years, only 35% were continuing athletics, often with recurrent symptoms of pain or swelling. A significant reinjury occurred in 36 patients within 6 months and in 53 patients within the 1st year after the initial injury. Moderate to severe symptoms of pain, swelling, and/or giving-way were reported in 32 patients during walking, in 45 patients during activities of daily living, and in 73 patients during turning or twisting athletic activities. Giving-way occurred in 22 patients during walking, in 34 patients during recreational sports, and in 66 patients during strenuous sports. Knees that had undergone meniscectomy had a two- to fourfold increase in pain and swelling symptoms. Radiographic findings lagged behind clinical findings, because 44% of patients in the subgroup 11 years from injury had moderate to severe arthritic changes. The “ring” sign was noted, which represents an osteophyte (Fig. 7-1) that forms around the circumference of the lateral tibial plateau. This is associated with an absence of a pivot shift phenomena, but abnormal anterior tibial translation, because the lateral femoral condyle rotation is restrained by the osteophyte and increased concavity of the lateral tibial plateau.
In a follow-up study, 84 of the initial 103 patients underwent a repeat evaluation 3 years after initiating a conservative and activity modification treatment program.139 The frequently quoted “rule of thirds” represents the outcome of this study regarding the effects of an ACL injury on subsequent functional activities. Approximately one third of the patients showed improvement in symptoms and functional limitations with daily or recreational activities, one third did not improve, and one third became worse with continuing symptoms and limitations. Thirty percent of the patients were noncompliant with the activity modification recommendations and stated they would continue athletics despite symptoms and the increased risk for future arthritis (labeled “knee abusers”). These patients had the poorest overall prognosis with conservative management. This general rule of thirds is not specific to individual patients with chronic ACL insufficiency because it does not take into account the presence of meniscus and arthritis grading, compliance with activity modification, and maintenance of a muscle strengthening program. The frequency of giving-way episodes correlated with chronic pain, swelling, and functional limitations. The conclusion was reached that any giving-way episode with athletics, even if followed by periods of no giving-way or other symptoms, was to be absolutely avoided because meniscus tears and joint deterioration result. The results of this program show the importance of counseling the patient on the expected levels of athletic activity possible and following closely those who do not initially choose to have surgery to lessen the chance of significant arthritis in future years.
Partial ACL tears occur more frequently than suspected and present in a manner similar to complete ACL ruptures. These injuries frequently occur from a noncontact giving-way episode during jumping or pivoting and are often accompanied with a popping sensation and acute hemarthrosis. There is a limitation of full extension with pain on even gentle attempts to passively achieve 0°, mimicking a torn displaced meniscus, which requires magnetic resonance imaging (MRI) to exclude a mechanical block to knee extension. In a study reported by the senior author and associates140 of 32 knees with a partial ACL tear verified arthroscopically, a tear to one or both menisci occurred in 53%. An average of 67 months (range, 24–110 mo) postinjury, 38% had progressed to complete ACL deficiency. The arthroscopic evaluation of the extent of ACL disruption was related to the prognosis and progression to complete ACL deficiency. Even though the arthroscopic evaluation does not define the true extent of ligament injury on a microscopic or functional basis, 86% of knees in which 75% of the ACL was torn progressed to ACL deficiency.136 One half of the knees with an estimated injury involving 50% of the ligament progressed to ACL deficiency, even though major portions of the ACL were still intact, preventing a pivot shift phenomena. Only 12% of knees that had an estimated one fourth or less of the ligament torn progressed to complete ACL deficiency; therefore, these knees had a reasonably good prognosis for return to athletics.
Messner and Maletius104 followed 22 consecutive patients with partial ACL ruptures (no more than 50% of the ACL fibers torn) a mean of 12 years postinjury; all but 1 were also reexamined a mean of 20 years postinjury. No patient required ACL reconstruction. Patients decreased their level of activity from contact sports to recreational activities. Few had an increase in anterior translation or the pivot shift test, and little differences were noted in symptoms between follow-up evaluations.
Revision ACL Reconstruction
Many factors influence the failure rate of ACL surgery.* The definition of a failed ACL reconstruction includes a return of knee instability (increased anterior tibial translation and internal tibial rotation as demonstrated by positive Lachman and pivot shift tests) and, in many cases, the presence of associated pathologies. These include limitation of knee motion with pain and stiffness, muscle atrophy from inadequate rehabilitation, articular cartilage damage, meniscectomy and its effects on joint symptoms and function, undiagnosed lower limb malalignment, and posterolateral (PL) or medial ligament deficiency. When present, each of these pathologies must be addressed and resolved before or during ACL revision. The goal is to restore the best possible function to the knee joint and lower extremity before ACL revision to maximize the success rate and minimize the possibility of failure of the revision procedure. Importantly, the patient and surgeon must address the goals of the revision because associated knee joint damage requires modification or elimination of strenuous, high-impact activities. Often, the patient has had multiple prior surgical procedures that have not achieved the desired outcome.118,126,131 The potential of the revision operation in these cases to accomplish the patient’s goals must be realistically discussed.
CONTRAINDICATIONS
The presence of residual signs and symptoms of a complex regional pain syndrome (CRPS) requires careful examination and questioning regarding the presence of burning pain, abnormal skin hypersensitivity, extremity discoloration, and intolerance to cold and ice. Even after resolution of a prior CRPS, there is an increased frequency for return of the syndrome in these knees that must be promptly recognized in the postoperative period (see Chapter 43, Diagnosis and Treatment of Complex Regional Pain Syndrome).
CLINICAL BIOMECHANICS
Effect of ACL and Lateral Structures on Anterior Tibial Translation and Internal Tibial Rotation Limits
The function of the ACL is discussed in Chapter 3, The Scientific Basis for Examination and Classification of Knee Ligament Injuries. Additional information regarding the restoration of ACL function related to surgical techniques and graft placement issues are discussed in this section. The ACL is the primary restraint to anterior tibial translation, providing 87% of the total restraining force at 30° of knee flexion and 85% at 90° of flexion.29 The iliotibial band (ITB), midmedial capsule, midlateral capsule, medial collateral ligament (MCL), and fibular collateral ligament (FCL) provide a combined secondary restraint to anterior tibial translation. The posteromedial (PM) and PL capsule structures provide added resistance with knee extension. The secondary restraints may become deficient after an ACL injury or repeat giving-way episode, resulting in increased symptoms.
The ACL resists the coupled motions of anterior tibial translation and internal tibial rotation along with the lateral structures. In the authors’ laboratory, Wroble and colleagues196 measured in ACL-deficient knees the simulated effect of lateral soft tissue injuries on internal tibial rotation and anterior tibial translation. The experimental design consisted of a six-degrees-of-freedom instrumented spatial linkage and loading of the knee joint under defined loads of 100 N anteroposterior (AP), 15 Nm varus-valgus, and 5 Nm internal-external tibial rotation torque. The limits of knee motions were determined in the intact knee and then after sectioning the ACL, ITB, lateral capsule, popliteus tendon and popliteofibular ligament, and PL capsule.
The effect of sectioning the ACL first, followed by the ITB and lateral capsule, is shown in Figure 7-2. Two distinct responses were measured. In the first set of knees (see Fig. 7-2A), there were only moderate increases in anterior tibial translation after ACL sectioning and negligible further increases after sectioning the ITB and lateral capsule. However, in the second set of knees (see Fig. 7-2B), much larger increases were noted in anterior tibial translation after sectioning the ACL and even greater increases were found after sectioning the ITB and lateral capsule. These findings are compatible with clinical tests after ACL injury in which, in some knees, there may be only a moderate increase in anterior tibial translation, whereas in others, a much larger increase is present. This concept is discussed in Chapter 3, The Scientific Basis for Examination and Classification of Knee Ligament Injuries, using the analogy of the bumper model to simulate the effect of the secondary restraints on motion limits once the ACL is removed. Clinically, an ACL graft would be expected to be subjected to greater in vivo forces in the second set of knees without the unloading provided by relatively “tight” secondary restraints. The increase in the magnitude of anterior translation in these physiologically loose knees after loss of the anterolateral structures results in a grade III pivot shift phenomenon. These knees require a high-strength, securely fixated ACL graft to resist these major displacements, and autografts are highly recommended in these grossly unstable knees, as is discussed later.
Critical Points CLINICAL BIOMECHANICS
The effect of sectioning the lateral and posterolateral structures (PLS) is shown in Figure 7-3.196 Sectioning of the FCL or PLS (popliteus tendon, popliteofibular ligament, PL capsule) produced statistically significant, but small, increases in anterior tibial translation at low flexion angles. The combined ACL-ALS (anterolateral structures; ITB, anterior and middle portions of the lateral capsule)–FCL-sectioned knee had large increases in anterior tibial translation, with the greatest changes occurring at higher flexion angles.
The effect of sectioning the ACL and lateral structures on the limits of internal tibial rotation are shown in Figure 7-4. Sectioning of the ACL produced only a small increase in the final internal rotation limit. Sectioning of the ALS and the FCL produced a sequentially larger increase in internal tibial rotation. In select revision knees that have large increases in internal tibial rotation, the data support the role of a lateral extra-articular procedure to partially unload the ACL graft.
The motions that occur during the pivot shift maneuver are shown in Figure 7-5. At the beginning of the tests, the lower extremity is simply supported against gravity. After ACL disruption, both anterior tibial translation and internal tibial rotation increase as the femur drops back and externally rotates with subluxation of the lateral tibiofemoral compartment. This position is accentuated as the tibia is lifted anteriorly. At approximately 30° knee flexion, the tibia is pushed posteriorly, reducing the tibia into a normal position with the femur. From position C to position A (see Fig. 7-5B), the knee is extended to again produce the subluxated position. In addition to major increases in anterior tibial translation, there is also an increase in internal tibial rotation (midrange limit increase).
The effect of the ACL in providing rotational stability to the motions of anterior translation and internal tibial rotation is shown in Figure 7-6. After ACL sectioning, there is an increase in medial and lateral compartment translation as the center of rotation shifts from inside the knee joint to outside the medial compartment. The medial ligamentous structures influence the new center of rotation. With injury to the medial ligament structures, the center of rotation shifts so far medially that a rotational motion is essentially lost, resulting in a gross anterior subluxation of both compartments. The data show the important surgical concept of restoring injured medial and lateral ligament structures to restore anterior knee stability in conjunction with the ACL.
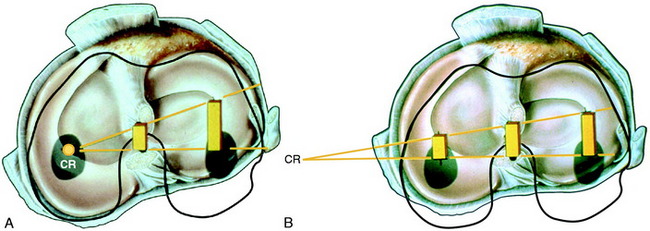
FIGURE 7-6 Intact knee and after ACL sectioning: response to coupled motions of anterior tibial translation and internal tibial rotation. A, Intact knee. The center of rotation may vary between the medial aspect of the PCL and the meniscus border, based on the loads applied and physiologic laxity of the ligaments. B, ACL sectioned; note shift in center of tibial rotation medially. The effect of the increase in tibial translation and internal tibial rotation produces an increase in medial and lateral tibiofemoral compartment translation (anterior subluxation). The millimeters of anterior translation of the tibiofemoral compartment represents the most ideal method to define knee rotational stability (see Chapter 3, The Scientific Basis for Examination and Classification of Knee Ligament Injury). The center of rotation under a pivot shift type of test shifts to the intact medial ligament structures. If these are deficient, the center of rotation shifts outside the knee joint.
Division of the ACL into Anteromedial and Posterolateral Bundles
Controversy exists in published studies on the division of the ACL into two distinct fiber bundles. Some authors provide evidence of both an anatomic and a functional division, whereas others doubt that this division exists and argue that ACL fiber function is too complex to be artificially divided into two bundles. In some studies,4,32 the anteromedial (AM) bundle is identified functionally at its femoral location as the proximal half of the attachment (knee in extension) that tightens with knee flexion. The PL bundle is identified as the distal half of the ACL femoral attachment that tightens with knee extension. The PL bundle is described to relax with knee flexion, as the ACL femoral attachment changes from a vertical to a horizontal structure. The problem is that this classic description of a reciprocal tightening and relaxation of the AM and PL bundles occurs under no anterior loading conditions. With substantial anterior tibial loading, and in particular with a coupled motion of anterior translation and internal tibial rotation, the majority of the ACL fibers are brought into a load-sharing configuration to a differing percentage, as is presented later.
The authors believe the characterization of the ACL into two fiber bundles represents a gross oversimplification not supported by biomechanical length-tension laboratory studies.70,169 Zavras and coworkers205 published a comparative study on previously published isometric points for the ACL and concluded that the ACL isometric zone was high and proximal in the ACL attachment, close to the posterior end of Blumensaat’s line. This data, along with other studies, focused on placing grafts in the proximal aspect of the ACL attachment, in a so-called isometric position. The problem with applying published isometric data to the clinical situation is that the data are valid only for knee flexion-extension and do not indicate the most effective ACL graft position for controlling knee rotational loading as occurs during the pivot shift test. To date, data regarding the most effective graft positions to resist the coupled motions of anterior translation and internal rotation are not available. However, there are data on what graft placement positions are ineffective and should be avoided.
The length-tension behavior of ACL fibers is primarily controlled by the femoral attachment in reference to the center of femoral rotation, the coupled motions applied, the resting length of ACL fibers, and tibial attachment locations. In Chapter 3, The Scientific Basis for Examination and Classification of Knee Ligament Injuries, the concept of an isometric transition zone or contour plot is presented. The zone represents a central transition zone in which fibers undergo 2 mm of length change during knee flexion and extension. This zone is not an isometric zone, because the length change is not zero. All ACL fibers anterior to the zone lengthen with knee flexion, whereas the posterior fibers lengthen with knee extension. Under loading conditions, fibers in both the AM and the PL divisions contribute to resist tibial displacements. ACL fibers in the PL division attach in part posterior to the transitional zone and lengthen with knee extension. Note that the function of the ACL fibers is determined by the anterior-to-posterior direction (knee at extension) as well as the proximal-to-distal femoral attachment. Placement of a graft in an anterior or a posterior position has a large effect in producing deleterious lengthening and graft failure. The obvious example is a graft placed anterior to “residents’ ridge,” which is anterior to the femoral ACL attachment. The proximal-to-distal division used in two-bundle descriptions as the control of fiber length in knee flexion and extension oversimplifies the functional behavior of the ACL fibers, which are even more dependent on the AP femoral attachment.
The division of the ACL into two bundles was historically based on the tibial attachment site and not on a corresponding femoral attachment site. Recent studies project the tibial bundles onto two corresponding femoral sites. In these studies, to be described, the authors usually divide the ACL femoral attachment site at a midpoint into a corresponding AM and PL bundle. In the authors’ opinion, there is not at present convincing anatomic data to support a division of the ACL into two separate bundles, although one study50 reported a bony ridge between the two femoral attachment bundles. Because of the lack of a clear anatomic division of the ACL into two bundles, there is discrepancy among authors on anatomic descriptions of the ACL “bundles” and recommendations for the surgical technique on tibial and femoral tunnels for two bundle graft reconstructions.
Colombet and associates32 measured the ACL femoral and tibial attachments of the AM and PL bundles in seven unpaired cadaveric knees. The reference position of the retroeminence ridge (RER), representing the posterior interspinous ridge anterior to the posterior cruciate ligament (PCL) attachment, provided an important landmark for measuring from this point to the posterior ACL attachment. The tibial measurement points are shown in Figure 7-7 and the mean values are displayed in Table 7-1. Note the ACL extends posteriorly on the tibia to a distance 10.3 ± 1.9 mm from the RER line (coordinate bg). There is a discrepancy in the published text where this measurement is reported to be 7.1 mm. The mean length of the ACL (coordinate ag) was 17.6 ± 2.1 mm. The distance between the center of the AM and the center of the PL bundle (coordinate ef) was 8.4 ± 0.6 mm. The authors reported that the AM bundle was an average of 17.8 ± 1.7 mm anterior to the RER. The center of the ACL attachment was 19 mm anterior to the RER line. The center of the PL and AM bundles was determined to lie at 52% and 36% of the tibial width (line described by Amis and Jakob5 that passes through the posterior corner of the tibial plateau and parallel to the medial tibial surface). The appearance of the ACL tibial attachments of the AM and PL bundles are shown in Figure 7-8 and represents a medial-to-lateral division, different from other authors who depict an anterior-to-posterior division.
The corresponding femoral attachments of the ACL from this study are shown in Figures 7-9 and 7-10 and the mean values are provided in Table 7-2. The overall length of the ACL was 18.3 ± 2.3 mm, with the center of the AM and PL bundles a mean of 8.2 mm apart and approximately 5 mm from the proximal and distal end of the ACL attachment. The authors recommended that the center of the PL bundle be located 8 mm lower and “shallower” in the notch than the center of the AM bundle. The femoral attachment was 2.5 mm from the articular cartilage. Using the grid system of Bernard and colleagues19 (shown in Fig. 7-10), the center of the AM bundle was 26.4% ± 2.6%, and the center of the PL bundle was 32.3% ± 3.9% the length of Blumensaat’s line.
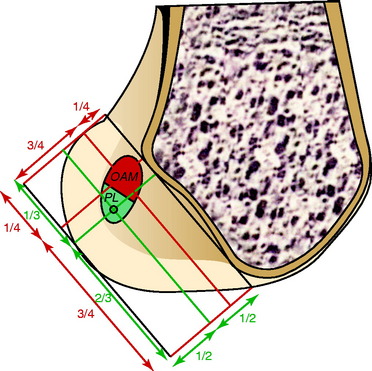
FIGURE 7-10 Position of the centers of the AM and PL bundles on the grid described by Bernard et al.19
(From Colombet, P.; Robinson, J.; Christel, P.; et al.: Morphology of anterior cruciate ligament attachments for anatomic reconstruction: a cadaveric dissection and radiographic study. Arthroscopy 22:984–992, 2006.)
In the most extensive study to date, Edwards and coworkers45 defined the ACL tibial attachment in 55 cadaveric specimens. In Figure 7-11, the tibial bony landmarks are depicted. The “over-the-back” ridge (RER) again corresponds to the interspinous ridge just anterior and proximal to the PCL attachment. In Figure 7-12, the authors present their recommendations for best-fit central and two tunnel positions. The center of the ACL attachment was 15 ± 2 mm (range, 11–18 mm) from the RER at 36% of the AP depth of the tibia. The center of the PL bundle anterior from the RER was 10 ± 1 mm (range, 8–13 mm) and the AM bundle was 17 ± 2 mm (range, 13–19 mm), corresponding to 29% and 46% of the tibial depth, respectively. The authors noted, in disagreement with other publications, that they were not able to define two separate anatomic bundles. Instead, they defined two functional bundles by observing the tightening and slackening behavior of the ACL fibers during flexion and extension.
Stabuli and Rauschning174a reported the center of the ACL tibial attachment at 43% of the AP depth, which extended from 25% to 62% of the tibial width. These important landmarks provide a reference to measure lateral radiographs before and after ACL graft placement to avoid a posterior and vertical graft placement (Fig. 7-13). Siebold and associates171 performed a cadaveric study in 50 knees and documented the ACL tibial insertion using a digital image analysis system and divided the ACL into AM and PL bundles. The average AP length of the ACL tibial attachment was 14 mm (range, 9–18 mm). The average male ACL tibial insertion area was 130 mm2 versus the average female knee of 106 mm2. The average AP length of the ACL footprint in females was 14 mm (range, 9–18 mm), and in males, 15 mm (range, 12–18 mm). The average width of the ACL in all knees was 10 mm. The study concluded that two tibial bone tunnels cannot be placed at the anatomic centers of the AM and PL bundles because the space is too narrow and the tunnels would overlap. This highlights the difficulty of the ACL double-bundle technique in re-creating the native ACL fiber attachment.
Zantop and colleagues204 performed a cadaveric study in 20 knees that outlined the AM and PL bundle locations at the tibial and femoral sites. The authors arrived at different conclusions from those of Siebold and associates.171 The tibia ACL division into the AM bundle was oriented from medial to lateral and occupied the anterior one half of the ACL footprint and was aligned with the anterior horn of the lateral meniscus. The center of the PL bundle was located 11 mm posterior and 4 mm medial to the anterior insertion of the lateral meniscus. The center of the AM bundle was located at 30% and the PL bundle was located at 40% on the lateral transtibial line.
Edwards and coworkers46 described the anatomic locations of the ACL femoral attachments of the AM and PL bundles in a companion study in 22 cadaveric knees using a measurement grid system shown in Figure 7-14. The authors reported a wide variation in the size and shape of the ACL attachment and bundle arrangement that was selected (Fig. 7-15). Note that the AM and PL bundle divisions differ from the prior study. The femoral attachment was oriented at 37° to the long axis of the femur. The authors reported that the AM bundle extended to the posterior proximal limit of the femoral notch between the 10:30 and the 11:30 positions. The PL bundle was located between the 9:00 and the 10:30 positions. The authors reported that a 6-mm graft tunnel had the best fit if the AM bundle was located at 11 o’clock, 6 mm from the posterior outlet, and the PL bundle was located at 10 o’clock, 9 mm from the posterior outlet. The authors noted that other studies had different recommendations for the placement of two femoral tunnels in the double-bundle technique.
Rue and associates159 in a cadaveric study used a transtibial-drilled femoral tunnel, placed at the 10:30 position, and determined the location of a single-graft femoral tunnel in relation to the AM and PL bundles. The authors placed the guide pin for the tibial tunnel approximately 7 mm anterior to the PCL, which would represent a posterior one third tibial attachment. The authors reported the transtibial drilling of the femoral tunnel resulted in the footprint of the AM occupying an average of 32% (range, 3%–49%) of the area of the tunnel, and the footprint of the PL bundle occupying an average of 26% (range, 7%–41%). In addition, the remainder of the area of the 10-mm tunnel did not overlap the ACL footprint. The wide ranges reported in this study for the location of the femoral tunnel in terms of the native ACL femoral attachment highlight the problems of using the tibial tunnel to drill the femoral tunnel.
Heming and colleagues71 reported the ACL tibial and femoral footprints in 12 cadaveric knees (Fig. 7-16). Note in Figure 7-16C, the orientation of the clock face places the 9 to 3 o’clock horizontal line at the base of the femoral condyles and not within the center of the notch, which is typically used by other authors. A guide pin placed between the ACL anatomic femoral and tibial attachment centers (as in a transtibial drilling technique) was only possible if the tibial tunnel started in a medial position close to the joint line that would be too short to be functional for ACL grafts. The study concluded that transtibial drilling techniques resulted in a more vertical graft orientation.
ACL Function Defined by the Role of AM and PL Divisions
Sakane and coworkers,160 in one of the first cadaveric robotic studies, reported the PL bundle provided the primary restraint to anterior tibial translation at low flexion angles. Increasing forces were reported in the AM bundle with knee flexion; these remained lower than the calculated in situ forces of the PL bundle.
The role of the ACL bundles in restraining rotational motions was examined by Zantop and associates203 in a robotic study. A 134-N anterior tibial load and a combined 10-Nm valgus and 4-Nm internal tibial torque were applied to cadaveric knees. Sectioning the PL bundle at 30° of flexion resulted in an approximately 7-mm increase in anterior tibial translation, whereas sectioning the AM bundle produced at 60° an approximately 9-mm increase in anterior translation. Similar data were reported at low flexion angles for the combined rotational loads. These data imply a near-absence of function of the remaining “intact” bundle. In contrast, Markolf and colleagues,97 in a cadaveric study, reported that cutting the PL bundle resulted in an increase of only 1.1 mm at 10° flexion and 0.5 mm at 30° flexion. These authors questioned the need to reconstruct the PL bundle for restoration of normal ACL laxity.
Gabriel and coworkers,55 in a robotic cadaveric experiment, removed all of the soft tissues to produce a femur-ACL-tibia specimen. For anterior tibial loading (Fig. 7-17), the AM bundle was reported to be nearly equal to the PL bundle at 15° of flexion, with increasing AM forces with knee flexion. Under the rotation loads of valgus and internal tibial rotation, the forces in the AM bundle exceeded those of the PL bundle (Fig. 7-18).
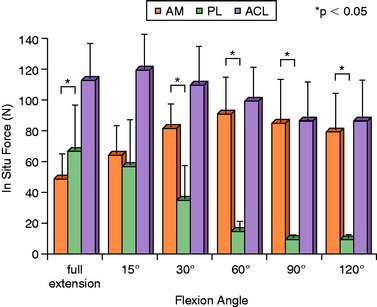
FIGURE 7-17 In situ force in the intact ACL and its AM and PL bundles in response to 134 N anterior tibial load.
(From Gabriel, M. T.; Wong, E. K.; Woo, S. L.; et al.: Distribution of in situ forces in the anterior cruciate ligament in response to rotatory loads. J Orthop Res 22:85–89, 2004; reprinted with permission of Wiley-Liss, Inc., a subsidiary of John Wiley & Sons, Inc.)
Li and associates90 used MRI and fluroscopic images of subjects performing a lunge to create three-dimensional models in which the AM and PL ACL attachments were outlined. The study reported that the AM and PL bundles reached their maximum elongation between full extension and 30° of flexion and did not demonstrate a reciprocal behavior with knee flexion-extension.