Fig. 3.1
Pathobiology of circulating Angptl4 in nephrotic syndrome (From [21]). Diagram representing the production of circulating Angptl4 protein and its biological effects. The circulating, sialylated form of Angptl4 is secreted from peripheral organs (mostly the skeletal muscle, heart, and adipose tissue) in minimal-change nephrotic syndrome (MCNS), membranous nephropathy (MN), focal and segmental glomerulosclerosis (FSGS), and non-HIV collapsing glomerulopathy (CG). In addition, podocytes in MCNS secrete a hyposialylated form of the protein that remains restricted to the kidney and induces proteinuria [4] and a normosialylated form that enters the circulation. Circulating Angptl4 binds to glomerular endothelial αvβ5 integrin to reduce proteinuria or inactivates endothelium-bound lipoprotein lipase (LPL) in the skeletal muscle, heart, and adipose tissue to reduce the hydrolysis of plasma triglycerides to free fatty acids (FFA), resulting in hypertriglyceridemia. Some Angptl4 and LPL are lost in the urine
3.1.3.2 aP2-Angptl4 Transgenic Rats
Under normal conditions, major Angptl4-secreting tissues in the body are the adipose tissue, skeletal muscle, heart, and liver. These organs secrete a sialylated form of Angptl4 that enters circulation and affects plasma triglyceride levels.
Rats that specifically overexpress Angptl4 from adipose tissue (aP2-Angptl4 transgenic rats) have high levels of circulating sialylated Angptl4 protein. High circulating sialylated Angptl4 derived from these organs is increased in many forms of human nephrotic syndrome, including MCNS, membranous nephropathy, focal and segmental glomerulosclerosis, and collapsing glomerulopathy [21]. The only difference between the other conditions and MCNS is that the podocyte is an additional source of circulating sialylated Angptl4 in MCNS. As discussed later, podocytes produce a combination of hyposialylated and sialylated Angptl4.
aP2-Angptl4 transgenic rats do not develop proteinuria, since circulating sialylated Angptl4 has an anti-proteinuric effect. These transgenic rats have normal glomerular morphology on light and electron microscopy, and they do not have any modification in the GBM charge. They have significant hypertriglyceridemia, since circulating Angptl4 is a potent inhibitor of lipoprotein lipase (LPL), the endothelium-bound enzyme that hydrolyzes triglycerides to release free fatty acids (FFA).
3.1.4 Study of Angptl4 in the PAN Model of Human MCNS
The PAN model is the most commonly used model of MCNS, induced by a single intravenous injection of puromycin aminonucleoside into rats [24]. These rats present some features of the human MCNS: explosive onset of proteinuria, no visible glomerular lesions by light microscopy, foot process effacement by electron microscopy, hypertriglyceridemia and hypercholesterolemia, and loss of GBM charge. However, proteinuria is not selective and is only partially glucocorticoid sensitive [5].
Confocal microscopy shows co-localization of Angptl4 with nephrin, indicating its expression in podocyte. There is low constitutive Angptl4 protein expression in the normal rat and human podocyte. In both human MCNS and PAN model, Angptl4 secreted from podocytes enters the GBM (co-localizes with proteoglycans) and the blood circulation and is also lost in urine.
In the PAN model, upregulation of Angptl4 gene expression and amount of proteinuria induced are dependent on the dose of puromycin aminonucleoside injected into rats. The proteinuria induced in the model is relatively nonselective, since only 66 % of urinary proteins comprise for albumin, compared to about 86 % in human MCNS relapse patient and 92 % in NPHS-Angptl4 transgenic rats. Regarding selectivity of proteinuria, this is not such a good model to study MCNS.
3.1.5 Molecular Mechanisms of Angptl4 in Proteinuria in MCNS: The Role of Sialylation
Two-dimensional electrophoresis gel studies showed that glomeruli from PAN rats express two forms of the Angptl4 protein: a positively charged form migrating at a high isoelectric point (pI) (8–8.5) and a neutral form migrating at pI between 6 and 7. The lack of adequate sialylation in the high-pI form is an important difference between these two forms of Angptl4. NPHS2-Angptl4 transgenic rats similarly express both forms of Angptl4 in glomeruli, making them suitable to further study the role of Angptl4 in MCNS.
In addition to the transgenic rats, two types of stable cell lines overexpressing Angptl4 were developed in mouse glomerular epithelial cells (GEC) and human embryonic kidney cells (HEK293). Cells secrete high-pI form of Angptl4 in the medium from where it can be harvested. Incubation of both cell lines with the naturally occurring sialic acid precursor N-acetyl-D-mannosamine (ManNAc) allows the conversion of the high-pI hyposialylated Angptl4 into the neutral-pI sialylated form within the cells and secretion of sialylated Angptl4 in the supernatant.
The choice of using ManNAc for sialylation-based therapeutics was determined by several factors [25]. First, ManNAc has a neutral charge allowing it to cross cell membranes easily. Once in the cell, it is converted into sialic acid and then incorporated into glycoproteins as Angptl4 [4]. Furthermore, ManNAc enters the sialic acid biosynthesis pathway after the rate-limiting enzymatic step catalyzed by GNE (UDP-N-acetylglucosamine-2-epimerase/N-acetylmannosamine kinase) [26] (Fig. 3.2). This surmounts the intrinsic limitations of the pathway during high-demand states. Second, podocytes are nondividing cells, and therefore, cellular ManNAc content is not divided by cell division. Lastly, orally administrated ManNAc is rapidly lost in the urine (90 % within 4 h). During this process, the podocyte, being the outermost layer of the glomerular filter, gets normal exposure during transit across this filter.
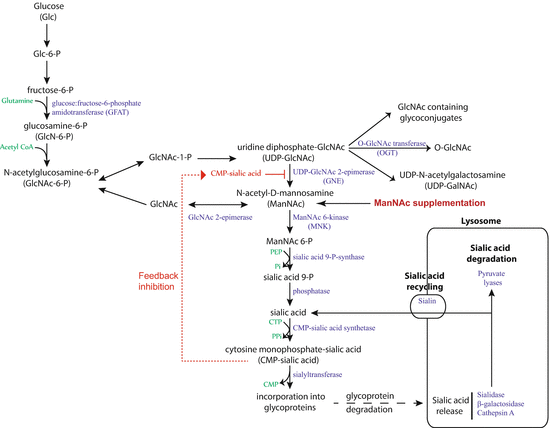
Fig. 3.2
Sialic acid biosynthesis and recycling pathway (From [27]). In humans, sialic acid is synthesized from glucose. The rate-limiting step, catalyzed by UDP-N-acetylglucosamine-2-epimerase/N-acetylmannosamine kinase (GNE), is subject to feedback inhibition. ManNAc is the product of this rate-limiting step, so exogenous ManNAc supplementation enters the pathway after this step. A substantial amount of sialic acid is recycled via the anion transporter sialin following lysosomal degradation of glycoproteins and glycolipids
To answer the question of the biological significance of Angptl4 sialylation, in vivo studies were conducted to verify its effects on albuminuria. Treatment of NPHS2-Angptl4 transgenic rats with daily oral ManNAc supplementation results in an over 40 % decrease in albuminuria from baseline over 12 days. Concomitantly, conversion of significant amounts of high-pI to neutral-pI Angptl4 occurs in the glomeruli of these ManNAc-treated rats compared to untreated rats. The neutral fraction reacts with sialic acid-binding lectin from Sambucus nigra (SNA I), confirming the increase in sialylation of Angptl4 in ManNAc-treated rats. This form of therapy has a significant memory effect, since it took 24 days for the proteinuria to rise back to the level of control-treated NPHS2-Angptl4 rats. To verify the biological effects of ManNAc on sialylation in the glomeruli, the two-dimensional electrophoresis gel patterns of another important protein in the podocyte were studied. Podocalyxin is a structural sialoglycoprotein expressed in podocytes. The non-modification of the overall charge of podocalyxin suggests that podocalyxin was not affected by ManNAc therapy [4].
The lack of sialylation of Angptl4 in MCNS is still under investigation and could have several origins. A simple possible explanation is based on the low constitutive expression of Angptl4 in normal podocytes. Therefore, only small amounts of sialic acid are required by this pathway at baseline. Due to severe and rapid upregulation of Angptl4 expression in experimental MCNS disease (70-fold increase in messenger RNA expression), demand exceeds supply, resulting in the secretion of hyposialylated high-pI protein. Another possible explanation for the lack of sialylation of Angptl4 is that the activity of a class of enzymes called sialyltransferases, which add sialic acid residues to proteins, is decreased in podocytes in MCNS.
3.1.6 Link Between Proteinuria and Hypertriglyceridemia in Nephrotic Syndrome
There are large gaps of knowledge in our understanding of the molecular relationship between proteinuria, the primary driver in nephrotic syndrome, and most of the other components. Only the link between proteinuria and hypertriglyceridemia has been clearly elucidated [21, 27], revealing the intrinsic role of circulating Angptl4 in nephrotic syndrome. This relationship is strongly influenced by the link between FFA and albumin. Albumin is the most abundant plasma protein that circulates as a 69 kDa monomer and serves as a vehicle to transport cations, hormones, and FFA. FFA are a critical energy source for the body and also serve as an important molecular mediator in nephrotic syndrome. FFA can be used as a source of energy by organs like the skeletal muscle and heart [28] or can be recycled by adipose tissue, since it both releases and takes up FFA for storage as triglycerides. These organs also have high expression for LPL, Angptl4, and peroxisome proliferator-activated receptor (PPAR) family members, which regulate Angptl4 expression in response to FFA uptake.
Normal sources of fatty acids include diet, mobilization from adipose tissue, and conversion of excess carbohydrates into fat by the liver. Some fatty acids are coupled with glycerol to form triglycerides (or triacylglycerols) for transport or storage and can be converted back into FFA by lipases. FFA that are not part of triglycerides circulate in the blood, mostly coupled non-covalently with albumin. Each albumin molecule has six high-affinity FFA-binding sites and many low-affinity-binding sites, and up to ten FFA molecules can be bound to an albumin molecule at any given time [29]. Adipose tissue releases FFA into circulation after serial conversion of triglycerides to diglycerides by adipose triglyceride lipase and diglycerides to monoglycerides by hormone-sensitive lipase. After digestion of dietary fat, medium-chain fatty acids (8–12 carbon chain) are transported coupled with albumin, whereas long-chain fatty acids (14 or more carbon chains) are converted back to triglycerides, incorporated into chylomicrons, and transferred to the circulation via the thoracic duct.
There are two sources of FFA for uptake by organs: albumin-bound FFA and conversion of circulating triglycerides into FFA by the endothelium-anchored enzyme LPL (Fig. 3.3). The balance between these two sources of FFA uptake is significantly altered in nephrotic syndrome. For reasons that are unknown and need to be explored in the future, albumin with a low FFA content is preferentially lost in urine by proteinuric kidneys, but albumin with high FFA content is not, so the result is a progressive retention of albumin with high FFA content [29, 21]. As proteinuria reaches nephrotic range, hypoalbuminemia develops, and a combination of high FFA containing albumin and hypoalbuminemia raises the plasma ratio of FFA to albumin [21]. This elevated plasma FFA-to-albumin ratio induces increased FFA uptake in the skeletal muscle, heart, and adipose tissue, which in turn increases Angptl4 expression and secretion from these tissues. Since Angptl4 is a known PPAR target gene [30–32], and PPAR expression is increased during the nephrotic phase in these tissues [21], at least part of this Angptl4 upregulation is likely to be PPAR mediated. Angptl4 secreted from these organs into the circulation has two effects presented in a local and a systemic feedback loops (Fig. 3.4). First, it binds to the αvβ5 integrin in glomerular endothelium and reduces proteinuria (Fig. 3.4, systemic feedback loop). The precise mechanism by which the Angptl4–αvβ5 integrin interaction reduces proteinuria is not known, but it is possible that additional feedback loops within the glomerulus are involved. Second, Angptl4 inactivates LPL activity in these organs, thereby reducing the conversion of triglycerides into FFA, which reduces FFA uptake by this pathway (Fig. 3.4, local feedback loop), and also results in hypertriglyceridemia. Overall, it looks like the local feedback loop, in which Angptl4 decreases LPL activity and then reduces the availability of FFA generated from triglycerides, reduces the effectiveness of the systemic feedback loop by limiting the extent of Angptl4 upregulation [21]. This attempt to reduce proteinuria by Angptl4 represents a systemic response against rising proteinuria.
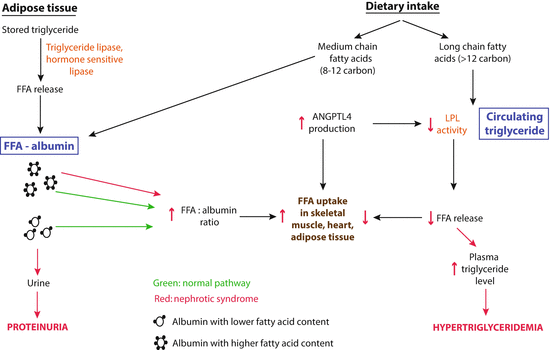
Fig. 3.3
Schematic illustration of the two sources of free fatty acids (FFA) available for uptake by the skeletal muscle, heart, and adipose tissue in the normal and nephrotic state (From [41]). Green shows normal conditions, and red illustrates changes in nephrotic syndrome. The balance shifts significantly to albumin-bound free fatty acids (FFA) because of retention of albumin with high FFA content in nephrotic syndrome. Angptl4 secreted from these organs reduces the conversion of triglycerides to FFA by inactivating lipoprotein lipase (LPL), thereby reducing use of triglycerides and resulting in hypertriglyceridemia
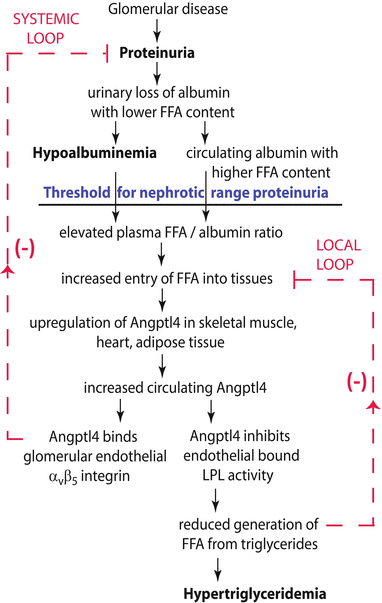
Fig. 3.4
Circulating Angptl4: link between proteinuria and hypertriglyceridemia in nephrotic syndrome (From [21]). Schematic illustration of negative feedback loops in the link between proteinuria, hypoalbuminemia, and hypertriglyceridemia that are mediated by Angptl4 and free fatty acids (FFA) (unesterified fatty acids with a free carboxylate group). Plasma FFAs are non-covalently bound to albumin, and because of the preferential loss of albumin with low FFA content during proteinuria, albumin with higher FFA content is retained in circulation. As glomerular disease progresses and proteinuria increases, hypoalbuminemia develops, and the combination of high albumin-FFA content and lower plasma albumin levels increases the plasma ratio of FFAs to albumin. This increased available FFA enters the skeletal muscle, heart, and adipose tissue to induce upregulation of Angptl4, mediated at least in part by peroxisome proliferator-activated receptor (PPAR) transcription factors. Angptl4 secreted from these organs participates in two feedback loops. In the systemic loop, it binds to glomerular endothelial αvβ5 integrin and reduces proteinuria. In a local loop, it inhibits lipoprotein lipase (LPL) activity in the same organs from which it is secreted to reduce the uptake of FFAs, thereby curtailing the stimulus for its own upregulation
3.1.7 Importance of the Nephrotic-Range Proteinuria Threshold
In patients, manifestation of the other components of nephrotic syndrome starts only after proteinuria crosses the nephrotic-range proteinuria threshold, which is usually defined as about 3.5 g/d in adults, but it is likely to be quite variable between different individuals and within the same individual among different components. Until recently, the molecular basis for this threshold was not known. It is, at present, only possible to explain the nephrotic threshold in the context of hypertriglyceridemia [21]. Hypertriglyceridemia is dependent on the retention of albumin with high FFA content, resulting in a plasma FFA-to-albumin ratio that is high enough to induce upregulation of Angptl4 expression in the skeletal muscle, heart, and adipose tissue. Studies in experimental animals reveal that, during mild proteinuria, plasma FFA-to-albumin ratio, plasma Angptl4 levels, peripheral organ Angptl4, and PPAR mRNA expression are similar to control non-proteinuric animals. During severe proteinuria, all these parameters are significantly elevated, suggesting that the threshold for nephrotic-range proteinuria, in the context of hypertriglyceridemia, correlates with the downstream effects of an increased plasma FFA-to-albumin ratio.
3.1.8 Current and Future Therapies for MCNS
3.1.8.1 Current Therapies
Current therapies for kidney disease related to proteinuric disorders rely on agents used in other fields than nephrology. In one category, glucocorticoids, cyclophosphamide, azathioprine, chlorambucil, mycophenolate mofetil, cyclosporine, tacrolimus, and the anti-CD20 antibody have been used to treat glomerular disease because of their immunosuppressive properties. Over the past decade, it became clear that several of these drugs have direct effects on glomerular cells [33, 4]. In another category, angiotensin-converting enzyme inhibitors, angiotensin receptor blockers, spironolactone, and renin inhibitors such as aliskiren block the renin–angiotensin system and are used for supportive therapy.
Among these drugs, glucocorticoids are usually the first line of therapy for MCNS. Indeed, up to 95 % of patients with MCNS are sensitive to glucocorticoid therapy [1
Buy Membership for Nephrology Category to continue reading. Learn more here