CHAPTER 84 Ankle and foot
The ankle joint (talocrural joint) is a diarthrodial articulation involving the distal tibia and fibula and the body of the talus: it is the only example in the human body of a true mortise joint. The human foot is a complex structure adapted to allow orthograde bipedal stance and locomotion and is the only part of the body which is in regular contact with the ground. There are 28 separate bones in the human foot, including the sesamoid bones of the first metatarsophalangeal joint and 31 joints, including the ankle joint.
SKIN AND SOFT TISSUE
SKIN
Cutaneous innervation
The skin covering the ankle and foot is supplied by the fourth and fifth lumbar and first sacral spinal nerves (see Figs 79.18, 79.20). Innervation of the dorsum of the foot is provided medially by the saphenous nerve, centrally by the superficial fibular nerve and laterally by the sural nerve; the deep fibular nerve supplies the dorsum of the first web space. Dorsal branches of the medial and lateral plantar nerves supply the nail beds. The plantar aspect of the foot is supplied by the medial and lateral plantar nerves, which arise as terminal branches of the tibial nerve. The medial plantar nerve supplies sensation to the plantar aspect of the hallux, the second, the third and the medial half of the fourth toes. The lateral plantar nerve supplies the remaining lateral aspect of the fourth and the entire fifth toe. The heel is innervated by calcaneal branches of the tibial nerve. Injury to any of these nerves can lead to painful neuromata and loss of protective sensation. The sural nerve is especially prone to neuroma formation.
SOFT TISSUES
Retinacula at the ankle
Extensor retinacula
Superior extensor retinaculum
The superior extensor retinaculum binds down the tendons of tibialis anterior, extensor hallucis longus, extensor digitorum longus and fibularis tertius immediately proximal to the anterior aspect of the talocrural joint (see Fig. 83.7; Fig. 84.1). The anterior tibial vessels and deep fibular nerve pass deep to the retinaculum and the superficial fibular nerve passes superficially. The retinaculum is attached laterally to the distal end of the anterior border of the fibula and medially to the anterior border of the tibia. Its proximal border is continuous with the fascia cruris, and dense connective tissue connects its distal border to the inferior extensor retinaculum. The tendon of tibialis anterior is the only extensor tendon that possesses a synovial sheath at the level of the superior extensor retinaculum.
Inferior extensor retinaculum
The inferior extensor retinaculum is a Y-shaped band lying anterior to the talocrural joint (see Fig. 83.7; Fig. 84.2A,B). The stem of the Y is at the lateral end, where it is attached to the upper surface of the calcaneus, in front of the sulcus calcanei. The band passes medially, forming a strong loop around the tendons of fibularis tertius and extensor digitorum longus (Fig. 84.2A). From the deep surface of the loop, a band passes laterally behind the interosseous talocalcaneal ligament and the cervical ligament and is attached to the sulcus calcanei. At the medial end of the loop, two diverging limbs extend medially to complete the ‘Y’ shape of the retinaculum. The proximal of the two limbs consists of two layers. The deep layer passes deep to the tendons of extensor hallucis longus and tibialis anterior, but superficial to the anterior tibial vessels and deep fibular nerve, to reach the medial malleolus. The superficial layer crosses superficial to the tendon of extensor hallucis longus and then adheres firmly to the deep one; in some cases it continues superficial to the tendon of tibialis anterior, before blending with the deep layer. The distal limb extends downwards and medially and blends with the plantar aponeurosis. It is superficial to the tendons of extensor hallucis longus and tibialis anterior, the dorsalis pedis artery and the terminal branches of the deep fibular nerve.
Flexor retinaculum
The flexor retinaculum is attached anteriorly to the tip of the medial malleolus, distal to which it is continuous with the deep fascia on the dorsum of the foot (Fig. 84.2B). From its malleolar attachment it extends posteroinferiorly to the medial process of the calcaneus and the plantar aponeurosis. Proximally, there is no clear demarcation between its border and the deep fascia of the lower leg, especially the deep transverse layer of the deep fascia. Distally, its border is continuous with the plantar aponeurosis, and many fibres of abductor hallucis are attached to it. The flexor retinaculum converts grooves on the tibia and calcaneus into canals for the tendons, and bridges over the posterior tibial vessels and tibial nerve. As these structures enter the sole they are, from medial to lateral, the tendons of tibialis posterior and flexor digitorum longus, the posterior tibial vessels, the tibial nerve and the tendon of flexor hallucis longus (see Fig. 84.14).
Synovial sheaths at the ankle
Anterior to the ankle, the sheath for tibialis anterior extends from the proximal margin of the superior extensor retinaculum to the interval between the diverging limbs of the inferior retinaculum (Figs 84.1, 84.2A,B). A common sheath encloses the tendons of extensor digitorum longus and fibularis tertius, starting just above the level of the malleoli, and reaching to the level of the base of the fifth metatarsal bone (Figs 84.1, 84.2A). The sheath for extensor hallucis longus starts at a level just distal to that for extensor digitorum longus and extends as far as the base of the first metatarsal bone (Figs 84.1, 84.2A,B).
Posteromedial to the ankle, the sheath for tibialis posterior starts approximately 4 cm above the medial malleolus and ends just proximal to the attachment of the tendon to the tuberosity of the navicular (Fig. 84.2B). The sheath for flexor hallucis longus starts at the level of the medial malleolus, and extends distally as far as the base of the first metatarsal bone (Fig. 84.2B). Occasionally, as a result of overuse, particularly in ballet dancers where balance on the tips of the toes en pointe involves sustained extreme plantar flexion of the ankle and first toe in the weight-bearing position, a fibrous nodule may develop in the tendon, just proximal to the tendon sheath. This may result in the thickened tendon being caught intermittently in the sheath, causing pain and ‘triggering’ of the great toe, a condition referred to as hallux saltans. Surgical opening of the sheath may be required. In athletes, the muscle belly of flexor hallucis longus may be abnormally large and may extend more distally than usual; it can also catch at the opening of the sheath. The sheath for flexor digitorum longus starts slightly above the level of the medial malleolus and ends at the level of the navicular (Fig. 84.2B).
Posterolateral to the ankle, the tendons of fibularis longus and brevis are enclosed in a sheath that is single proximally but double distally (Fig. 84.2A). From the tip of the lateral malleolus it extends for about 4 cm both proximally and distally.
Plantar fascia
The plantar fascia or aponeurosis is composed of densely compacted collagen fibres orientated mainly longitudinally, but also transversely (Fig. 84.3). Its medial and lateral borders overlie the intrinsic muscles of the hallux and fifth toe respectively, while its dense central part overlies the long and short flexors of the digits.
The central part is the strongest and thickest. The fascia is narrow posteriorly, where it is attached to the medial process of the calcaneal tuberosity proximal to flexor digitorum brevis, and traced distally it becomes broader and somewhat thinner. Just proximal to the level of the metatarsal heads it divides into five bands, one for each toe. As these five digital bands diverge below the metatarsal shafts, they are united by transverse fibres (Fig. 84.3). Proximal, plantar and a little distal to the metatarsal heads and the metatarsophalangeal joints, the superficial stratum of each of the five bands is connected to the dermis by skin ligaments (retinacula cutis). These ligaments reach the skin of the ball of the foot proximal to, and in the floors of, the furrows that separate the toes from the sole: Dupuytren’s disease may involve these ligaments resulting in contractures of the affected digits. The deep stratum of each digital band of the aponeurosis yields two septa that flank the digital flexor tendons and separate them from the lumbricals and the digital vessels and nerves. These septa pass deeply to fuse with the interosseous fascia, the deep transverse metatarsal ligaments (which run between the heads of adjacent metatarsals), the plantar ligaments of the metatarsophalangeal joints, and the periosteum and fibrous flexor sheaths at the base of each proximal phalanx. Pads of fat develop in the webs between the metatarsal heads and the bases of the proximal phalanges; they cushion the digital nerves and vessels from adjoining tendinous structures and extraneous plantar pressures. Just distal to the metatarsal heads, a plantar interdigital ligament (superficial transverse metatarsal ligament) blends progressively with the deep aspect of the superficial stratum of the plantar aponeurosis where it enters the toes (Fig. 84.3). The central part of the plantar aponeurosis thus provides an intermediary structure between the skin and the osteoligamentous framework of the foot via numerous cutaneous retinacula and deep septa that extend to the metatarsals and phalanges. The central part is also continuous with the medial and lateral parts: at the junctions, two intermuscular septa, medial and lateral, extend in oblique vertical planes between the medial, intermediate and lateral groups of plantar muscles to reach bone. Thinner horizontal intermuscular septa, derived from the vertical intermuscular septa, pass between the muscle layers.
The lateral part of the plantar aponeurosis, which covers abductor digiti minimi, is thin distally and thick proximally, where it forms a strong band, sometimes containing muscle fibres, between the lateral process of the calcaneal tuberosity and the base of the fifth metatarsal bone. It is continuous medially with the central part of the aponeurosis, and with the fascia on the dorsum of the foot around its lateral border. The medial part of the plantar aponeurosis, which covers abductor hallucis, is thin. It is continuous proximally with the flexor retinaculum, medially with the fascia dorsalis pedis, and laterally with the central part of the plantar aponeurosis.
Fascial compartments of the foot
There are four main compartments of the plantar aspect of the foot (Jones 1949) (Fig. 84.4). The medial compartment contains abductor hallucis and flexor hallucis brevis, and is bounded inferiorly and medially by the medial part of the plantar aponeurosis and its medial extension, laterally by an intermuscular septum, and dorsally by the first metatarsal. The central compartment contains flexor digitorum brevis, the lumbricals, flexor accessorius and adductor hallucis, and is bounded by the plantar aponeurosis inferiorly, the osseofascial tarsometatarsal structures dorsally and intermuscular septa medially and laterally. The lateral compartment contains abductor digiti minimi and flexor digiti minimi brevis, and its boundaries are the fifth metatarsal dorsally, the plantar aponeurosis inferiorly and laterally, and an intermuscular septum medially. The interosseous compartment contains the seven interossei and its boundaries are the interosseous fascia and the metatarsals.
Compartment syndrome in the foot
A compartment syndrome results from an increase in intracompartmental pressure sufficient to impair venous outflow from that compartment. As blood enters at arterial pressure, the compartment pressure increases further until it exceeds arterial pressure, at which point inflow of arterial blood ceases, leading to muscle and nerve ischaemia. Failure to relieve the increased pressure in the compartment surgically results in necrosis of the soft tissues within the compartment. The most common cause of compartment syndrome in the foot is trauma, usually of high-energy (high impact) type: crush injuries, calcaneal fractures and disruption of the tarsometatarsal joints are the usual antecedents associated with compartment syndrome in the foot.
BONE
DISTAL TIBIA
The distal end of the tibia has anterior, medial, posterior, lateral and distal surfaces, and projects inferomedially as the medial malleolus (see Figs 83.2A,B; 83.3A,B). The distal surface articulates with the talus and is wider anteriorly than posteriorly. It is concave sagittally and slightly convex transversely and continues medially into the malleolar articular surface. The medial malleolus is short and thick and has a smooth lateral surface with a crescentic facet that articulates with the medial surface of the talar body. The distal end of the tibia, including its ossification, is described in detail in Chapter 83.
DISTAL FIBULA
The distal end of the fibula or lateral malleolus projects distally and posteriorly relative to the medial malleolus (see Figs 83.2A,B; 83.3A,B). Its lateral aspect is subcutaneous, the posterior surface has a broad groove with a prominent lateral border, and the anterior surface is rough and somewhat rounded and articulates with the anteroinferior aspect of the tibia. The medial surface has a triangular articular facet, vertically convex with its apex directed distally. It articulates with the lateral talar surface. Behind the facet is a rough malleolar fossa. The distal end of the fibula, including its ossification, is described in detail in Chapter 83.
TARSUS
The seven tarsal bones occupy the proximal half of the foot (Figs 84.5A,B; 84.6). The tarsus and carpus are homologous, but the tarsal elements are larger, reflecting their role in supporting and distributing body weight. As in the carpus, tarsal bones are arranged in proximal and distal rows, but medially there is an additional single intermediate tarsal element, the navicular. The proximal row is made up of the talus and calcaneus; the long axis of the talus is inclined anteromedially and inferiorly, its distally directed head is medial to the calcaneus and at a higher level. The distal row contains, from medial to lateral, the medial, intermediate and lateral cuneiforms and the cuboid. Collectively these bones display an arched transverse alignment that is dorsally convex. Medially, the navicular is interposed between the head of the talus and the cuneiforms. Laterally, the calcaneus articulates with the cuboid.
The tarsus and metatarsus are arranged to form intersecting longitudinal and transverse arches. Hence thrust and weight are not transmitted from the tibia to the ground (or vice versa) directly through the tarsus, but are distributed through the tarsal and metatarsal bones to the ends of the longitudinal arches. For the purposes of description, each tarsal bone is arbitrarily considered to be cuboidal in form, with six surfaces. The ossification sites and dates are summarized in Fig. 84.7.
Talus
The talus is the link between the foot and leg, through the ankle joint (see Figs 84.16 and 84.18).
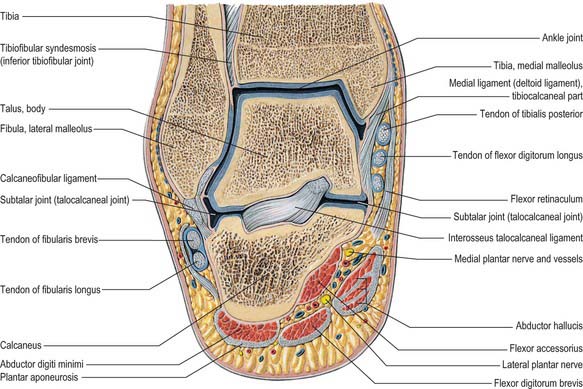
Fig. 84.16 Coronal section through the left ankle and talocalcaneal joint (seen from behind).
(From Sobotta 2006.)
Directed distally and somewhat inferomedially, the head has a distal surface which is ovoid and convex; its long axis is also inclined inferomedially to articulate with the proximal navicular surface. The plantar surface of the head has three articular areas, separated by smooth ridges. The most posterior and largest is oval, slightly convex and rests on a shelf-like medial calcaneal projection, the sustentaculum tali. Anterolateral to this and usually continuous with it, a flat articular facet rests on the anteromedial part of the dorsal (proximal) calcaneal surface; distally it continues into the navicular surface. Between the two calcaneal facets, a part of the talar head, covered with articular cartilage, is in contact with the plantar calcaneonavicular ligament, which is covered here, superiorly, by fibrocartilage (see Fig. 84.15A,B). When the foot is inverted passively, the dorsolateral aspect of the head is visible and palpable approximately 3 cm distal to the tibia; it is hidden by extensor tendons when the toes are dorsiflexed.
The long axis of the neck, inclined downwards, distally and medially, makes an angle of approximately 150° with that of the body; it is smaller (130–140°) at birth, accounting in part for the inverted foot in young children. The dorsal talonavicular ligament and ankle articular capsule are attached distally to its dorsal surface. Thus the proximal part of this surface lies within the capsule of the ankle joint. The medial articular facet of the talar body and part of the trochlear surface may extend onto the neck. The anterior talofibular ligament is attached on the lateral aspect of the neck, spreading along the adjacent anterior border of the lateral surface. The interosseous talocalcaneal and cervical ligaments are attached to the inferior surface of the neck. A dorsolateral, so-called ‘squatting facet’ is commonly present on the talar neck in those individuals who habitually adopt the squatting position: it articulates with the anterior tibial margin in extreme dorsiflexion and may be double.
The body is cuboidal, covered dorsally by a trochlear surface articulating with the distal end of the tibia. It is anteroposteriorly convex, gently concave transversely, widest anteriorly and, therefore, sellar. The triangular lateral surface is smooth and vertically concave for articulation with the lateral malleolus. Superiorly, it is continuous with the trochlear surface; inferiorly its apex is a lateral process. Proximally, the medial surface is (posterosuperiorly) covered by a comma-shaped facet, which is deeper in front and articulates with the medial malleolus. Distally, this surface is rough and contains numerous vascular foramina. The small posterior surface features a rough projection termed the posterior process. The process is marked by an oblique groove between two tubercles which lodges the tendon of flexor hallucis longus. The lateral tubercle is usually larger; the medial is less prominent and immediately behind the sustentaculum tali (Fig. 84.8A). The plantar surface articulates with the middle one-third of the dorsal calcaneal surface by an oval concave facet, its long axis directed distolaterally at an angle of approximately 45° with the median plane. The medial edge of the trochlear surface is straight, but its lateral edge inclines medially in its posterior part and is often broadened into a small elongated triangular area which is in contact with the posterior tibiofibular ligament in dorsiflexion.
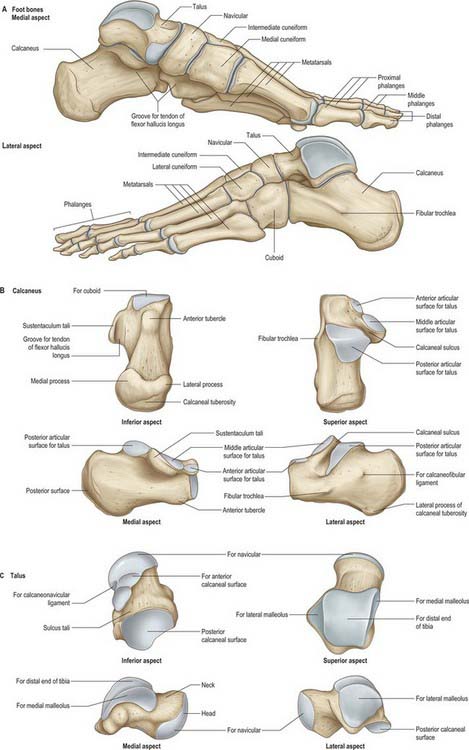
Fig. 84.8 Skeleton of the foot. A, Foot bones. B, Calcaneus. C, Talus.
(From Drake, Vogl, Mitchell, Tibbitts and Richardson 2008.)
The posterior talofibular ligament is attached to the lateral tubercle of the posterior process. Its attachment extends up to the groove, or depression, between the process and posterior trochlear border. The posterior talocalcaneal ligament is attached to the plantar border of the posterior process. The groove between the tubercles of the process contains the tendon of flexor hallucis longus and continues distally into the groove on the plantar aspect of the sustentaculum tali. The medial talocalcaneal ligament is attached below to the medial tubercle, whereas the most posterior superficial fibres of the deltoid ligament are attached above the tubercle. The deep fibres of the deltoid ligament are attached still higher to the rough area immediately below the comma-shaped articular facet on the medial surface (Fig. 84.8A,C).
No muscles are attached to the talus. However, many ligaments are attached to the bone, and these confer stability to the ankle, subtalar and talocalcaneonavicular joints (Fig. 84.8A–C).
The talar blood supply is rather tenuous because of the lack of muscle attachments. The first comprehensive account of talar blood supply was provided by Wildenauer in 1950. The extraosseous blood supply is via the posterior tibial, dorsalis pedis and fibular arteries (Fig. 84.9). The ‘artery of the tarsal canal’ arises from the posterior tibial artery approximately 1cm proximal to the origin of the medial and lateral plantar arteries (Fig. 84.10) and passes anteriorly between the sheaths of flexor digitorum longus and flexor hallucis longus to enter the tarsal canal in which it lies anteriorly, close to the talus. (The ‘tarsal canal’ is the term that is commonly used to describe the tunnel-shaped medial end of the sinus tarsi.) Branches from the arterial network in the tarsal canal enter the talus. The artery continues through the tarsal canal into the lateral part of the tarsal sinus, where it anastomoses with the artery of the tarsal sinus, forming a vascular sling under the talar neck. A branch of the artery of the tarsal canal known as the deltoid branch passes deep to the deltoid ligament and supplies part of the medial aspect of the talar body. Sometimes it arises from the posterior tibial artery; rarely, it arises from the medial plantar artery. In talar fractures it may be the only remaining arterial supply to the talus to maintain the viability of the talar body. The dorsalis pedis artery supplies branches to the superior aspect of the talar neck and also gives off the artery of the tarsal sinus. This large vessel is always present and anastomoses with the artery of the tarsal canal. The artery of the tarsal sinus receives a contribution from the anterior perforating branch of the fibular artery and supplies direct branches to the talus. The fibular artery provides small branches which form a plexus of vessels posteriorly with branches of the posterior tibial artery, however, the contribution that the fibular artery makes to the talar blood supply is thought to be insignificant.
A single ossification centre appears prenatally at 6 months (Fig. 84.7). The posterior process (Stieda’s process) is a separate bone in 5% of individuals and arises from a separate ossification centre, which appears between 8 and 11 years. In athletes and dancers, it may be susceptible to impingement against the posterior tibia, resulting in pain and sometimes requires surgical removal. Another accessory bone (although rare) is the os supratalare, which lies on the dorsal aspect of the talus; it rarely measures more than 4 mm in length.
The talus has no muscular attachments and 70% of its surface is covered by articular cartilage: displaced talar neck fractures, where the blood supply to the talar body is interrupted, can therefore result in avascular necrosis and non-union. In general, these complications do not occur in undisplaced fractures. If a subchondral lucency is seen in the talar dome on radiographs at 8 weeks after fracture of the talar neck (Hawkin’s sign), it may be assumed that vascularity to the talar body is intact and that the fracture is likely to heal satisfactorily.
Calcaneus
The calcaneus is the largest tarsal bone and projects posterior to the tibia and fibula as a short lever for muscles of the calf attached to its posterior surface. It is irregularly cuboidal, its long axis being inclined distally upwards and laterally (Fig 84.8A,B). The superior or proximal surface is divisible into three areas. The posterior one-third is rough and concavo-convex; the convexity is transverse and supports fibroadipose tissue (Kager’s fat pad) between the calcaneal tendon and ankle joint. The middle one-third carries the posterior talar facet, which is oval and convex anteroposteriorly. The anterior one-third is partly articular; distal (anterior) to the posterior articular facet, a rough depression, the sulcus calcanei, narrows into a groove on the medial side and completes the sinus tarsi with the talus. (The sinus tarsi is a conical hollow bounded by the talus medially, superiorly and laterally, with the superior surface of the calcaneus below. Its medial end is narrow and tunnel-shaped, and is often referred to as the tarsal canal.) Distal and medial to this groove, an elongated articular area covers the sustentaculum tali and extends distolaterally on the body of the bone. This facet is often divided into middle and anterior talar facets by a non-articular interval at the anterior limit of the sustentaculum tali (the incidence of this subdivision varies with sex, race and occupation). Rarely, all three facets on the upper surface of the calcaneus are fused into one irregular area. A detailed analysis of patterns of anterior talar articular facets in a series of 401 Indian calcanei revealed four types. Type I (67%) showed one continuous facet on the sustentaculum extending to the distomedial calcaneal corner; type II (26%) presented two facets, one sustentacular and one distal calcaneal; type III (5%) possessed only a single sustentacular facet; and type IV (2%) showed confluent anterior and posterior facets.
The plantar surface is rough, especially proximally as the calcaneal tuberosity, the lateral and medial processes of which extend distally, separated by a notch. The medial process is longer and broader (Fig. 84.8B). Further distally, an anterior tubercle marks the distal limit of the attachment of the long plantar ligament.
The lateral surface is almost flat. It is proximally deeper and palpable on the lateral aspect of the heel distal to the lateral malleolus. Distally, it presents the fibular trochlea (Fig. 84.8A,B), which is exceedingly variable in size and palpable 2 cm distal to the lateral malleolus when well developed. It bears an oblique groove for the tendon of fibularis longus and a shallower proximal groove for the tendon of fibularis brevis. About 1 cm or more behind and above the fibular trochlea, a second elevation may exist for attachment of the calcaneofibular part of the lateral ligament.
The medial surface is vertically concave, and its concavity is accentuated by the sustentaculum tali, which projects medially from the distal part of its upper border (Fig. 84.8B). Superiorly, the process bears the middle talar facets and inferiorly a groove which is continuous with that on the talar posterior surface for the tendon of flexor hallucis longus (Fig. 84.8A,B). The medial aspect of the sustentaculum tali can be felt immediately distal to the tip of the medial malleolus; occasionally it is also grooved by the tendon of flexor digitorum longus.
The calcaneus is the only tarsal bone that always has two ossification centres (Fig. 84.7). In addition to the main ossification centre, there is a scale-like posterior apophysis that covers most of the posterior, and part of the plantar, surfaces. The main centre appears prenatally in the third month, whereas the posterior apophysis appears in the sixth year in females and the eighth in males, fusing in the 14th and 16th years, respectively.
Navicular
The navicular articulates with the talar head proximally and with the cuneiform bones distally (Figs 84.8A,B; 84.11). Its distal surface is transversely convex and divided into three facets (the medial being the largest) for articulation with the cuneiforms. The proximal surface is oval and concave and articulates with the talar head. The dorsal surface is rough and convex. The medial surface is also rough and projects proximally as a prominent tuberosity, palpable approximately 2.5 cm distal and plantar to the medial malleolus. The plantar surface, rough and concave, is separated from the tuberosity medially by a groove. The lateral surface is rough, irregular and often bears a facet for articulation with the cuboid.
The facet for articulation with the medial cuneiform is roughly triangular, its rounded apex is medial and its ‘base’, facing laterally, is often markedly curved; the articular facets for the intermediate and lateral cuneiforms are also triangular, with plantar facing apices. The facet for the lateral cuneiform may approach a wide crescent or a semicircle rather than a triangle (Fig. 84.11). Dorsal talonavicular, cuneonavicular and cubonavicular ligaments are attached to the dorsal navicular surface.
Muscle and ligament attachments
The navicular tuberosity is the main attachment of tibialis posterior and a groove lateral to it transmits part of the tendon distally to the cuneiforms and middle three metatarsal bases. The plantar calcaneonavicular ligament is attached to a slight projection lateral to the groove and adjacent to the proximal surface. The calcaneonavicular part of the bifurcate ligament is attached to the rough part of the lateral surface (Fig. 84.5B).
The navicular ossification centre appears during the third year (Fig. 84.7). It is sometimes affected by avascular necrosis between the ages of 4 and 7 years (Köhler’s disease). An accessory navicular bone, which is considered an anatomic variant, occurs in approximately 5% of individuals. It arises from a separate ossification centre in the region of the navicular tuberosity. There are three distinct types of accessory navicular. Type I is probably a sesamoid bone within the plantar aspect of the tendon of tibialis posterior at the level of the inferior calcaneonavicular ligament. In type II, the accessory bone is separated from the body of the navicular by a synchondrosis. Type III is commonly called ‘the cornuate navicular,’ where the accessory bone is united to the navicular by a bony ridge, and may represent the possible end stage of type II. An accessory navicular may be the source of pain in athletes. Type II is the most commonly symptomatic variant: it has been suggested that the pull of the tendon of tibialis posterior, the degree of foot pronation, and the location of the accessory bone in relation to the undersurface of the navicular may produce tension, shear, and/or compression forces on the synchondrosis.
Cuboid
The cuboid, the most lateral bone in the distal tarsal row, lies between the calcaneus proximally and the fourth and fifth metatarsals distally (Fig. 84.11). Its dorsolateral surface is rough for the attachment of ligaments. The plantar surface is crossed distally by an oblique groove for the tendon of fibularis longus and bounded proximally by a ridge that ends laterally in the tuberosity of the cuboid, the lateral aspect of which is faceted for a sesamoid bone or cartilage that is frequently found in the tendon of fibularis longus. Proximal to its ridge, the rough plantar surface extends proximally and medially because of the obliquity of the calcaneocuboid joint, making its medial border much longer than the lateral. The lateral surface is rough; the groove for fibularis longus extends from a deep notch on its plantar edge. The medial surface, which is much more extensive and partly non-articular, bears an oval facet for articulation with the lateral cuneiform, and proximal to this another facet (sometimes absent) for articulation with the navicular: the two form a continuous surface separated by a smooth vertical ridge. The distal surface is divided vertically into a medial quadrilateral articular area for the fourth metatarsal base and a lateral triangular area, its apex lateral, for the fifth metatarsal base. The proximal surface, triangular and concavo-convex, articulates with the distal calcaneal surface; its medial plantar angle projects proximally and inferior to the distal end of the calcaneus.
Cuneiforms
Medial cuneiform
The medial cuneiform (Figs 84.5A,B; 84.11) articulates with the navicular and first metatarsal base. It has a rough, narrow dorsal surface. The distal surface is a reniform facet for the first metatarsal base, its ‘hilum’ being lateral. The proximal surface bears a piriform facet for the navicular, which is concave vertically and dorsally narrowed. The medial surface, rough and subcutaneous, is vertically convex; its distal plantar angle carries a large impression which receives the principal attachment of the tendon of tibialis anterior (Fig. 84.5B). The lateral surface is partly non-articular; there is a smooth right-angled strip along its proximal and dorsal margins for the intermediate cuneiform. Its distal dorsal area is separated by a vertical ridge from a small, almost square, facet for articulation with the dorsal part of the medial surface of the second metatarsal base. Plantar to this, the medial cuneiform is attached to the medial side of the second metatarsal base by a strong ligament. Proximally, an interosseous intercuneiform ligament connects this surface to the intermediate cuneiform. The distal and plantar area of the surface is roughened by attachment of part of the tendon of fibularis longus (Fig. 84.5B).
Intermediate cuneiform
The intermediate cuneiform articulates proximally with the navicular and distally with the second metatarsal base (Figs 84.5A; 84.11). It has a narrow, plantar surface that receives a slip from the tendon of tibialis posterior. The distal and proximal surfaces are both triangular articular facets and articulate with the second metatarsal base and the navicular, respectively. The medial surface is partly articular: it articulates via a smooth, angled strip that is occasionally double with the medial cuneiform along its proximal and dorsal margins. The lateral surface is also partly articular: along its proximal margin a vertical strip, usually indented, abuts the lateral cuneiform. Strong interosseous ligaments connect non-articular parts of both surfaces to the adjacent cuneiforms.
Lateral cuneiform
The lateral cuneiform is between the intermediate cuneiform and cuboid, and also articulates with the navicular and, distally, with the third metatarsal base (Figs 84.5A; 84.11). Like the intermediate cuneiform, its dorsal surface, which is rough and almost rectangular, is the base of a wedge. The plantar surface is narrow and receives a slip from tibialis posterior and sometimes part of flexor hallucis brevis. The distal surface is a triangular articular facet for the third metatarsal base. The proximal surface is rough on its plantar aspect, but its dorsal two-thirds articulate with the navicular by a triangular facet. The medial surface is partly non-articular and has a vertical strip, indented by the intermediate cuneiform, on its proximal margin; on its distal margin, a narrower strip (often two small facets) articulates with the lateral side of the second metatarsal base. The lateral surface, also partly non-articular, bears a triangular or oval proximal facet for the cuboid; a semilunar facet on its dorsal and distal margin articulates with the dorsal part of the medial side of the fourth metatarsal base. Non-articular areas of the medial and lateral surfaces receive intercuneiform and cuneocuboid ligaments, respectively, which are important in the maintenance of the transverse arch.
Tarsal coalition
Tarsal coalition is a hereditary condition in which there is a fibrous, cartilaginous or osseous union of two or more tarsal bones, and is believed to arise as a result of a failure of segmentation of primitive mesenchyme. Harris & Beath (1948) were the first to recognize an association between tarsal coalitions and ‘peroneal (fibular) spastic flat foot’. The two most common examples are talocalcaneal and calcaneonavicular coalitions, which usually present with symptoms early in the second decade of life. They are often, but not invariably, associated with flat feet (see below). A talonavicular coalition is rare, but when present is often associated with a ‘ball and socket’ ankle joint. Surgical resection of tarsal coalitions may eradicate associated pain but seldom improves the range of movement.
METATARSALS
Individual metatarsals
First metatarsal
The first metatarsal (Fig. 84.5A,B; 84.11) is the shortest and thickest, and has a strong shaft, of marked prismatic form. The base sometimes has a lateral facet or ill-defined smooth area as a result of contact with the second metatarsal. Its large proximal surface, usually indented on the medial and lateral margins, articulates with the medial cuneiform. Its circumference is grooved for tarsometatarsal ligaments and, medially, part of the tendon of tibialis anterior is attached; its plantar angle has a rough, oval, lateral prominence for the tendon of fibularis longus. The medial head of the first dorsal interosseous is attached to the flat lateral surface of the shaft. The large head has a plantar elevation, the crista, which separates two grooved facets (of which the medial is larger), on which sesamoid bones glide.
The first metatarsal has two centres of ossification, one in the shaft, the other in the base (unlike the other metatarsals, in which the secondary ossification centre is distal). They appear during the tenth week of prenatal life and the third year of life, respectively (Fig. 84.7) and fuse between the 17th and 20th years. There may be a third centre in the first metatarsal head.
Second metatarsal
The second metatarsal is the longest (Figs 84.5A,B; 84.11). Its cuneiform base bears four articular facets. The proximal one, concave and triangular, is for the intermediate cuneiform. The dorsomedial one, for the medial cuneiform, is variable in size and usually continuous with that for the intermediate cuneiform. Two lateral facets, dorsal and plantar, are separated by non-articular bone, each divided by a ridge into distal demifacets, which articulate with the third metatarsal base, and a proximal pair (sometimes continuous) for the lateral cuneiform. The areas of these facets vary, particularly the plantar facet, which may be absent. An oval pressure facet, caused by contact with the first metatarsal, may appear on the medial side of the base, plantar to that for the medial cuneiform. Because of its length, its steep inclination, and the position of its base recessed in the tarsometatarsal joint, it is at risk of stress overload; perhaps this is why it is a common site for stress fractures in athletes and an avascular phenomenon in its head (Freiberg’s infraction).
There are two centres of ossification, one in the shaft and one distally in the metatarsal head (Fig. 84.7). Ossification of the shaft starts during the ninth prenatal week and ossification of the metatarsal head starts between the third and fourth years; fusion occurs between the 17th and 20th years.
Third metatarsal
The third metatarsal (Figs 84.5A,B; 84.11) has a flat triangular base, articulating proximally with the lateral cuneiform, medially with the second metatarsal, via dorsal and plantar facets, and laterally, via a single facet, with the dorsal angle of the fourth metatarsal. The medial plantar facet is frequently absent. The third tarsometatarsal joint is relatively immobile and predisposes the third metatarsal to stress fracture.
There are two centres of ossification, one in the shaft and one distally in the metatarsal head (Fig. 84.7). Ossification of the shaft starts during the ninth prenatal week and ossification of the metatarsal head starts between the third and fourth years; fusion occurs between the 17th and 20th years.
Fourth metatarsal
The fourth metatarsal is smaller than the third (Figs 84.5A,B; 84.11). Its base has proximally, an oblique quadrilateral facet for articulation with the cuboid; laterally, a single facet for the fifth metatarsal; medially, an oval facet for the third metatarsal. The latter is sometimes divided by a ridge, in which case the proximal part articulates with the lateral cuneiform.
There are two centres of ossification, one in the shaft and one distally in the metatarsal head (Fig. 84.7). Ossification of the shaft starts during the ninth prenatal week and ossification of the metatarsal head commences between the third and fourth years; fusion occurs between the 17th and 20th years.
Fifth metatarsal
The fifth metatarsal has a tuberosity (styloid process) on the lateral side of its base (Figs 84.5A,B; 84.11). The base articulates proximally with the cuboid by a triangular, oblique surface, and medially with the fourth metatarsal. The tuberosity can be seen and felt midway along the lateral border of the foot; in acute inversion it may be fractured. The metaphysial–diaphysial junction of the fifth metatarsal base is prone to traumatic or stress fractures, and these have a tendency to delayed and non-union, and often require surgical fixation. It is believed that fractures at this level damage the nutrient artery and the extraosseous plexus, resulting in compromised vascularity of the fracture site and consequent poor fracture healing.
There are three centres of ossification, one at the base in the region of the tuberosity (an apophysis), one in the shaft and one distally in the metatarsal head. Ossification of the shaft starts during the tenth prenatal week and ossification of the metatarsal head starts between the third and fourth years (Fig. 84.7). Fusion of the distal and shaft centres occurs between the 17th and 20th years, the proximal apophysis fuses earlier. An os vesalianum is a rare variant that should not be confused with the basal apophysis. (Fig. 84.12)
PHALANGES OF THE FOOT
In general, the phalanges of the foot resemble those of the hand: there are two in the hallux, and three in each of the other toes (Fig. 84.5A,B). On occasion there are only two phalanges in the little toe and, rarely, this is the case with the other lesser toes. The phalanges of the toes are much shorter than their counterparts in the hand and their shafts, especially those of the proximal set, are compressed from side to side. In the proximal phalanges, the compressed shaft is convex dorsally, with a plantar concavity. The base is concave for articulation with a metatarsal head and the head is a trochlea for a middle phalanx. Middle phalanges are small and short, but broader than their proximal counterparts. Distal phalanges resemble those in the fingers, but are smaller and flatter. Each has a broad base for articulation with a middle phalanx and an expanded distal end. A rough tuberosity on the plantar aspect of the latter supports the pulp of the toe, and provides a weight-bearing area.
Tendons of the long digital flexors and extensors are attached to the plantar and dorsal aspects of the bases of the distal phalanges of the lateral four toes. Flexor hallucis longus and extensor hallucis longus are similarly attached to the hallux. The bases of the middle phalanges receive the tendons of flexor digitorum brevis and extensor digitorum brevis. The proximal phalanges of the second, third, fourth and fifth toes each receive a lumbrical on their medial side; those of the second, third and fourth toes also receive an interosseous muscle on both sides. For further details of muscular, capsular and ligamentous arrangements in the toes refer to Fig. 84.5A,B. The terminal phalanx of the hallux normally shows a small degree of valgus (lateral) deviation, as may the proximal phalanx. This is presumed to be unrelated to footwear, because this degree of. deviation has also been observed in fetal specimens.
Phalanges are ossified from a primary centre for the shaft and a basal epiphysis (Fig. 84.7). Primary centres for the distal phalanges appear between the ninth and twelfth prenatal weeks, somewhat later in the fifth digit. Primary centres for the proximal phalanges appear between the 11th and 15th weeks, and later for intermediate phalanges, but there is wide variation. Basal centres appear between the second and eighth years (usually second or third in the hallux), and union with the shaft occurs by the 18th year. There is considerable variation in ossification and fusion dates.
SESAMOIDS
Galen is believed to have been the first to use the term ‘sesamoid’ because of the resemblance of these bones to sesame seeds. Most sesamoid bones are only a few millimetres in diameter and their shape is variable. Some have a predictable location (see below), but many others vary in terms of location and frequency of occurrence (Fig. 84.12). Some sesamoids ossify, whereas others remain cartilaginous. Most sesamoid bones are embedded in tendons in close proximity to joints. Their precise role is not understood; it is believed that they may alter the direction of muscle pull, decrease friction and modify pressure.
Medial and lateral sesamoids of the first metatarsophalangeal joint
Approximately 30% of these sesamoid bones are bipartite. The medial is much more commonly affected and may have two, three or four parts, but the fibular sesamoid rarely has more than two. The condition may be bilateral. The sesamoids may be absent congenitally.
JOINTS
ANKLE (TALOCRURAL) JOINT
Articular surfaces are covered by hyaline cartilage. The talar trochlear surface, which is convex parasagittally and gently concave transversely, is wider in front; the distal tibial articular surface is reciprocally curved. The talar articular surface for the medial malleolus is a proximal area on the medial talar surface, and is fairly flat, comma-shaped and deeper anteriorly. The larger lateral talar articular surface is triangular and vertically concave, while the articular surface on the lateral malleolus is reciprocally curved. Posteriorly, the edge between the trochlear and fibular articular surfaces of the talus is bevelled to a narrow, flat triangular area that articulates with the inferior transverse tibiofibular ligament (Fig. 84.13A); all surfaces are contiguous. The bones are held together by a fibrous capsule, and by medial (deltoid), anterior and posterior talofibular and calcaneofibular ligaments.
The ligaments of the talocrural joint are the medial and lateral collateral ligaments.
Medial collateral ligament (deltoid ligament)
The medial collateral ligament (deltoid ligament) is a strong, triangular band, attached to the apex and the anterior and posterior borders of the medial malleolus (Fig. 84.13B). Of its superficial fibres, the anterior (tibionavicular) pass forwards to the navicular tuberosity, behind which they blend with the medial margin of the plantar calcaneonavicular ligament; inter-mediate (tibiocalcaneal) fibres descend almost vertically to the entire length of the sustentaculum tali; posterior fibres (posterior tibiotalar) pass posterolaterally to the medial side of the talus and its medial tubercle. The deep fibres (anterior tibiotalar) pass from the tip of the medial malleolus to the non-articular part of the medial talar surface. The ligament is crossed by the tendons of tibialis posterior and flexor digitorum longus. It is rarely injured alone, and when torn, is commonly associated with a fracture of the distal fibula. Chronic instability is rare.
The lateral ligament has three discrete parts. The anterior talofibular ligament extends anteromedially from the anterior margin of the fibular malleolus to the talus, attached in front of its lateral articular facet and to the lateral aspect of its neck (Fig. 84.13C). The posterior talofibular ligament runs almost horizontally from the distal part of the lateral malleolar fossa to the lateral tubercle of the posterior talar process (Fig. 84.13A); a ‘tibial slip’ of fibres connects it to the medial malleolus. The calcaneofibular ligament, a long cord, runs from a depression anterior to the apex of the fibular malleolus to a tubercle on the lateral calcaneal surface and is crossed by the tendons of fibularis longus and brevis (Fig. 8.13A,C). The lateral ligament complex is injured most commonly with inversion sprains, often during sport; the posterior talofibular ligament is almost always spared. Although the resulting increased laxity is tolerated in most cases, some require surgical reconstruction.
For a comprehensive account of the innervation of the ankle joint (and other foot joints) see Gardner & Gray (1968).
Anteriorly, from medial to lateral, are tibialis anterior, extensor hallucis longus, the anterior tibial vessels, deep fibular nerve, extensor digitorum longus and fibularis tertius; posteromedially from medial to lateral, are tibialis posterior, flexor digitorum longus, the posterior tibial vessels, tibial nerve, flexor hallucis longus; in the groove behind the lateral malleolus are the tendons of fibularis longus and brevis. The tendon of fibularis brevis lies anterior to the tendon of fibularis longus at this level (Fig. 84.14). The long saphenous vein and saphenous nerve cross the ankle joint medial to the tendon of tibialis anterior and anterior to the medial malleolus, the nerve lying posterior to the vein.
All of the above structures are at risk during surgery on the ankle: the main structures at risk are the neurovascular structures anteriorly and posteromedially. Branches of the superficial fibular nerve are at risk on the anterolateral aspect of the ankle, particularly during ankle arthroscopy.
Ankle fractures
Ankle fractures are common and of importance because failure to achieve accurate anatomical alignment in the treatment of ankle fractures often results in significant long term morbidity. Except for very simple and undisplaced fractures, most ankle fractures are associated with a ligamentous injury. The direction and nature of forces applied to the ankle correlate with the fracture pattern and concomitant ligament injury. The Lauge–Hansen classification, although slightly cumbersome, classifies injuries according to two components: the position of the foot at the time of injury and the direction of the force applied. In a supination/adduction type injury, the foot is supinated and an adduction force is applied, resulting in a transverse fracture of the distal fibula (stage 1), followed by an oblique fracture of the medial malleolus (stage 2). In a supination/external rotation type injury, the first structure to rupture is the anterior inferior tibiofibular ligament, followed in order by a spiral fibular fracture, a posterior malleolar fracture and then a medial malleolar fracture (or deltoid ligament rupture). In pronation/abduction injuries, a transverse medial malleolar fracture is followed by rupture of the anterior and posterior inferior tibiofibular ligaments and interosseous ligament, and then by an oblique fibular fracture. Pronation/external rotation injuries commence with a medial malleolar fracture (or deltoid ligament rupture), followed in turn by rupture of the anterior inferior tibiofibular ligament, the interosseous ligament, the interosseous membrane, a high fibular fracture and fracture of the posterior tibial plafond (‘posterior malleolus’).
Inferior (distal) tibiofibular joint
Anterior tibiofibular ligament
The anterior tibiofibular ligament is a flat band which descends laterally between the adjacent margins of the tibia and fibula, anterior to the syndesmosis (Fig. 84.13C). Bassett’s ligament is a variant that presents as a low lying slip of the ligament which is inserted so far distally on the fibula that it may cause irritation of the lateral dome of the talus: it is amenable to arthroscopic removal.
Posterior tibiofibular ligament
The posterior tibiofibular ligament is stronger than the anterior, and is disposed similarly on the posterior aspect of the syndesmosis (Fig. 84.13A). Its distal, deep part is the inferior transverse ligament, a thick band of yellow fibres which crosses from the proximal end of the lateral malleolar fossa to the posterior border of the tibial articular surface almost to the medial malleolus. The ligament projects distal to the bones, in contact with the talus. Its colour reflects its content of yellow elastic fibres.
Talocalcaneal joint
The subtalar joint proper involves the concave posterior calcaneal facet on the posterior part of the inferior surface of the talus and the convex posterior facet on the superior surface of the calcaneus (Fig. 84.15A,B).
Interosseous talocalcaneal ligament
The interosseous talocalcaneal ligament is a broad, flat, bilaminar transverse band in the sinus tarsi (Figs 84.15A; 84.16). It descends obliquely and laterally from the sulcus tali to the calcaneal sulcus. The posterior lamina of the ligament is associated with the talocalcaneal joint, and the anterior lamina with the talocalcaneonavicular joint. Its medial fibres are taut in eversion.
The cervical ligament is just lateral to the tarsal sinus and attached to the superior calcaneal surface (Fig. 84.13C). It is medial to the attachment of extensor digitorum brevis, from where it ascends medially to an inferolateral tubercle on the talar neck (Barclay-Smith 1896). It is considered to be taut in inversion.
Posteromedially, in the region of the posterior aspect of the talocalcaneal joint, and viewed from anterior to posterior, lie the veins on either side of the posterior tibial artery, the tibial nerve and the tendon of flexor hallucis longus. These neurovascular structures are at risk in posteromedial approaches to the ankle and talocalcaneal joints. On the lateral side, the tendon of fibularis brevis lies anterior to the tendon of fibularis longus, both passing behind the fibular malleolus in proximity to the talocalcaneal joint. The sural nerve lies just posterior to the fibular tendons and is at risk during lateral exposure of the joint.
Talocalcaneonavicular joint
An eloquent account of the talocalcaneonavicular joint was provided by Barclay-Smith in 1896. In terms of function, and in clinical practice, it is helpful to regard this complex joint as comprising two articulations, i.e. the anterior part of the ‘subtalar’ joint and the talonavicular joint. It is a compound, multiaxial articulation.
The talonavicular ligament is a broad, thin band (Fig. 84.13B,C). It connects the dorsal surfaces of the neck of the talus and the navicular, and is covered by extensor tendons. The plantar calcaneonavicular ligament and the calcaneonavicular part of the bifurcate ligament (Fig. 84.13C) are the plantar and lateral ligaments of the joint, respectively. Although the calcaneus and navicular do not articulate directly, they are connected by calcaneonavicular and plantar calcaneonavicular ligaments.
Plantar calcaneonavicular (spring) ligament
The plantar calcaneonavicular (spring) ligament is a broad, thick band connecting the anterior margin of the sustentaculum tali to the plantar surface of the navicular (Figs 84.13B; 84.15A; 84.17). It ties the calcaneus to the navicular below the head of the talus as part of its articular cavity and it sustains the medial longitudinal arch of the foot. The dorsal surface of the ligament has a triangular fibrocartilaginous facet on which part of the talar head rests (Fig. 84.15A). Its plantar surface is supported medially by the tendon of tibialis posterior and laterally by the tendons of flexors hallucis longus and digitorum longus; its medial border is blended with the anterior superficial fibres of the medial (deltoid) ligament. The calcaneonavicular ligament is described below as the medial band of the bifurcate ligament.
Calcaneocuboid joint
The fibrous capsule is thickened dorsally as the dorsal calcaneocuboid ligament. The synovial cavity of this joint is separate, and does not communicate with those of other tarsal articulations (Fig. 84.6).
The bifurcate ligament is a strong Y-shaped band (Fig. 84.13C). It is attached by its stem proximally to the anterior part of the upper calcaneal surface, and distally it divides into calcaneocuboid and calcaneonavicular parts. The (lateral) calcaneocuboid ligament extends to the dorsomedial aspect of the cuboid, forming a main bond between the two rows of tarsal bones; the (medial) calcaneonavicular ligament is attached to the dorsolateral aspect of the navicular.
The long plantar ligament is the longest ligament associated with the tarsus (Figs 84.13C; 84.17; 84.18). It extends from the plantar surface of the calcaneus (anterior to the processes of its tuberosity) and from its anterior tubercle, to the ridge and tuberosity on the plantar surface of the cuboid. Deep fibres are attached to the cuboid and more superficial fibres continue to the bases of the second to fourth, and sometimes fifth, metatarsals. This ligament, together with the groove on the plantar surface of the cuboid, makes a tunnel for the tendon of fibularis longus. It is a most powerful factor limiting depression of the lateral longitudinal arch.
Plantar calcaneocuboid ligament
This short plantar ligament (Fig. 84.17) is deeper than the long plantar ligament, from which it is separated by areolar tissue. It is a short, wide band of great strength, stretching from the anterior calcaneal tubercle and the depression anterior to it, to the adjoining part of the plantar surface of the cuboid; it also sustains the lateral longitudinal arch.
Naviculocuneiform joints
The naviculocuneiform joint is a compound joint, often described as plane.
Cuboideonavicular joint
The cuboideonavicular joint is usually a fibrous joint, the bones being connected by dorsal, plantar and interosseous ligaments. This syndesmosis is often replaced by a synovial joint that is almost plane; its articular capsule and synovial lining are continuous with that of the naviculocuneiform joint. The dorsal ligament extends distolaterally, the plantar nearly transversely from the cuboid to the navicular. The interosseous ligament is made of strong transverse fibres and connects non-articular parts of adjacent surfaces to the two bones (Fig. 84.13C).
Intercuneiform and cuneocuboid joints
The intercuneiform and cuneocuboid joints are all synovial and approximately plane or slightly curved. Their articular capsules and synovial linings are continuous with those of the naviculocuneiform joints. The bones are connected by dorsal, plantar and interosseous ligaments (Fig. 84.13B,C; 84.15A).
Tarsometatarsal articulations
Tarsometatarsal articulations are approximately plane synovial joints.
The first metatarsal articulates with the medial cuneiform; the second is recessed between the medial and lateral cuneiforms and articulates with the intermediate cuneiform; the third articulates with the lateral cuneiform; the fourth articulates with the lateral cuneiform and the cuboid; the fifth articulates with the cuboid. The joints are approximately on a line from the tubercle of the fifth metatarsal to the tarsometatarsal joint of the hallux, except for that between the second metatarsal and intermediate cuneiform, which is 2–3 mm proximal (Figs 84.5A; 84.11).
Interosseous cuneometatarsal ligaments
There are three interosseous cuneometatarsal ligaments. One (the strongest) passes from the lateral surface of the medial cuneiform to the adjacent angle of the second metatarsal (Fig. 84.17). Known as Lisfranc’s ligament, it is crucial to the stability of the tarsometatarsal joint complex. Disruption of this ligament can lead to instability and deformity and subsequent degenerative changes. A second ligament connects the lateral cuneiform to the adjacent angle of the second metatarsal: it does not divide the joint between the second metatarsal and lateral cuneiform and is inconstant. A third ligament connects the lateral angle of the lateral cuneiform to the adjacent fourth metatarsal base.
Intermetatarsal joints
Dorsal and plantar intermetatarsal ligaments
As with their dorsal counterparts, the plantar intermetatarsal ligaments are longitudinal, oblique or transverse and they vary considerably in both number and organization. The plantar ligaments are significantly stronger than the corresponding dorsal ligaments. The strongest is the second oblique plantar ligament, which connects the medial cuneiform to the second and third bases (Fig. 84.17).
Metatarsophalangeal articulations
Deep transverse metatarsal ligaments
The deep transverse metatarsal ligaments are four short, wide, flat bands that unite the plantar ligaments of adjoining metatarsophalangeal joints. The interossei are dorsal to, and the lumbricals, digital vessels and nerves are plantar to, these ligaments. They resemble the deep transverse metacarpal ligaments except that, in the foot, there is a deep transverse metacarpal ligament between the plantar ligament of the second metatarsophalangeal joint and that of the hallucal metatarsophalangeal joint (Fig. 84.17).
The types of movements that occur at these joints are like those that occur at the corresponding joints in the hand, but the range of movement is quite different. In contrast to the metacarpophalangeal joints, the range of active extension that can occur at the metatarsophalangeal joints (50–60°) is greater than that of flexion (30–40°): this is an adaptation to the needs of walking, and is most marked in the hallucal joint, where flexion is a few degrees while extension may reach 90°. When the foot is on the ground, metatarsophalangeal joints are already extended to at least 25° because the metatarsals incline proximally in the longitudinal arches of the foot (Fig. 84.8A). The range of passive movements in these joints is 90° (extension) and 45° (flexion), according to Kapandji (1974). The following muscles produce movements at the metatarsophalangeal joints:
Interphalangeal articulations
Interphalangeal articulations are almost pure hinge joints, in which the trochlear surfaces on the phalangeal heads articulate with reciprocally curved surfaces on adjacent phalangeal bases. Each has an articular capsule and two collateral ligaments, as occurs in the metatarsophalangeal joints. The plantar surface of the capsule is a thickened fibrous plate, like the plantar metatarsophalangeal ligaments, and is often termed the plantar ligament.
ARCHES OF THE FOOT
MEDIAL LONGITUDINAL ARCH
The medial margin of the foot arches up between the heel proximally and the medial three metatarsophalangeal joints to form a visible arch (Fig. 84.8A). It is made up of the calcaneus, talar head, navicular, the three cuneiforms and the medial three metatarsals. The posterior and anterior pillars are the posterior part of the inferior calcaneal surface and the three metatarsal heads, respectively. The bones themselves contribute little to the stability of the arch, whereas the ligaments contribute significantly. The most important ligamentous structure is the plantar aponeurosis, which acts as a tie beam between the supporting pillars (Hicks 1953). Dorsiflexion, especially of the hallux, draws the two pillars together, thus heightening the arch: the so-called ‘windlass’ mechanism. Next in importance is the spring ligament, which supports the head of the talus. If this ligament fails, the navicular and calcaneus separate, allowing the talar head, which is the highest point of the arch, to descend. The talocalcaneal ligaments and the anterior fibres of the deltoid ligament, from the tibia to the navicular, also contribute to the stability of the arch.
LATERAL LONGITUDINAL ARCH
The lateral longitudinal arch is much less pronounced arch than the medial one. The bones making up the lateral longitudinal arch are the calcaneus, the cuboid and the fourth and fifth metatarsals: they contribute little to the arch in terms of stability (Fig. 84.8B). The pillars are the calcaneus posteriorly, and the lateral two metatarsal heads, anteriorly. Ligaments play a more important role in stabilizing the arch, especially the lateral part of the plantar aponeurosis and the long and short plantar ligaments. However, the most important contribution to the maintenance of the lateral arch is made by the tendon of fibularis longus. The lateral two tendons of flexor digitorum longus (and flexor accessorius), the muscles of the first layer (lateral half of flexor digitorum brevis and abductor digiti minimi) and fibularis brevis and peronius tertius also contribute to the maintenance of the lateral longitudinal arch.
TRANSVERSE ARCH
The bones involved in the transverse arch are the bases of the five metatarsals, the cuboid and the cuneiforms (Fig. 84.5B). The intermediate and lateral cuneiforms are wedge-shaped and thus adapted to maintenance of the transverse arch. The stability of the arch is mainly provided by the ligaments, which bind the cuneiforms and the metatarsal bases, and also by the tendon of fibularis longus, which tends to approximate the medial and lateral borders of the foot. A shallow arch is maintained at the metatarsal heads by the deep transverse ligaments, transverse fibres that bind together the digital slips of the plantar aponeurosis, and, to a lesser extent, by the transverse head of adductor hallucis.
PES PLANUS AND PES CAVUS
In Charcot–Marie–Tooth disease, an overactive fibularis longus leads to plantar hyperflexion of the first metatarsal. To keep the forefoot in contact with the ground, the affected individual develops a progressive compensatory hindfoot varus. If ignored, the hindfoot varus, which is initially flexible, becomes fixed. The Coleman block test involves standing with the lateral part of the forefoot on a wooden block (Coleman & Chesnut 1977). This effectively balances the forefoot supination and the hindfoot deformity corrects itself spontaneously, provided the hindfoot varus is flexible. If the hindfoot is still flexible, surgery need only address the forefoot supination. If fixed, however, the hindfoot deformity also needs to be surgically corrected.
MUSCLES
The muscles acting on the foot may be divided into extrinsic and intrinsic groups.
EXTRINSIC MUSCLES
The extrinsic muscles are described in Chapter 83. Their tendons cross the ankle, and move and stabilize this joint. Distally, the tendons also act on the small joints of the foot and help to stabilize these joints. The muscles can be grouped according to their arrangement in the leg. The extensors arise in the anterior compartment of the leg and their tendons pass anterior to the ankle, where they are bound down by the extensor retinacula. The lateral group arises in the relatively narrow lateral compartment of the leg and their tendons pass posterior to the lateral malleolus, bound down by the fibular retinacula. The flexors arise in the posterior compartment of the leg and their tendons pass posterior to the ankle, where the tendons of the superficial group of flexors are inserted into the calcaneus (see below) and the tendons of the deep group of flexors are bound down by the flexor retinaculum.
Anterior group
Tibialis anterior, extensor hallucis longus, extensor digitorum longus and fibularis tertius are all described in Chapter 83.
Posterior group
Superficial group
Gastrocnemius and soleus are described in Chapter 83; the calcaneal tendon is described below.
Calcaneal (Achilles) tendon
The calcaneal tendon is the thickest and strongest tendon in the human body (see Fig. 82.3). It is approximately 15 cm long, and begins near the middle of the calf, although its anterior surface receives muscle fibres from soleus almost to its lower end. It gradually becomes more rounded until approximately 4 cm above the calcaneus, below this level it expands and becomes attached to the midpoint of the posterior surface of the calcaneus. The fibres of the calcaneal tendon are not aligned strictly vertically and they display a variable degree of spiralization (Cummins et al 1946).
The blood supply to the calcaneal tendon is poor: the predominant artery is a recurrent branch of the posterior tibial artery which mainly supplies peritendinous tissues (Taylor & Razaboni 1994). There is an additional supply proximally from intramuscular arterial branches and distally from the calcaneus. The vascularity of the mid-substance of the tendon is relatively poor, although some blood enters the tendon at this point through its anterior surface. During surgery, the paratenon is therefore best left intact in this region.
INTRINSIC MUSCLES
Plantar muscles of the foot: first layer
This superficial layer includes abductor hallucis, abductor digiti minimi and flexor digitorum brevis (Fig. 84.19A). All three extend from the calcaneal tuberosity to the toes, and all assist in maintaining the concavity of the foot.
Abductor hallucis
Abductor hallucis lies along the medial border of the foot and covers the origins of the plantar vessels and nerves (Fig. 84.20).
Abductor hallucis is supplied by the medial malleolar network, medial calcaneal branches of the lateral plantar artery (Fig. 84.9), the medial plantar artery (directly and via superficial and deep branches), the first plantar metatarsal artery and perforators from the plantar arterial arch.
Flexor digitorum brevis
Flexor digitorum brevis arises by a narrow tendon from the medial process of the calcaneal tuberosity, from the central part of the plantar aponeurosis, and from the intermuscular septa between it and adjacent muscles. It divides into four tendons, which pass to the lateral four toes; the tendons enter digital tendon sheaths accompanied by the tendons of flexor digitorum longus, which lie deep to them. At the bases of the proximal phalanges, each tendon divides around the corresponding tendon of flexor digitorum longus; the two slips then reunite and partially decussate, forming a tunnel through which the tendon of flexor digitorum longus passes to the distal phalanx. The short flexor tendon divides again and attaches to both sides of the shaft of the middle phalanx. The way in which the tendons of flexor digitorum brevis divide and attach to the phalanges is identical to that of the tendons of flexor digitorum superficialis in the hand. The slip to a given toe may be absent, or it may be replaced by a small muscular slip from the long flexor tendon or from flexor accessorius. Conversely, the slip may be joined by a second, supernumerary slip.
Flexor digitorum brevis lies immediately deep to the central part of the plantar aponeurosis (Fig. 84.19A). Its deep surface is separated from the lateral plantar vessels and nerves by a thin layer of fascia.
Abductor digiti minimi
Abductor digiti minimi arises from both processes of the calcaneal tuberosity, from the plantar surface of the bone between them, from the plantar aponeurosis and from the intermuscular septum between the muscle and flexor digitorum brevis. Its tendon glides in a smooth groove on the plantar surface of the base of the fifth metatarsal and is attached, with flexor digiti minimi brevis, to the lateral side of the base of the proximal phalanx of the fifth toe; hence it is more a flexor than an abductor. Some of the fibres arising from the lateral calcaneal process usually reach the tip of the tuberosity of the fifth metatarsal (Figs 84.8B; 84.11) and may form a separate muscle, abductor ossis metatarsi digiti quinti. An accessory slip from the base of the fifth metatarsal is not infrequent.
Abductor digiti minimi lies along the lateral border of the foot, and its medial margin is related to the lateral plantar vessels and nerve (Fig. 84.20).
Abductor digiti minimi is supplied by the medial and lateral plantar arteries (Fig. 84.20; see Fig. 84.24A), the plantar digital artery to the lateral side of minimus, branches from the plantar arch, the fourth plantar metatarsal artery and end twigs of the arcuate and lateral tarsal arteries (Fig. 84.9).
Plantar muscles of the foot: second layer
The second layer consists of flexor digitorum accessorius and four lumbrical muscles. The tendons of flexor hallucis longus and flexor digitorum longus run in the same plane as the muscles of the second layer (Fig. 84.19B): flexor hallucis longus and flexor digitorum longus are described in Chapter 83.
Flexor tendon sheaths
The terminations of the tendons of the long and short flexor muscles are contained in osseo-aponeurotic canals similar to those which occur in the fingers. These canals are bounded above by the phalanges, and below by fibrous bands, the digital fibrous sheaths, which arch across the tendons and attach on either side to the margins of the phalanges (Fig. 84.19B). Along the proximal and intermediate phalanges, the fibrous bands are strong, and the fibres are transverse (anular part); opposite the joints they are much thinner and the fibres decussate (cruciform part). Each osseo-aponeurotic canal has a synovial lining, which is reflected around its tendon; within this sheath, vincula tendinum are arranged as they are in the fingers.
Flexor digitorum accessorius
Flexor digitorum accessorius (Fig. 84.19B), also known as quadratus plantae, arises by two heads, with the long plantar ligament situated deeply in the interval between the two heads. The medial head is larger and more fleshy and is attached to the medial concave surface of the calcaneus, below the groove for the tendon of flexor hallucis longus. The lateral head is flat and tendinous and is attached to the calcaneus distal to the lateral process of the tuberosity, and to the long plantar ligament. The muscle belly inserts into the tendon of flexor digitorum longus at the point where it is bound by a fibrous slip to the tendon of flexor hallucis longus and where it divides into its four tendons.
Lumbrical muscles
The lumbrical muscles are four small muscles (numbered from the medial side of the foot) which are accessory to the tendons of flexor digitorum longus (Fig. 84.19A,B). They arise from these tendons as far back as their angles of separation, each springing from the sides of two adjacent tendons, except for the first lumbrical, which arises only from the medial border of the first tendon. The muscles end in tendons that pass distally on the medial sides of the four lesser toes and are attached to the dorsal digital expansions on their proximal phalanges.
Plantar muscles of the foot: third layer
The third layer of the foot contains the shorter intrinsic muscles of the hallux and minimus, i.e. flexor hallucis brevis, adductor hallucis and flexor digiti minimi brevis (Fig. 84.21).
Flexor hallucis brevis
Flexor hallucis brevis has a bifurcate tendon of origin (Figs 84.19A,B; 84.21). The lateral limb arises from the medial part of the plantar surface of the cuboid, posterior to the groove for the tendon of fibularis longus, and from the adjacent part of the lateral cuneiform. The medial limb has a deep attachment directly continuous with the lateral division of the tendon of tibialis posterior, and a more superficial attachment to the middle band of the medial intermuscular septum. The belly of the muscle divides into medial and lateral parts, the twin tendons of which are attached to the sides of the base of the proximal phalanx of the hallux. The medial part blends with the tendon of abductor hallucis, and the lateral with that of adductor hallucis, as they reach their terminations.
Adductor hallucis
Adductor hallucis arises by oblique and transverse heads (Figs 84.19B; 84.21). The oblique head springs from the bases of the second, third and fourth metatarsal bones, and from the fibrous sheath of the tendon of fibularis longus. The transverse head, a narrow, flat fasciculus, arises from the plantar metatarsophalangeal ligaments of the third, fourth and fifth toes (sometimes only from the third and fourth), and from the deep transverse metatarsal ligaments between them. The oblique head has medial and lateral parts. The medial part blends with the lateral part of flexor hallucis brevis and is attached to the lateral sesamoid bone of the hallux. The lateral part joins the transverse head and is also attached to the lateral sesamoid bone and directly to the base of the first phalanx of the hallux. There is no phalangeal attachment for the transverse part of the muscle; fibres that fail to reach the lateral sesamoid bone are attached with the oblique part.
Flexor digiti minimi brevis
Flexor digiti minimi brevis arises from the medial part of the plantar surface of the base of the fifth metatarsal bone, and from the sheath of fibularis longus (Figs 84.19B; 84.21). It has a distal tendon that inserts into the lateral side of the base of the proximal phalanx of the minimus; this tendon usually blends laterally with that of abductor digiti minimi. Occasionally, some of its deeper fibres extend to the lateral part of the distal half of the fifth metatarsal bone, constituting what may be described as a distinct muscle, opponens digiti minimi.
Plantar muscles of the foot: fourth layer
The fourth layer of muscles of the foot consists of the plantar and dorsal interossei and the tendons of tibialis posterior and fibularis longus (tibialis posterior and fibularis longus are described in Ch. 83). The interossei resemble their counterparts in the hand except that, when describing adduction and abduction of the toes, the axis of reference is a longitudinal axis corresponding to the shaft of the 2nd metatarsal (unlike the hand where reference is made to the long axis of the third metacarpal).
Dorsal interossei
The dorsal interossei (Fig. 84.22A) are situated between the metatarsal bones. They consist of four bipennate muscles, each arising by two heads from the sides of the adjacent metatarsal bones. Their tendons are attached to the bases of the proximal phalanges and to the dorsal digital expansions. The first inserts into the medial side of the second toe; the other three pass to the lateral sides of the second, third and fourth toes.
Between the heads of each of the three lateral muscles, there is an angular space through which a perforating artery passes to the dorsum of the foot. Between the heads of the first muscle, the corresponding space transmits the terminal part of the dorsalis pedis artery to the sole (see Fig. 84.1).
Plantar interossei
There are three plantar interossei (Fig. 84.22B). They lie below, rather than between, the metatarsal bones, and each is connected to one metatarsal bone only. They arise from the bases and medial sides of the third, fourth and fifth metatarsal bones, and insert into the medial sides of the bases of the proximal phalanges of the numerically corresponding toes, and into their dorsal digital expansions.
Extensor muscles of the foot
Extensor digitorum brevis and extensor hallucis brevis
Extensor digitorum brevis (Fig. 84.2A) is a thin muscle which arises from the distal part of the superolateral surface of the calcaneus in front of the shallow lateral groove for fibularis brevis, from the interosseous talocalcaneal ligament, and from the deep surface of the stem of the inferior extensor retinaculum. It slants distally and medially across the dorsum of the foot, and ends in four tendons. The medial part of the muscle is usually a more or less distinct slip, ending in a tendon that crosses the dorsalis pedis artery superficially to insert into the dorsal aspect of the base of the proximal phalanx of the hallux; this slip is sometimes termed extensor hallucis brevis. The other three tendons attach to the lateral sides of the tendons of extensor digitorum longus for the second, third and fourth toes.
VASCULAR SUPPLY
ARTERIES
Dorsalis pedis artery
The dorsalis pedis artery is the dorsal artery of the foot (Figs 84.1; 84.23), and is the continuation of the anterior tibial artery distal to the ankle. It passes to the proximal end of the first intermetatarsal space, where it turns into the sole between the heads of the first dorsal interosseous to complete the plantar arch, and provides the first plantar metatarsal artery.
Branches
The dorsalis pedis artery gives off the tarsal, arcuate and first dorsal metatarsal arteries.
There are two tarsal arteries, lateral and medial (Fig. 84.23). They arise as the dorsalis pedis artery crosses the navicular. The lateral runs laterally under extensor digitorum brevis; it supplies this muscle and the tarsal articulations, and anastomoses with branches of the arcuate, anterior lateral malleolar and lateral plantar arteries, and the perforating branch of the fibular artery. Two or three medial tarsal arteries ramify on the medial border of the foot and join the medial malleolar network.
The arcuate artery (Fig. 84.23) arises near the medial cuneiform, passes laterally over the metatarsal bases, deep to the tendons of the digital extensors, and anastomoses with the lateral tarsal and plantar arteries. It supplies the second to fourth dorsal metatarsal arteries, running distally superficial to the corresponding dorsal interossei, and divides into two dorsal digital branches for the adjoining toes in the interdigital clefts. Proximally, in the interosseous spaces, these branches receive proximal perforating branches from the plantar arch. Distally, they are joined by distal perforating branches from the plantar metatarsal arteries. The fourth dorsal metatarsal artery sends a branch to the lateral side of the fifth toe.
First dorsal metatarsal artery
The first dorsal metatarsal artery (Fig. 84.23) arises just before the dorsalis pedis artery enters the sole. It runs distally on the first dorsal interosseous and divides at the cleft between the first and second toes. One branch passes under the tendon of extensor hallucis longus and supplies the medial side of the hallux; the other bifurcates to supply the adjoining sides of the hallux and the second toe.
Plantar arch
The plantar arch (Fig. 84.24) is deeply situated, extending from the fifth metatarsal base to the proximal end of the first interosseous space. Convex distally, it is plantar to the bases of the second to fourth metatarsal bones and corresponding interossei, but dorsal to the oblique part of adductor hallucis.
Branches
The plantar arch gives off three perforating and four plantar metatarsal branches, and numerous branches that supply the skin, fasciae and muscles in the sole. Three perforating branches ascend through the proximal ends of the second to fourth intermetatarsal spaces, between the heads of dorsal interossei, and anastomose with the dorsal metatarsal arteries. Four plantar metatarsal arteries extend distally between the metatarsal bones in contact with the interossei (Fig. 84.24). Each divides into two plantar digital arteries, supplying the adjacent digital aspects. Near its division, each plantar metatarsal artery sends a distal perforating branch dorsally to join a dorsal metatarsal artery. The first plantar metatarsal artery springs from the junction between the lateral plantar and dorsalis pedis arteries, and sends a digital branch to the medial side of the hallux. The lateral digital branch for the fifth toe arises directly from the lateral plantar artery near the fifth metatarsal base. Haemorrhage from the plantar arch is difficult to stem, because of the depth of the vessel and its important close relations.
Posterior tibial artery
Branches
The medial plantar artery is the smaller terminal branch of the posterior tibial artery (Fig. 84.24). It arises midway between the medial malleolus and the medial calcaneal tubercle and passes distally along the medial side of the foot, with the medial plantar nerve lateral to it. At first deep to abductor hallucis, it runs distally between abductor hallucis and flexor digitorum brevis, supplying both. Near the first metatarsal base, when its calibre is already diminished as a result of supplying numerous muscular branches, it is further diminished by a superficial stem. It passes to the medial border of the hallux, where it anastomoses with a branch of the first plantar metatarsal artery. Its superficial stem then trifurcates and supplies three superficial digital branches that accompany the digital branches of the medial plantar nerve and join the first to third plantar metatarsal arteries.
The lateral plantar artery is the larger terminal branch of the posterior tibial artery (Fig. 84.24). It passes distally and laterally to the fifth metatarsal base; the lateral plantar nerve is medial. The plantar nerves lie between the plantar arteries. Turning medially, with the deep branch of the nerve, it gains the interval between the first and second metatarsal bases, and unites with the dorsalis pedis artery to complete the plantar arch. As it passes laterally, it is first between the calcaneus and abductor hallucis, then between flexor digitorum brevis and flexor accessorius. Running distally to the fifth metatarsal base, it passes between flexor digitorum brevis and abductor digiti minimi and is covered by the plantar aponeurosis, superficial fascia and skin.
DEEP AND SUPERFICIAL VENOUS SYSTEMS IN THE FOOT
The principal named superficial veins are the great and small saphenous. Their numerous tributaries are mostly (but not wholly) unnamed; named vessels will be noted (see Fig. 79.9A,B). As in the upper limb, the vessels will be described centripetally from peripheral to major drainage channels.
INNERVATION OF THE FOOT
Tibial nerve
The branches of the tibial nerve that innervate structures in the ankle and foot are articular, muscular, sural, medial calcaneal and medial and lateral plantar nerves. The course and distribution of the tibial nerve in the calf are described in Chapter 83.
Medial plantar nerve
The common digital branches of the medial plantar nerve are distributed in a manner similar to those of the median nerve, as are the motor branches of the two nerves. In the hand, the median nerve supplies abductor and flexor pollicis brevis, opponens pollicis and the first and second lumbricals. An opponens is absent in the foot, but abductor hallucis, flexor hallucis brevis and the first lumbrical are all supplied by the medial plantar nerve. Flexor digitorum brevis and flexor digitorum superficialis (innervated by the median nerve) correspond, but the innervation of the second lumbrical of the foot does not mirror the arrangement in the hand.
Lateral plantar nerve
The lateral plantar nerve supplies the skin of the fifth toe, the lateral half of the fourth toe, and most of the deep muscles of the foot (Fig. 84.20). Its distribution therefore closely resembles that of the ulnar nerve in the hand. It passes laterally forwards medial to the lateral plantar artery, towards the tubercle of the fifth metatarsal. Next, it passes between flexores digitorum brevis and accessorius, and ends between flexor digiti minimi brevis and abductor digiti minimi by dividing into superficial and deep branches. Before division, it supplies flexor digitorum accessorius and abductor digiti minimi and gives rise to small branches that pierce the plantar fascia to supply the skin of the lateral part of the sole (Fig. 84.25). The superficial branch splits into two common plantar digital nerves: the lateral supplies the lateral side of the fifth toe, flexor digiti minimi brevis and the two interossei in the fourth intermetatarsal space; the medial connects with the third common plantar digital branch of the medial plantar nerve and divides into two to supply the adjoining sides of the fourth and fifth toes. The deep branch accompanies the lateral plantar artery deep to the flexor tendons and adductor hallucis and supplies the second to fourth lumbricals, adductor hallucis and all the interossei (except those of the fourth intermetatarsal space). Branches to the second and third lumbricals pass distally deep to the transverse head of adductor hallucis, and curl round its distal border to reach them.
BIOMECHANICS OF STANDING, WALKING AND RUNNING
STANDING
When considering the distribution of load in the lower limb, a knowledge of limb alignment is important. The ‘anatomic axis’ is produced by lines passing along the shafts of the femur and tibia, and is therefore angled at the knee by 5° to 7°. The ‘mechanical axis’ is a straight line connecting the centres of the femoral head and ankle. Exactly where the line passes through the knee has yet to be defined: for convenience it is usually taken to pass through the centre, but may well lie more medially. For the tibia, the lines of the mechanical and anatomic axes coincide. Whilst the weight of the body acts in a vertical line from the centre of gravity to the ground, the line of the mechanical axis of the lower limbs is inclined 3° to this in normal stance, with the ankles closer than hips.
PROPULSION
Walking
In walking, each foot is on the ground (stance phase) for approximately 60% of the stride, and off the ground (swing phase) for approximately 40% (Fig. 84.26). Thus single-support phases (one foot on the ground) alternate with double-support phases (two feet on the ground). The knee is straight at heel strike and remains nearly straight (10–30°) for most of the stance phase of that leg, bending more only immediately before toe-off. During the swing phase the knee flexes to a maximum of 60° at mid-swing.
Stance phase starts with ‘heel strike’. With the foot still planted in front of the body, ‘foot flat’ is reached, and becomes ‘mid-stance’ when the body comes to be directly above the planted foot. The heel then rises as the contralateral foot makes contact with the ground (the ‘double stance’ phase). The last event of stance is ‘toe off ’ when the ‘swing phase’ starts. Early in the stance phase, while it is ‘foot flat’ in front of the trunk, the foot pushes downwards and forwards on the ground, decelerating the body as well as supporting it. Later, when the foot is behind the trunk, it pushes downwards and backwards, re-accelerating the body (Fig. 84.26). The speed, and therefore the kinetic energy, of the body passes through a maximum in each double-support phase, and a minimum in each single-support phase.
The function of arm swinging in walking is not fully understood but it aids postural stability. For further discussion of standing and walking see Alexander (1992).
Development of walking
The average child sits at 6 months, crawls at 9 months, walks with support at 12 months, and without support at 18 months. The characteristic early gait matures rapidly and is similar to that of the adult by 3 years. Some minor changes occur up to 7 years which are largely a reflection of neurological development, but are also related to stature. Early gait is jerky, unsteady, and wide-based. The arms are held abducted at the shoulder and initially flexed at the elbows. Initial ground contact varies, and heel-toe, whole foot, and toe-heel are all possible. Generally a plantarflexed posture is adopted which contrasts with the adult pattern. In adults, heel strike is accompanied by a straight knee which then flexes. A child strikes the ground with a flexed knee which is then extended in response to weight-bearing, and a short time is spent in single leg stance (Fig. 84.27). Maturation is associated with diminution of base width, and increase in step length and velocity. The earliest changes are development of heel-strike, knee flexion during stance, and reciprocal upper limb swing. The period of single leg stance increases.
Running
Walking involves dual-support phases, but in running each foot is on the ground for 40% (jogging) to 27% (sprinting) of the stride, so there is an aerial phase, the ‘double float’ phase, when neither foot is on the ground. The faster the subject runs, the shorter the stance phase: world-class sprinters spend approximately 22% of the gait cycle in stance. During each aerial phase the body rises and then falls under gravity, which means that its height and potential energy are maximal in the middle of this phase and minimal at mid-stance, when, in marked contrast to walking, the knee of the supporting leg bends. Walking has been described as ‘controlled falling’, as the centre of gravity falls from a peak in mid-stance to a low during double support. The analogy that is used for running is an individual on a ‘pogo stick’. The runner propels himself from a low point in mid-stance to a peak during double float. During walking, efficiency is maintained by the interchange of potential and kinetic energy. During running, when potential and kinetic energies are in phase, efficiency is maintained in two ways: storage and release of potential energy in the elastic structure of tendons, and transfer of energy between limb segments via muscles which cross two joints (e.g. rectus femoris and hamstrings). The changes in muscle belly lengths are relatively slight during running. The muscles are acting as tensioners of the tendons, indeed, most of the change of length is produced by the stretch and recoil of the tendons. It has been estimated that, of the kinetic and potential energy lost and regained in each stance phase, 35% is stored temporarily as elastic strain energy in the calcaneal tendon, and 17% in the ligaments of the arch of the foot. Together these springs approximately halve the work required from the muscles.
Movements of the foot
With the foot on the ground, body weight causes some supination and flattening of the longitudinal arches; about one-third of the weight borne by the forefoot is taken by the head of the first metatarsal. When a resting position becomes active, as occurs on starting to walk, the foot is pronated by muscular effort, and the first metatarsal is depressed (the second less so), which accentuates the longitudinal arch to its maximum height (Hicks 1953). Similar changes can be imposed on a weight-bearing foot by active lateral femoral rotation, which is transmitted through the tibia to the talus and entails passive supination of the foot. Medial femoral rotation has an opposite effect. When the foot is grounded and immobile, muscles which move it when it is freely suspended may exert effects on the leg, e.g. the dorsiflexors can then pull the leg forwards at the ankle joint.
The medial longitudinal arch contains the calcaneus, talus, navicular, cuneiform and medial three metatarsal bones. Its summit, at the superior talar articular surface, takes the full thrust from the tibia and passes it backwards to the calcaneus, and forwards through the navicular and cuneiforms to the metatarsals. When the foot is grounded, these forces are transmitted through the three metatarsal heads and calcaneus (especially its tuberosity). The medial arch is higher, and more mobile and resilient than the lateral arch; its flattening progressively tightens the plantar calcaneonavicular ligament and plantar fascia. The lateral arch is adapted to transmit weight and thrust rather than to absorb such forces: the long plantar and plantar calcaneocuboid ligaments tighten as it flattens.
The lateral arch makes contact with the ground more extensively than the medial arch. As the foot flattens, an increasing fraction of load traverses soft tissues which are inferior to the entire arch. The whole lateral border usually touches the ground, whereas the medial border does not. However the medial border is visibly concave, usually even in standing, which explains the familiar outline of human footprints (though this varies with the position of the feet (Fig. 84.28), the development of associated soft tissues, and the nature of the surface). In any activity, as soon as the heel rises, the toes are extended and muscular structures (including the plantar aponeurosis) tighten up in the sole, accentuating the longitudinal arches. It has been suggested that tension diminishes in the deeper plantar ligaments in this phase.
In standing, with only body weight to support, both the intrinsic and extrinsic muscles appear to relax. If the longitudinal arches are allowed to sink as a result of muscular relaxation, the plantar ligaments tie the bones into an arched form. The medial arch is more elevated when the feet are together than when they are apart, i.e. inversion with supination increases as the feet are separated. This medial sag can be countered by voluntary contraction of muscles such as tibialis anterior. Pronation and supination ensure that in standing, whatever the position of the feet, a maximal weight-bearing area is grounded, from the metatarsal heads along the lateral border of the foot to the calcaneus. The twist imparted by pronation (which is partly undone in supination) prompts the likening of the foot to a twisted but resilient plate (Fig. 84.28), where adequate ground contact was ensured whatever the angle between the foot and leg, and adaptable resilience was imparted in standing and progression.
Alexander R McN. The Human Machine. London: Natural History Museum Publications, 1992.
Barclay-Smith E. The astragalo-calcaneo-navicular joint. J Anat Physiol. 1896;30:390-412.
Coleman S, Chesnut W. A simple test for hindfoot flexibility in the cavovarus foot. Clin Orthop. 1977;123:60-62.
Cummins JE, Anson JB, Carr WB, Wright RR, Hauser DWE. The structure of the calcaneal tendon (of Achilles) in relation to orthopedic surgery with additional observations on the plantaris muscle. Surg Gynecol Obstet. 1946;83:107-116.
Gardner E, Gray DJ. The innervation of the joints of the foot. Anat Rec. 1968;161:141-148.
Harris RI, Beath T. Etiology of peroneal spastic flat foot. J Bone Joint Surg [Br]. 1948;30:624-634.
Hicks JH. The mechanics of the foot II. The plantar aponeurosis and the arch. J Anat. 1953;88:25-30.
Jones FW. Structure and Function as Seen in the Foot, 2nd edn., London: Baillière, Tindall and Cox; 1949:63-65.
One of the classic texts on the foot..
Kapandji IA. The Physiology of the Joints. Annotated Diagrams of the Mechanics of the Human Joints, 2nd edn (3 vols translated by LH Honoré: Vol 1 Upper Limb. Vol 2 Lower Limb. Vol 3 The Trunk and the Vertebral Column.). Edinburgh: Churchill Livingstone, 1970–1974.
Sarrafian SK. Anatomy of the Foot and Ankle, Descriptive, Topographic, Functional, 2nd edn. Philadelphia: Lippincott, 1993.
Taylor GI, Razaboni RM. Arteries of the Muscles of the Extremities and the Trunk. St Louis: Quality Medical Publishing Inc, 1994.
Wildenauer F. Die Blutversorgung des Talus. Z Anat Entwicklungsgesch. 1950;115:32-36.