45 Anesthesia Outside the Operating Room
Off-Site Anesthesia: Structure
Quality Assurance of Anesthesia Services and Outcome in the Off-Site Areas
Anesthesia versus Sedation for Non–Operating Room Procedures and Tests in Children
Logistics of Managing Acute Emergencies and Cardiopulmonary Arrest Outside the Operating Room
Difficult Airway Management in the Non–Operating Room (Off-Site) Environment
THE APPROACH TO PROVIDING ANESTHESIA outside the operating room (OR) for children (also known as non–operating room anesthesia [NORA] or off-site anesthesia) varies greatly among organizations and even from one anesthesia provider to another. NORA practice is not as standardized as anesthesia delivered within the OR setting. As such, it is a difficult topic to cover using an evidence-based approach. Although most anesthesiologists would agree (generally) on the optimal methodology for delivering anesthesia to a 2-year-old child for inguinal hernia repair, much more variability (in terms of drugs used, airway management techniques, and general organization) exists in providing anesthesia for a magnetic resonance imaging (MRI) scan. This issue is made even more confusing by the fact that procedures that are performed with sedation in one institution may be accomplished with general anesthesia in another. Furthermore, a procedure that is performed under sedation with an anesthesia provider in one institution may have sedation provided by a specialist other than an anesthesiologist at a different institution.1
The discussion of anesthesia services outside the operating room must also include the recognition that the level of sedation or anesthesia for a given child at any moment during a procedure is often a matter of some conjecture. Almost any procedure that involves pain, or the requirement for absolute movement control in a child, necessitates deep sedation or general anesthesia. The distinction between these two states—defined by the presence or absence of movement in reaction to painful stimuli—is often difficult to determine.2 For anesthesiologists, the difference between these two states is more of semantic interest than practical importance. Anesthesia services are often requested outside the OR when sedation is actually what is required. Finally, anesthesia is often provided outside of the OR for patients with significant comorbidities undergoing routine procedures. Many of these children would be managed with sedation by other specialists were it not for the complexity of the coincident condition(s). Comorbid conditions that require referral to an anesthesiologist vary with institutions but common (generally accepted) examples include:
1. Extremely young age, including well children younger than 1 month of age
2. History of significant prematurity (younger than 32 weeks at birth) and post–conceptional age younger than 60 weeks.
3. History of ongoing apnea and bradycardia episodes
4. Craniofacial anomalies or any known difficult functional or anatomic airway problem
5. Cyanotic congenital heart disease or cardiomyopathy
6. Any serious coexisting disease such as sickle cell disease or muscular dystrophy that would qualify a patient as American Society of Anesthesiologists (ASA) III-IV.
7. Procedures that require elective airway control (intubation) or respiratory control such as breath holding
With these considerations in mind, this chapter focuses on issues specifically related to the delivery of anesthesia and deep sedation outside of the OR provided by anesthesiologists. Issues concerning minimal, moderate, and deep sedation, as well as issues involving care by providers other than anesthesiologists, are covered Chapter 47.
Standards and Guidelines
Anesthesia outside of the OR must meet the same standards as anesthesia given in the OR. Specifically, Medicare’s Conditions for Participation for hospitals are enforced by The Joint Commission (TJC) regardless of location of care. The Conditions for Participation are principles that are articulated to surveyors as instructions in the Interpretive Guidelines published in January, 2011, and available at http://www.cms.gov/SurveyCertificationgeninfo/dounloads/SCLetter11_10.pdf. These guidelines describe appropriate training, credentialing, and oversight of sedation and anesthesia providers and some of the specific requirements for care documentation. The ASA has developed templates for the required policies that help institutions meet the standards of the new Interpretive Guidelines. These resources can be downloaded from the ASA website at http://www.asahq.org. With particular reference to NORA, there are several notable templates, as follows:
1. Preanesthesia Evaluation Policy, Form and Note (template)
2. Intraoperative record policy. Standard data elements and timing must be included in the intraoperative record—just as in the OR.
3. Postanesthesia Evaluation Policy, Note and Form (template)
NORA Services must be organized in such a way as to meet the Conditions for Participation (and thus TJC) standards mentioned earlier, just as they are met in the OR environment. Depending on how NORA is organized in a particular institution, this can be challenging. The departments that require anesthesia services must appreciate the need to meet these standards and allow for the infrastructure to meet or exceed them, particularly in the preanesthesia and postanesthesia timeframes.
Off-Site Anesthesia: Structure
Anesthesia services outside of the OR can be organized in various ways. In some institutions these services are organized through an off-site anesthesia unit or sedation unit. These units have the advantage of providing all anesthesia-related care through one location that contains all of the personnel and equipment required for anesthesia. Ideally, these units provide for preanesthesia assessment, induction, procedure location, and a recovery area. Children may be transported to remote locations when equipment (such as MRI) cannot be brought to the sedation unit. The advantages of this organizational scheme are clear. The uniform environment leads to maximum consistency in the equipment and personnel that interact with children and their families and thus adds to safety, efficiency, parent satisfaction, and effectiveness of care. Many experts (along with TJC) advise the formation of specialty teams or microsystems for provision of anesthesia out of the OR. Microsystems are coordinated groups of professionals who deliver a specific service, to achieve the best outcomes by developing reliable, efficient, and responsive systems that have the capability of meeting the individual needs of one child, continually improving care for the next child, and creating a great place to work for all staff. The sedation microsystem should be made up of pediatric anesthesiologists and nursing, technical, and administrative personnel who are familiar with the service and dedicated to this care.3 The microsystem can provide structure and expertise for this care. As members gain expertise and comfort with the off-site environment, their care is consistent and reproducible, leading to less confusion with other services. Such systems of care lead to improved effectiveness (decreasing failed anesthesia and sedation cases) and improve patient and family and staff satisfaction.4–6
Finally, anesthesia services outside of the OR may be primarily organized at the site of procedural care. Most typically these services are organized in radiology departments or gastrointestinal procedure suits, where a majority of pediatric sedation and anesthesia takes place outside of the OR. For this organizational setup, the procedure unit itself may be outfitted for admission and preanesthesia assessment. An anesthetizing location can be provided and recovery of children can be accomplished in a space contiguous within the immediate location of the procedural equipment. This kind of organization is most common in children’s hospitals, where high volumes of procedures are performed in a given location such as the MRI scanner.7 In locations such as this, where anesthesia services are required on a daily basis for a multitude of children, the investment in the infrastructure for anesthesia support services makes economic sense in addition to optimizing patient care.
Personnel Requirements
1. Anesthesia providers should rotate on this service and have a frequency of experience with the environment and ancillary personnel that allow familiarity among the anesthesiologists, nurse anesthetists, respiratory therapists, registered nurses, patient care technicians, biomedical engineers, and child life specialists. This familiarity should be based on a common understanding of the routines and protocols for standard procedures and a common agreement on the goals of the service.
2. Effective and efficient communication among personnel is critical to optimize outcomes. Logically, help from all members of the care team, including the supervising anesthesiologist, should be available within time frames that would allow optimal outcomes for children with critical events—specifically within 3 to 4 minutes. The use of cell phones, Internet phones, or other devices to optimize communication in NORA locations is often helpful.
3. The ancillary personnel in each location must be familiar with the needs and processes of providing anesthesia to children.
4. Equipment and monitoring standards should mimic that of the OR environment (see later discussion). Anesthesia carts and machine preparation and setup should mirror the OR environment as much as possible to maximize the similarity to the most common workspace. It is critical to have a system that allows appropriate restocking and security of anesthesia carts in all of the off-site locations. All off-site carts should include a full range of drugs, intravenous equipment, fluids, and airway equipment such as tracheal tubes, laryngeal mask airways (LMAs), laryngoscopes, oral and nasal airways, masks, and suction equipment in sizes that would fit all possible pediatric age groups.
5. Scheduling off-site anesthesia resources is complex and personally demanding. Timing for some procedures is inexact. In addition, anesthesia time requirements can vary with the child and the associated pathology. Success is enhanced by focusing the task of scheduling NORA procedures with one individual (or a small group) (similar to scheduling cases for the OR) who intimately understands the process involved in anesthesia. This type of organization allows the NORA service to have one focal point for communication between the individuals who perform procedures and the anesthesia service, thus maximizing communication among services and minimizing incorrect assumptions of staffing or timing for procedures.
Specific Environmental Requirements
Equipment (see earlier discussion) and monitoring standards (see later discussion) must meet those of the main OR environment. Regardless of whether the anesthetizing location is inside or outside the OR, the ASA has established minimum standards for equipment, monitors, and conditions of anesthesia delivery. These guidelines may, of course, be exceeded at any time based on the judgment of the involved anesthesia personnel. The ASA specifically requires that remote locations must have two sources of oxygen (O2) (preferably a central source of piped O2 and a backup E cylinder), suction, an anesthesia machine if administering inhalational anesthetics, a scavenging system for waste anesthetic gases, a self-inflating hand resuscitator bag able to deliver 90% O2 and positive-pressure ventilation, standard of care monitors and equipment,7,8 and sufficient electrical outlets, illumination, and space. The ASA Standards for Basic Anesthetic Monitoring include the following:
Pulse oximetry with audible pulse tone and low-threshold alarm
Adequate illumination and exposure of the patient to assess color
Anesthesia machine with O2 analyzer
Continuous end-tidal carbon dioxide (ETco2) analysis with an audible alarm
Continuous electrocardiogram (ECG)
Arterial blood pressure and heart rate every 5 minutes or more frequently as indicated
Temperature, if there is potential for clinically significant changes in body temperature
Many off-site areas do not have wall suction, especially in the MRI environment. MRI-compatible wall suction is not widely available. An alternative method for providing suction in the MRI suite is to mount a suction canister with 30 feet of suction tubing outside the scanner room.9 The suction tubing can then be threaded through a hole in the console wall to access for use in the MRI unit.
It is in the nature of off-site anesthesia that the physical environment and practice patterns are typically that of another medical specialty. It should also be noted that these other specialties practice under standards developed by their own professional organizations that apply to the procedures within their locations reflecting the different procedure goals. The varying specialties involved include (but are not limited to) gastroenterology, dentistry, cardiology, oncology, intensive care, emergency medicine, and radiology.10 Anesthesia providers who work in these environments are well served by familiarizing themselves with the standards for the given specialty area they are working in, as published on the individual websites for the various professional organizations.
Quality Assurance of Anesthesia Services and Outcome in the Off-Site Areas
This chapter reviews issues related to care delivered by members of anesthesiology departments outside of the OR. In this case the quality improvement (QI) and assurance (QA) activities center in the anesthesiology department. Issues relating to sedation services provided by other services are discussed in Chapter 47. Just as is the case in the OR, it is important to have a consistent tracking tool to follow all clinically important complications. Each department can set its own thresholds for review; however, certain incidents logically require inquiry, as follows11:
Unscheduled admissions to the hospital as a direct result of the sedation (i.e., because of protracted emesis, prolonged sedation, respiratory or cardiac complication)
Failed procedures resulting from inadequate or problematic anesthesia or sedation
Cardiovascular compromise that requires assistance from an outside rescue team
Anesthesia versus Sedation for Non–Operating Room Procedures and Tests in Children
Anesthesiologists can deliver either deep sedation or anesthesia for procedures outside of the OR. The choice of whether to deliver general anesthesia with a secured airway (tracheal tube or LMA) using potent inhalational anesthetics versus deep sedation with facemask O2 and propofol infusion depends on many factors, including the child’s comorbid conditions, the procedure, and the experience and comfort level of the anesthesia provider. Several reviews are available on this topic. For MRI scans, propofol sedation has been suggested as an (overall) safe and effective option in children with airway pathologic conditions or who are premature or very young.12 Similarly, multiple reports have recommended both propofol sedation and general anesthesia with tracheal intubation (GETA) techniques for endoscopies.13,14
No clear evidence exists on which to conclude that one technique is specifically better than another for procedures in which both techniques are effective and safe, such as MRI or endoscopy procedures. Recognizing this fact, it is still important for anesthesiologists to avoid providing GETA in every case (ignoring the possible advantages that deep or moderate sedation could provide in terms of rapidity of emergence and lack of side effects). It is appropriate to carefully evaluate the nature of the sedation/anesthesia provided in the non-OR setting and consider all of the possible implications of a given technique. For instance, how efficient and effective is the care that is provided? How well does the care provided meet the requirements of the procedure in terms of pain and movement control? What are the nature and rapidity of the emergence from sedation or anesthesia and the time that children should remain in the hospital or ambulatory unit between emergence and discharge after a specific procedure with a given technique? Only after careful analysis can guidelines be established for the optimal technique for a given procedure.15
When delivering general anesthesia to children out of the OR, the risk benefit of instrumenting the airway must be carefully evaluated. The LMA is perhaps most useful in the MRI or computed tomography (CT) setting because it maintains spontaneous ventilation, enables the anesthesiologist to monitor ETco2 continuously and provides a clear airway in a child who may otherwise have obstruction with a natural airway. With the LMA in place, the child can be maintained with a relatively low level of anesthesia, allowed to breathe spontaneously, and then rapidly awakened at the conclusion of the scan. After the LMA is placed, anesthesia can be provided with either a continuous infusion of propofol or with a low-dose inhalation agent (sevoflurane 1.5% in 50% N2O/O2). In some circumstances, the LMA may provide a suitable airway in children with bronchopulmonary dysplasia, cystic fibrosis, severe asthma, or active respiratory issues. In children with upper respiratory tract infections, the incidence of mild bronchospasm, laryngospasm, breath holding, and major O2 desaturation (less than 90%) in those whose airway was managed with an LMA was reduced compared with those whose airway was managed with a tracheal tube.16 Similarly, the use of LMAs in ex-preterm infants with bronchopulmonary dysplasia resulted in less coughing and wheezing and greater hemodynamic stability than in those managed with tracheal tubes. In children who underwent a vitrectomy for retinopathy of prematurity, the time to discharge after an LMA was less than that after a tracheal tube.17 LMAs provide more hemodynamic stability during their removal than during tracheal extubation and thus the former may be useful in children with hemodynamic instability.18
Others think any instrumentation of the airway theoretically increases the risk of triggering airway reflexes or regurgitation compared with no airway instrumentation. In healthy children, some clinicians prefer a deep sedation technique, for example, for brain MRI that includes a propofol infusion, an optimally positioned upper airway (with a roll under the cervical spine and the neck extended) and noninvasive monitoring (nasal capnometry supplemented with oximetry, an electrocardiogram, and noninvasive blood pressure).19 Some note that the majority of children do not require an airway during deep sedation for medical procedures. However, in some (e.g., those with excess secretions or upper airway obstruction during the sedation), the following algorithm of airway intervention may be followed to relieve the obstruction: reposition the head and shoulders, insert an oral airway, insert a nasal airway, place an LMA, and finally, if the LMA fails to clear the airway, place a tracheal tube.
Logistics of Managing Acute Emergencies and Cardiopulmonary Arrest Outside the Operating Room
Although the actual management of a cardiopulmonary arrest should not vary between the OR setting and the non-OR setting, the logistics of performing a resuscitation may be challenged by unanticipated factors, such as personnel who may not be familiar with code situations, an environment that makes performing a resuscitation difficult, or equipment that may be unsafe if used in the particular location (e.g., the MRI environment). It is important that all personnel in the off-site location be familiar with the location and operation of the code cart. The anesthesia cart and the code cart in the off-site location should be stocked in the same precise configuration as all others throughout the hospital and ORs. Standardizing the code carts throughout the hospital ensures that all ancillary personnel can be helpful in locating critical items. If the code cart is kept locked, the key or access code must be readily accessible and in a location that is known to all essential personnel. A hard board on which chest compressions may be performed should also be readily available. Each off-site location should have an identified and rehearsed routine for announcing a code situation and summoning aid. The use of human patient simulation can be very helpful in testing the team response to critical events. Simulators can be used in place in off-site locations to replicate critical events and evaluate the ability of the care team and backup systems to resuscitate a patient. Blike and colleagues used exactly this methodology to document significant variation in the ability of rescuers to resuscitate children from sedation or anesthesia critical events in locations outside the OR.20
Of all the non-OR environments in which anesthesiologists are asked to provide care, the MRI scanner poses a unique challenge for cardiopulmonary resuscitation. The MRI environment is divided up into four zones that correlate with the intensity of the magnetic field and the risk to children and health care providers. These zones are delineated in Table 45-1.
TABLE 45-1 Descriptions of the American College of Radiology’s Four Zones in the Magnetic Resonance Imaging Suite
ACR Zones | Occupants | Hazards |
---|---|---|
Zone I | General public | Negligible |
Zone II | Unscreened MRI patients | Immediately outside area of hazard |
Zone III | Screened MRI patients and personnel | Potential biostimulation interference, access to magnet room |
Zone IV | Screened MRI patients under constant direct supervision of trained MRI personnel | Biostimulation interference, radiofrequency heating, missile effect, cryogens |
ACR, American College of Radiology; MRI, magnetic resonance imaging.
From the Joint Commission International Center for Patient Safety. Available at: http://www.acr.org/~/media/ACR/Documents/PDF/QualitySafety/MR%20Safety/SafeMR07.pdf.
In 2009 the ASA published a report titled Practice Advisory on Anesthetic Care for Magnetic Resonance Imaging: A Report by the American Society of Anesthesiologists Task Force on Anesthetic Care for Magnetic Resonance Imaging.21 This document advises that in the case of a medical emergency in a patient within the scanner, the anesthesia providers should (1) initiate cardiopulmonary resuscitation while immediately removing the patient from zone IV, (2) call for help, and (3) transport the patient to a previously designated safe location in proximity to the MRI suite. This designated location should contain a defibrillator, vital signs monitors, and a code cart with all resuscitation drugs, airway equipment, O2, and suction. Other acute emergencies that are unique to the MRI environment include a “quench” or a fire in the scanner. Quenching occurs when the liquid that cools the magnet boils off rapidly and results in helium escaping from the cryogen bath. The magnetic field of the magnet is rapidly decreased because the coils in the magnet cease to be superconducting and become resistive. In addition to performing the institution’s protocol in reaction to either of these events, the ASA consultants involved in writing the advisory agree that in the event of a quench, (1) the child should be removed from zone IV immediately and (2) O2 should be administered immediately; (3) because of the powerful magnetic field that can exist after a quench or a fire, emergency response personnel should be restricted from entering zone IV.
Difficult Airway Management in the Non–Operating Room (Off-Site) Environment
The more difficult scenario is that of the unrecognized difficult airway22; this scenario may best be handled by establishing a local management protocol that can be activated when the situation arises. Each institution has peculiar equipment, space, and personnel resources. NORA leaders should establish a local protocol for management of the unanticipated difficult airway in an off-site location. In some cases this might involve bringing advanced airway management equipment to the location in a rapid, organized manner. In other cases the best option would include “temporizing” management with alternative airway devices and transporting the child to a location where a definitive airway can be placed in a controlled manner. For this reason, it is important to have alternative airway devices such as LMAs stocked in all anesthesia carts that are designated for off-site locations. In the event that the lungs cannot be ventilated or the trachea intubated, the LMA can provide a lifesaving temporary airway until more definitive action can be taken.23,24
Specific Locations for Non–Operating Room Anesthesia
Computed Tomography
CT involves ionizing radiation and can provide a good modality for differentiating between high-density (calcium, iron, bone, contrast-enhanced vascular and cerebrospinal fluid [CSF] spaces) and low-density (O2, nitrogen, carbon in air, fat, CSF, muscle, white matter, gray matter, and water-containing lesions) structures. Because the scan time for current devices is brief, with actual imaging time ranging from 5 to 50 seconds per sequence, many children are able to tolerate CT without sedation or anesthesia. In cases in which anesthesia is required, it is often for those who have a fragile or unstable respiratory or cardiovascular status. Anesthesia or sedation is often required for children who are unable to cooperate (cognitively impaired children and those younger than 2 to 3 years of age) or require CT emergently. Emergent indications for CT include head trauma, unstable respiratory status in need of a pulmonary diagnosis, unexplained changes in mental status, or neoplasm workup in severely debilitated children. Anesthesia management is also necessary with a potentially unstable airway (peritonsillar abscess, anterior mediastinal mass, craniofacial anomaly, tracheoesophageal fistula, uncontrolled vomiting, or gastroesophageal reflux), or the need for breath holding during acquisition of images (three-dimensional dynamic airway studies) (Figs. 45-1 and 45-2). Some CT units are particularly concerned about children moving when contrast is injected (because of dose restrictions the contrast injection cannot be repeated) and therefore request anesthesia services for any child they cannot confidently predict will stay motionless for the study.
Children with Down syndrome present a particular area of interest for the anesthesiologist working with the CT scanner. These children pose a unique risk for atlantoaxial instability and may require a head or neck CT to evaluate cervical and temporomandibular anatomy, recurrent sinusitis, or choanal atresia. The incidence of atlantoaxial instability varies from 12% to 32%.25 Many children with Down syndrome require cervical spine radiographs before entering grade school or participating in the Special Olympics. Usually, the parents know the outcome of these tests and can relay the results of cervical spine radiographs. These studies alone do not indicate to the practitioner whether the child is at risk of dislocation.23 Rather, it is the presence of neurologic signs or symptoms that would herald a spine that is “at risk”: abnormal wide-based gait, incontinence, increased clumsiness, fatigue with ambulation, complaints of numbness, tingling in an extremity, weakness of an extremity, or a new preference for sitting games. In infants, these clinical signs may be difficult to assess. In younger children, developmental milestones (e.g., crawling, sitting up, reaching for objects) should be evaluated. Physical signs may include clonus, hyperreflexia, quadriparesis, neurogenic bladder, hemiparesis, ataxia, and sensory loss. Children with atlantoaxial instability on a radiograph are at less risk for dislocation if they do not exhibit any signs or symptoms of instability. Children who are capable of following commands are asked to perform full neck flexion and extension maneuvers to determine whether pain, sensory, or motor manifestations of cord compression develop.
Perhaps the most controversial issue facing anesthesiologists with regard to CT scans is the issue of oral contrast for CT. Because children lack abundant retroperitoneal fat, they do not have the natural contrast needed to elucidate abdominal images. For this reason, children may be required to ingest (orally or via nasogastric tube) diatrizoic acid (Gastrografin) to opacify the stomach and bowel. Oral contrast is useful in the identification of an intraabdominal abscess, mass, fluid collection, bowel injury, pancreatic injury, or other traumatic injury. The oral contrast comes as Gastrografin 3% and may be diluted to a 1.5% to 2.5% strength concentration. Gastrografin 3% is the full-strength concentration; in this undiluted form, Gastrografin is hypertonic (2200 mOsm/L). At this concentration, it can cause pulmonary edema, pneumonitis, osmotic effusions, and death if aspirated. Fortunately, when Gastrografin is administered to children for CT it is diluted to 1.5% to 2.5% strength, which is thought to be much less dangerous if aspirated. The volume of oral contrast that is administered can be quite large. Neonates typically receive 60 to 90 mL. Infants between 1 month and 1 year of age may receive up to 240 mL. Children between the ages of 1 and 5 years receive between 240 to 360 mL of contrast medium. Risk is introduced when these children require anesthesia within an optimal window after ingestion (usually 30 minutes to 1 hour after receiving the contrast agent) to enhance visualization. By most fasting guidelines (nil per os [NPO]), Gastrografin consumption within 1 to 2 hours of an anesthetic or sedation does not fall within the usual NPO guidelines. Yet, the scan must be completed while the Gastrografin is present in the gastrointestinal tract. Despite the large volume of Gastrografin that may be ingested, there does not appear to be a significant aspiration risk in this population, and many anesthesiologists do not secure the airway with a tracheal tube (Fig. 45-3). A review of the pediatric and adult literature confirms that over the last 35 years only a few case reports have been published of aspiration syndrome attributed to Gastrografin, all in extremely high-risk patients.24–26 Several investigators have evaluated this issue from different perspectives. In one study, a cohort of 50 patients who received oral contrast after blunt abdominal trauma were evaluated for radiologic evidence of aspiration pneumonia or clinical complications of aspiration.27 Some of the patients received general anesthesia, and some were neurologically impaired (including several with increased intracranial pressure). In this very high-risk group, only one patient had a question of aspiration on a chest radiograph after the CT scan, and that patient did not develop pulmonary symptoms. Another study evaluated the volume of gastric contents of 365 patients undergoing deep sedation or general anesthesia for abdominal CT scans.25 Gastric contents exceeded 0.4 mL/kg in 49% of those who received gastric contrast. Two cases of vomiting were recorded. None of the patients in the study developed clinical evidence of aspiration. When dilute Gastrografin is used, the risks associated with pulmonary aspiration appear to be small, even in children who are moderately to deeply sedated.28 In spite of this evidence, no large cohort studies have been conducted that have the power to determine the actual incidence of aspiration in this setting. Therefore no accepted standard of care for the airway management of these children has been determined. Some anesthesiologists induce anesthesia with an intravenous or inhalational technique and maintain the anesthetic without securing the airway with a tracheal tube. Others perform rapid-sequence induction and tracheal intubation. The lack of consensus among anesthesiologists and the absence of evidence preclude a clear and consistent recommendation for managing the airway in these children.
Nuclear Medicine
Nuclear medicine is one of the oldest functional imaging disciplines. Because of the advances in instrumentation and radiopharmaceuticals, nuclear scanning is becoming an increasingly popular imaging modality. Nuclear imaging can be used to evaluate an incredible range of pathologic conditions. These scans are useful for identification of the extent of disease for a large number of neoplasms.29 They can also be used to detect epileptic foci in refractory epilepsy, evaluate cerebrovascular disease (e.g., moyamoya disease) and cognitive and behavior disorders, and detect and delineate renal function and disease, including detection of reflux and acute pyelonephritis.23 Improvements in the hardware for nuclear imaging has greatly decreased the scan time, although even with the advent of two-level emission and transmission scans and combined CT imaging, a child must remain motionless for 20 to 60 minutes. The nuclear medicine environment is quiet, and the scan is pain-free. Accordingly, the indications for anesthesia or sedation include only those children who could not be reasonably expected to hold still for the 20 to 60 minutes, such as those with cognitive or behavioral impairment, young age, or severe pain or who are claustrophobic.29 Sedation providers should be aware that the equipment in nuclear medicine imaging emits no ionizing radiation, but rather the radiation is contained within the child and is of very low energy levels. Depending on the nature of the study, the children require intravenous access for administration of the nuclear tracer well in advance of the scan and therefore usually have intravenous access for the anesthesia or sedation. Many nuclear scans also require an empty bladder to avoid interference from concentrated tracer in an enlarged bladder. Accordingly, the bladder is often catheterized after anesthesia is induced and the radioactive urine that is collected is disposed of in a radioactive-safe manner.
Two relatively new nuclear scans that involve anesthesia and present particular challenges are single-photon emission computed tomography (SPECT) and positron emission tomography (PET) scans. SPECT scans use single-photon gamma-emitting radioisotopes and rotating gamma cameras to produce three-dimensional brain images. SPECT scans involve the use of radiolabeled technetium-99m (half-life of 6 hours), which has a high rate of first-pass extraction and intracellular trapping in proportion to regional cerebral blood flow. This scan is useful for localizing seizure foci. It appears to be as accurate as invasive direct cortical mapping in this regard.30 Injection of the radionuclide during a seizure will tag areas of increased cerebral blood flow and localize the seizure foci. The child should be scanned within 1 to 6 hours of the seizure and injection of the tracer. Logistically this can pose a problem because there is no way to predict exactly when a seizure will occur. Therefore the neurology service needs to communicate to the anesthesiology service the importance of being flexible in providing anesthetic services within the window of time allowed to complete the test whenever the next seizure occurs.
PET scans use radionuclide tracers of metabolic activity such as O2 usage and glucose metabolism. Radionuclide tracers of glucose may be useful when seeking seizure foci or tumor recurrence.29,31,32 Unlike SPECT scans, PET scans should be performed during the seizure itself. Because of the short half-life of the glucose tracer (110 minutes), the scan is best completed during the seizure or within 1 hour thereafter. The logistical problem in providing this service is even more difficult than that of SPECT scans. In addition to the need to have a team available to provide anesthesia on very short notice, there is a risk of hypovolemia, especially in infants, who remain on NPO status and without intravenous hydration while anticipating a seizure. It is therefore important to work with the neurology and neurosurgery services to plan these scans in advance, to ensure intravenous access to avoid hypovolemia, and to arrange anesthesia coverage when needed.
Stereotactic Radiosurgery
Stereotactic radiosurgery (gamma knife) is a major advance in the treatment of selected malignant tumors (ependymoma, glioblastomas), vascular malformations, acoustic neuromas, and pituitary adenomas in children.33,34 Radiosurgery is indicated, especially for those children with a tumor located deep in the brain or in an area that could put the child at significant surgical risk (e.g., speech, motor, cerebellum, brainstem areas) or for the recurrent brain tumor that has failed prior treatment. Radiosurgery involves the use of a single large fraction of radiation that is directed at a specific target with minimal radiation exposure to the surrounding normal tissues. Optimal results are achieved with small tumor volumes (14 cm3 or less).35
After radiosurgery is completed, the child is returned to postanesthesia care unit, where the trachea is extubated under controlled conditions. Risks are inherent with this prolonged anesthesia, which requires multiple transports between sites. One study examined 68 radiosurgery procedures in 65 children and reported four potentially serious anesthesia-related events.36 In those children who received general anesthesia, serious complications included obstruction of the tracheal tube while in the head frame and lobar collapse requiring prolonged mechanical ventilation.
Radiation Therapy
Radiation therapy for children uses ionizing photons to destroy lymphomas, acute leukemias, Wilms tumor, retinoblastomas, and tumors of the central nervous system. Improved three-dimensional imaging and enhanced computing power have allowed radiation oncologists to conform radiation dose to the shape of the tumor and minimize radiation to the surrounding tissues.37 The energy absorbed by the tissues is measured in grays (Gy), which has replaced the term rad. One Gy is equivalent to 100 rad. Although most children receive standard x-ray therapy, specific lesions may respond better to bombardment with electron, proton, or neutron beam therapy. The anesthetic considerations are identical regardless of the type of therapy in this respect.38 A planning session in a simulator is typically scheduled before the initiation of radiation therapy to map the fields that require irradiation while the child is in a fixed position. For proton beam treatments, a planning session is carried out in a CT scanner. A fiberglass immobilization cast of the head is made while the child is anesthetized with propofol and with a natural airway (Fig. 45-4). This cast allows secure positioning to ensure that the child does not move during treatment, but considerable care is required to configure the mask for optimal airway patency while ensuring proper windows for treatments because the mask may interfere with airway access.
Radiation therapy involves “fractionated” exposure. Repeat sessions are typical. The child must be motionlessness during therapy to precisely target malignant cells. Radiation therapy is typically administered by dividing the total radiation therapy course among daily or twice-daily sessions. In general, most treatment sessions last between 15 and 30 minutes and the planning simulation session may take as long as 2 hours, depending on the nature and location of the target lesion or area for therapy. For children who require neuraxial treatments for spinal metastases, as many as four fields may be irradiated and in both the supine and prone position. Dividing the total radiation therapy course into discrete daily sessions allows normal tissue repair between sessions while the tumor burden is lessened or destroyed. These children are optimally managed via central venous access that obviates the need for repeated venipunctures. Unless there is a specific airway anomaly, daily anesthetic management typically consists of general anesthesia using a propofol infusion (with or without midazolam pretreatment),39 blow-by oxygen via nasal cannula or face mask, and spontaneous ventilation monitored with ETco2. Tachyphylaxis does not appear to be an issue even with multiple, frequent, treatments.40 One retrospective review of 177 patients undergoing 3833 radiation treatments documented a 1.3% complication rate.41 This rate compares favorably with the rate of complications for children undergoing propofol-based anesthesia without cancer.42
Dexmedetomidine sedation has been used for radiation therapy, but its use has not become widespread, most likely because of the need for a 10-minute loading dose and relatively frequent need for repeated boluses at the doses that have so far been studied.43 Single-dose IV ketamine 0.5 to 0.8 mg/kg provides effective sedation, albeit with a greater half-life and more adverse effects (e.g., vomiting) than propofol.44
One exception to the technique of moderate or deep sedation for radiation therapy is the treatment of retinoblastoma. In this case, the eye must be completely immobile during the treatment. General anesthesia or very deep propofol sedation is indicated in these cases to ensure appropriate irradiating conditions; ketamine, with its side effect of lateral nystagmus, is not appropriate in these cases.44
Magnetic Resonance Imaging
MRI, magnetic resonance spectroscopy (MRS), magnetic resonance angiography (MRA), and magnetic resonance venography (MRV) are employed for the evaluation of neoplasms, nonhemorrhagic trauma, vascular lesions, cardiac lesions, orthopedic lesions (including joint disorders, osteomyelitis), central nervous system and spinal cord lesions, craniofacial disorders, detecting the origin of developmental delay, behavioral disorders, seizures, failure to thrive, apnea, cyanosis, hypotonia, and in the workup of mitochondrial and metabolic diseases.45–48 MRA and MRV are especially helpful in evaluating vascular flow and often can replace invasive angiography in follow-up evaluations of vascular malformations, interventional therapy, or radiotherapy.49,50 All of these imaging modalities are essentially equivalent in terms of the requirements for the provision of anesthesia or sedation and thus can be considered the same as for MRI in the following discussion.
A variety of safety issues with respect to MRI are important, as mentioned in the earlier discussion of management of acute emergencies during anesthesia outside the OR. A useful review of these issues has been published by the American College of Radiology entitled “ACR Guidance Document for Safe MR Practices: 2007.”51 All individuals who work in the MR environment should be familiar with the primary recommendations in this paper.
First and foremost is the risk of injury from ferromagnetic objects that can become attracted to the magnetic core of the MRI scanner and cause significant morbidity and mortality (Fig. 45-5). In the presence of an external magnetic field, a ferromagnetic object can develop its own intrinsic magnetic field. The attractive forces created between the intrinsic and extrinsic magnetic fields can propel the ferromagnetic object toward the MRI scanner. Numerous injuries have been reported from this mechanism. Objects that have been reported as accidentally attracted to the MRI magnet include a metal fan, pulse oximeter, shrapnel, wheelchair, cigarette lighter, stethoscope, pager, hearing aid, vacuum cleaner, calculator, hair pin, O2 tank, prosthetic limb, pencil, insulin infusion pump, keys, watches, clipboards, and steel-toe or heeled shoes.52,53 Mortality can result from projectile disasters, as in the case of a death in 2001 in which a ferrous O2 tank was inadvertently brought into the MRI scanner after the wall O2 source failed. The O2 tank was “pulled” from the hands of the respiratory therapist and crushed the skull of the child being scanned (New York Times, July 31, 2001:B1, B5). It is absolutely essential that all portable O2 tanks are MRI compatible and that anesthesia carts are not brought into the MRI. Many MRI scanner units now have screening protocols that include a small handheld magnet to test whether objects are ferromagnetic and thus at risk of being pulled into the magnet.
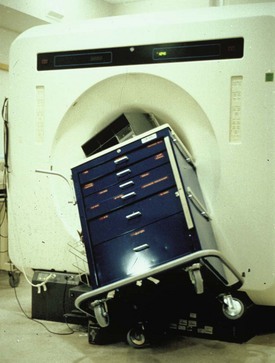
FIGURE 45-5 Magnetic resonance imaging cart that is not compatible inadvertently brought into scanner room.
The other major potential morbidity related to MRI scanning results from implanted devices (i.e., cardiac pacemakers, spinal cord stimulators, programmable ventriculoperitoneal shunts) that may malfunction in the powerful magnetic field of the MRI scanner and can cause injury. These injuries are most often caused by inappropriate patient screening or unfamiliarity with a particular implant’s MRI compatibility. Recently the U.S. Food and Drug Administration (FDA) changed the terminology for designating MRI safe objects from MRI-compatible and MRI-incompatible to MRI-safe, MRI-unsafe, and MRI-conditional (www.fda.gov/MedicalDevices/DeviceRegulationandGuidance/GuidanceDocuments/ucm107705.htm). This terminology will not be applied retrospectively to objects previously designated as MRI-non-compatible. MRI-safe is defined as not posing any known hazard in any MR environment. MRI-conditional refers to objects that may or may not be safe, depending on the specific conditions that are present. Examples include a laryngoscope, cardiac occluder device (e.g., for closure of an atrial septal defect), halo vest, or cervical fixation device. MRI-unsafe means the object should never be brought into the MRI environment because it poses a potential or realistic risk or hazard.
All implanted objects should be carefully evaluated before a patient or health care provider enters the MRI suite. The website http://www.MRIsafety.com is a useful resource for identifying MRI-safe objects. Note that stainless steel or surgical stainless objects may interact with the external magnetic field, potentially resulting in translational (attractive) and rotational (torque) forces. Special attention should be paid to intracranial aneurysm clips (may move and potentially dislodge), vascular stents, cochlear and stapedial implants, shrapnel, intraorbital metallic bodies, and prosthetic limbs. In fact, some eye make-up and tattoos may contain metallic dyes and are at risk of causing skin, ocular, periorbital, and cutaneous irritation and burning.54–56 Bivona (Smiths Medical, Dublin, Ohio) tracheostomy tubes pose a risk in the MRI environment because of the ferrous material within the tracheostomy tube. Bivona tracheostomy tubes are not FDA approved as MRI-compatible or MRI-safe. These tubes may produce rotational and translational motion on the tracheostomy tube itself, may create artifact on MRI images of the head or neck, and may create a thermal hazard from the heating of the ferrous elements in the magnetic environment. All Bivona tracheostomy tubes should be replaced with an MRI-safe tracheostomy tube or a tracheal tube until the FDA confirms otherwise.57–58
Cardiac pacemaker compatibility is a matter of some debate.59 Historically, the presence of a pacemaker has been considered a contraindication for the MRI environment. Most pacemakers have a reed relay switch that can be activated when exposed to a strong magnetic field and convert the pacemaker to the asynchronous mode. In at least two known cases, patients with pacemakers died from cardiac arrest while in the MRI scanner.57 Adverse events associated with pacemakers in the MRI scanner include ventricular fibrillation, rapid atrial pacing, asynchronous pacing, inhibition of pacing output, and movement of the device.58,60
Since 1996, however, changes in pacemaker electronics have included decreased ferromagnetic content and increased sophistication of the computer capabilities. In 2004, one study reported no changes in pacemaker capabilities or adverse outcomes after 56 patients with pacemakers underwent 62 MRI procedures.61 Similar results were reported in 68 patients whose pacemakers were reprogrammed to asynchronous or demand mode before undergoing scans in a 1.5-T MRI scanner. Unfortunately, there is a paucity of data on children with pacemakers or implanted defibrillators who underwent MRI scans, particularly with respect to scanners with 3-T field strength. Extreme caution and consultation with local experts is advisable before administering sedation or anesthesia in children with pacemakers in these environments.
Auditory considerations exist with respect to MRI. Specifically, a loud banging sound and vibrations are produced as the forces generated within the gradient coils of the MRI scanner cause the gradient coils to vibrate. The noises generated range from 65 to 95 dB in a 1.5-T magnet. Cases have been reported of temporary hearing loss after an MRI scan.62 This report suggests that earplugs may prevent the temporary hearing loss associated with MRI. Given these data, earplugs or MRI-compatible headphones are routinely used in children undergoing MRI scans.
Temperature regulation for children during sedation or analgesia for MRI has been the source of significant discussion and speculation. Cool temperatures and humidity are required for proper magnet function. These conditions are a setup for radiant and convective heat loss. Anesthesia limits intrinsic thermoregulation. On the other hand, the MRI generates radiofrequency radiation (RFR) that is absorbed by the child and may offset heat lost to the environment. The specific absorption rate (SAR) is measured in watts per kilogram and is used to follow the effects of RF heating. The FDA allows a SAR of 0.4 watts/kg averaged over the whole body.63 Data suggest that children can increase their core body temperature by 0.5° C during MRI of less than 1 hour duration in a 1.5-T environment.64,65 The temperature of infants who underwent MRI scans of the brain increased 0.2° C with a 1.5-T scanner and 0.5° C with a 3-T scanner, with minimal efforts to prevent passive heat loss.66 Accordingly, it seems appropriate to minimize efforts to avoid passive heat loss in the MRI scanner and to monitor temperature in the 3-T environment particularly for long scans.
Focal heating remains a concern with respect to monitoring equipment in the MRI scanner. For example, the ECG leads should not have frays or exposed wires; any coils or loops in a conductor such as ECG wires or a pulse oximeter probe can cause tissue burns (Fig. 45-6). Cases of first-, second-, and third-degree burns after MRI have been reported.67 To avoid patient injury, the following precautions should be taken: (1) Avoid creating a conductive loop between the child and a conductor (ECG monitoring or gating leads, plethysmographic gating wire, and fingertip attachment), (2) do not leave any unconnected imaging coils in the magnet during imaging, and (3) prevent all exposed wires or conductors from touching the child’s skin during the scan.
Many MRI scans require contrast administration. Gadolinium (gadopentetate dimeglumine) is an FDA-approved contrast agent that is intravascularly administered for MRI enhancement. Approved for use in 1998, gadolinium provides greater contrast between normal and abnormal tissue throughout the body. Gadolinium forms a complex with chelating agents that facilitates biodistribution to the extracellular compartment and excretion via the kidneys. Unlike iodinated contrast agents, gadolinium complexes do not present a significant osmotic load. Gadolinium agents are considered to be safer than iodine agents with respect to adverse reactions. It is reportedly easily removed with dialysis.68 On the other hand, recent warnings from the FDA suggest that gadolinium-containing contrast agents may be associated with the development of nephrogenic systemic fibrosis (NSF) or nephrogenic fibrosing dermopathy (NFD) in patients with moderate to end-stage kidney disease. The FDA suggests that gadolinium be administered only if necessary in children with advanced kidney failure and that, in those children, prompt dialysis be considered after gadolinium administration for MRA studies (http://www.fda.gov/Drugs/DrugSafety/DrugSafetyNewsletter/ucm142889.htm). The incidence of severe anaphylactic reactions to gadolinium is less than that of iodine-based contrast media. The incidence of severe anaphylactic or anaphylactoid reactions is 0.01% to 0.0003%.69 Care must be taken to ensure the proper dose by weight and creatinine if renal function is compromised. Dosing estimates may be obtained from the following website based on the glomerular filtration rate: https://www.radiologyprotocols.com (free trials available for 1 month).
MRI-compatible equipment continues to be developed and improved. Multiple MRI-compatible anesthesia machines (with ventilators), monitors (including wireless models), and infusion pumps are now available. Unfortunately the cost for this equipment is substantial. An MRI-compatible anesthesia machine costs approximately $60,000, MRI-compatible monitors range up to $140,000, and compatible infusion pumps are $12,000 to $18,000. This cost may be overwhelming, especially in facilities with limited financial resources or limited need for MRI anesthetics. However, it is highly advisable for institutions to make the investment. If patient volume is not high enough or financial backing to support such an investment is lacking, then special planning may be instituted to deliver anesthesia without a full complement of MRI-compatible equipment. Specifically, a non–MRI-compatible anesthesia machine could be used in the following manner: the machine must be positioned outside the MRI suite, and 30 feet of parallel ventilator circuit (Bain Mapleson D) or circle system extension may be threaded through the appropriate-sized hole in the console wall to the child within. Alternatively, intravenous sedation or anesthesia can be delivered using MRI-compatible infusion pumps and O2 can be delivered using a facemask or nasal cannula. If an MRI-compatible ETco2 monitor is not available, a conventional carbon dioxide (CO2) monitor may be situated outside the MRI room and the 30-foot-long tubing threaded through the console wall in a similar fashion to attach to the child within.66 If GETA is required without an MRI-compatible anesthesia machine, it is safer to anesthetize the child outside the scanner (anesthesia circuit threaded through the console wall and then back out the entrance door to an induction area), secure the airway, and then move the child into the scanner. Similarly, if propofol sedation is intended, the propofol infusion pump can also be situated outside the scanner and equipped with 30 feet of intravenous infusion tubing. It is important to determine whether the pump is able to infuse accurately through the resistance of the long tubing and that the caliber of the tubing is sufficiently large that the length of tubing required does not trigger the pump’s high-pressure alarm. An Ambu bag or Mapleson circuit must always be situated in the MRI suite and connected directly to an O2 source within the scanner. This is critical, especially when the anesthesia machine is far from the child.
Anesthetic management of children in the MRI suite depends to a large extent on the availability of support personnel and equipment, the anesthesiologist’s personal anesthetic practice, and the child’s medical history. In clinical practice, the choice of anesthetic varies greatly among anesthesiologists. Airway management may include an LMA, a tracheal tube, or a natural airway (with a roll placed under the shoulders). With an LMA or tracheal tube, either inhalational or intravenous anesthesia may be used to establish a motionless child. With a natural airway, only intravenous sedation or anesthesia with propofol or dexmedetomidine is commonly used.7,70–75 ETco2 should be monitored; in the case of nasal prongs, a septate design that delivers O2 (2 to 4 L/min) through one nostril while aspirating gas for CO2 (capnometry) through the other allows for continuous assessment of respirations during spontaneous ventilation. If respiratory problems occur, immediate access to the airway is not possible while the child is in the bore of the scanner; for this reason, some anesthesiologists may prefer to insert an LMA or tracheal tube in all children. Most LMAs are MRI-compatible, although the pilot balloon should be taped to the circuit tubing when imaging the head or neck because it may create imaging artifacts (Figs. 45-7 and 45-8).
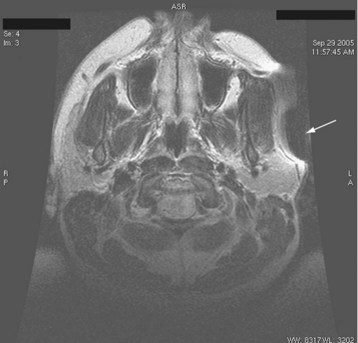
FIGURE 45-8 MRI scan of the patient in Figure 45-7 demonstrating artifact (arrow) created by ferrous material in pilot balloon.
Few studies have directly compared general anesthesia with a controlled airway to intravenous sedation or anesthesia techniques during MRI. One randomized study of 200 children demonstrated no difference in airway complications between the two groups. However, more pauses occurred during scans (for movement, etc.) with propofol sedation, but much less agitation was experienced on emergence after the scan.76 To ensure MRI scans without interruption in the majority of children, many begin with infusion rates of propofol 200 to 250 µg/kg/min in children and either maintain that infusion rate throughout or taper the infusion rate as described previously.77 For younger children (infants) and children with severe cognitive dysfunction, infusion rates 20% to 50% greater than 250 mg/kg/min may be required to prevent movement during MRI scans. Other studies documented agitation78 and prolonged nausea and vomiting79 after MRI scans performed under general anesthesia with inhalation agents. Some MRI scans (cardiac, thoracic, or abdominal) require breath holding to obtain adequate images. In such cases, it is necessary to control the airway with an LMA or endotracheal tube and deliver general anesthesia. After reviewing the literature, insufficient evidence exists to recommend a particular anesthetic technique for MRI scans. Anesthesiologists still must consider their own practice environment and expertise, the demands of the scan itself, and the comorbidities of the particular child when selecting an anesthetic technique, particularly for children undergoing a cardiac MRI.
Interventional Radiology and Invasive Angiography
Interventions involving the abdomen and pelvis have unique considerations; N2O can diffuse into the bowel, causing distention and, in some cases, distort or mask the vasculature of interest; it should be used with caution. In addition, when angiographic imaging of the abdomen is required, the interventional radiologist may request that the anesthesiologist administer intravenous glucagon, usually in 0.25-mg increments. Glucagon reduces peristalsis and, as a consequence, reduces motion artifact during image acquisition.80 Glucagon has been reported to have side effects, including nausea, vomiting, hyperglycemia, depression of clotting factors, and electrolyte disturbances70; close monitoring is warranted, particularly in neonates and small infants.
Cerebral angiograms and interventions can be complex and carry significant risk. Cerebral studies may be indicated in the workup or postoperative follow-up of vascular malformation or tumor resections, stroke, hemorrhagic events, vascular disease, and unexplained mental status changes. These interventions typically require GETA to provide hypercarbia and breath holding. Hypercarbia to ETco2 values of 50 mm Hg or greater promote vasodilation to allow better access and visualization of cerebral vasculature (Fig. 45-9). During these cases, orogastric and nasogastric tubes, esophageal stethoscopes, and esophageal temperature probes should be used with caution because they may cause artifacts on the angiographic images.
Any child who requires a study for the potential or confirmed diagnosis of moyamoya disease should be treated with utmost precaution. These children should have an anesthetic that minimizes the risk of transient ischemic attacks and stroke during the procedure.71 Anesthetic care should begin with the preinduction administration of 10 mL/kg of intravenous fluid to minimize the risk of hypotension (and potential cerebral ischemia) on induction of anesthesia. Hypocarbia should be avoided throughout and mild hypercarbia promoted. In the event of vasospasm or difficult access of small, tortuous vessels, locally administered (through the catheter) nitroglycerin in small doses (25 to 50 µg) may facilitate visualization and access. Although often effective for discretely vasodilating specific areas, nitroglycerin will generally not have a clinically important systemic effect on blood pressure. Consequently, intraarterial blood pressure monitoring usually is not required. Specific protocols have been suggested for minimizing perioperative strokes in these children.72 Sedating the child before starting the intravenous line decreases crying and hyperventilation that can lead to cerebral ischemia. Close attention to postoperative pain control can similarly improve outcomes (see Chapter 24).
The anesthetic management of children with vascular malformations who present for embolization or sclerotherapy can be challenging. These lesions, although present at birth, are often discrete and not clearly visible. As the child grows, the vascular malformation may expand rapidly, growing with the child. In one review, only 18% of lesions presented before 15 years of age.73 This rapid proliferative phase may occur in response to hormonal changes (pregnancy, puberty), trauma, or other stimuli. Vascular malformations may be classified as high-flow or low-flow lesions, depending on which vessels are involved. High-flow lesions may include arteriovenous fistulas, some large hemangiomas, and arteriovenous malformations. Symptoms of high-output cardiac failure predominate in the neonate, and symptoms of stroke, seizures, or hydrocephalus are more common in infants and older children.73 The potential for pulmonary edema should be anticipated and assessed in the physical examination and past medical history (Fig. 45-10). Low-flow lesions consist of venous, intramuscular venous, and lymphatic malformations. Surgical resection of symptomatic vascular malformations may be hazardous, as well as unsuccessful—any vascular element that is not resected may enlarge and cause further problems. It is for this reason that invasive angiography and embolization is a popular alternative to surgical resection.
When embolizing vascular malformations, radiologists often strive to cut off the feeding vessels with agents such as ethanol, stainless steel coils, absorbable gelatin pledgets and powder, polyvinyl alcohol foam, glues, thread, and ethanol. The choice of agent depends on the clinical situation and the size of the blood vessel. Absolute ethanol (99.9% alcohol) is a powerful sclerosing agent that thromboses vessels even at the level of the capillary bed. It is particularly useful in the embolization of symptomatic vascular malformations. Ethanol causes thrombosis by injuring the vascular endothelium. Ethanol also denatures blood proteins. When permanent occlusion is the goal, polyvinyl alcohol foam and ethanol are often employed because they both occlude at the level of the arterioles and capillaries. Medium to small arteries may be occluded with coils, which are the equivalent of surgical ligation. In trauma situations, when only temporary (days) occlusion is the goal, absorbable gelatin pledgets or powder are employed.74
The embolization and sclerotherapy of vascular malformations usually requires a general anesthetic to ensure motionless conditions, especially during high-risk procedures that involve injection of a contrast agent and potentially painful sclerosants. These procedures require careful planning and discussion between the interventional radiologist and the anesthesiologist for safe airway management, intraprocedural and postprocedural care, and disposition. The anesthesiologist must have a basic understanding of the procedure and the risks involved. Some of the risks are inherent to the procedure and the agents used for embolization or sclerotherapy. Pulmonary emboli have been reported after these procedures as a result of the inadvertent migration of embolization material to the lungs. The incidence of pulmonary embolization after cerebral interventions has been reported to be as great as 35% in children after embolization or coiling.75 Before each procedure, the anesthesiologist and radiologist should discuss the radiologist’s specific requirements and concerns and decide on an anesthetic plan. Some procedures, especially embolizations of the head and neck, carry an increased risk of neurologic or cardiovascular complications.81 It is important that the anesthesiologist anticipate these potential complications and modify the anesthetic technique to permit neurologic assessment soon after extubation.
The anesthesiologist should be familiar with the mechanism of action and potential risks associated with the various agents and devices used for embolization and sclerotherapy. All sclerosants produce local hemolysis when administered into the vascular bed. Large amounts of sclerosant, particularly sodium tetradecyl and ethanol, can cause hemoglobinuria, which can result in renal injury if the child is not adequately hydrated during and after the procedure.82 Most children have a urine catheter placed to monitor the urine output, facilitate generous hydration, and monitor for hematuria. The anesthesiologist should generously hydrate the child with intravenous saline. On average, it has been our practice to administer as much as 50 mL/kg intravenous fluids over the course of the procedure. When hemoglobinuria is noted during the procedure, the anesthesiologist should notify the radiologist coincident with increasing the rate of fluid administration (Fig. 45-11). Hemoglobinuria may not occur until the end of the procedure, sometimes after a cumulative large dose of sclerosant has been administered or the tourniquet (if used) has been released. During procedures that involve lower extremity lesions and tourniquets, hemoglobinuria can develop soon after the tourniquet is released. Promoting diuresis with furosemide 0.5 to 1 mg/kg helps resolve gross hematuria. Occasionally, hemoglobinuria develops 1 to 2 hours after the procedure, usually while in the postanesthesia care unit. It is important to carefully balance fluid administered with urine output. In the event of persistent hemoglobinuria, sodium bicarbonate 75 mEq/L in D5W may be administered at two times maintenance rate to alkalinize the urine and minimize precipitation of hemoglobin in the renal tubules.8
Administering ethanol to sclerose a vascular malformation has the potential for severe complications.83 The most infrequent but serious risk is cardiovascular collapse, which is generally preceded by hypoxemia and bradycardia. Most reported cases of cardiovascular collapse involved lower extremity malformations.84 The cause of the cardiovascular collapse associated with ethanol is unclear, although it is reported to occur uniquely when there is direct release of ethanol into the systemic veins. Collapse can occur after release of tourniquets in extremities that have been injected with ethanol. It is critical that the radiologist communicate with the anesthesiologist whenever ethanol is being injected, as well as before the deflation of the tourniquet. It has been recommended that any child at significant risk of systemic ethanol egress should have a pulmonary artery catheter placed before the procedure to identify changes in pulmonary artery pressure that might herald electromechanical dissociation. Significant increases in pulmonary artery pressure resulting from pulmonary vasoconstriction necessitate interrupting the administration of ethanol until the pressure normalizes. Significant fluctuations in vital signs include an increase in pulmonary artery pressure, a decrease in systemic blood pressure, decrease in ETco2, or O2 saturation, cardiac arrhythmias, or full cardiac arrest.
Ethanol can produce a state of intoxication. The ethanol used for embolization and sclerotherapy is 95% to 98% pure. Children who receive greater than 0.75 mL/kg can become clinically intoxicated. Blood levels correlate with the volume of ethanol administered, regardless of the location or type of vascular malformation (Fig. 45-12). On extubation, these children display either significant agitation or excessive sedation and analgesia; opioids should be administered with caution because they have a synergistic effect with ethanol and potentially cause unwanted respiratory depression. It is generally advisable to administer minimal or no opioids until the child is extubated and confirms the presence of pain. Ketorolac may be administered intravenously after soliciting the agreement of the radiologist, removing the invasive catheters, and ensuring hemostasis has been achieved.
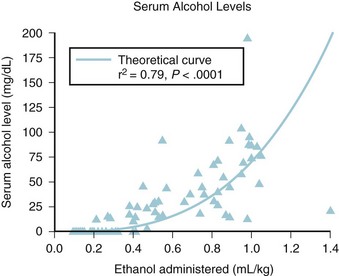
FIGURE 45-12 Positive relationship between serum ethanol level and amount of ethanol administered.
(From Mason KP, Michna E, Zurakowski D, Koka BV, Burrows PE. Serum ethanol levels in children and adults after ethanol embolization or sclerotherapy for vascular anomalies. Radiology 2000;217:127-32.)
Children with vascular malformations, especially venous malformations, can have preexisting coagulation disturbances that resemble disseminated intravascular coagulation.85 Children with laboratory indices consistent with preexisting consumptive coagulopathy should have a hematology consultation and, if possible, receive heparin for 2 weeks before the procedure to replenish their fibrinogen levels. The anesthesiologist may be asked to administer cryoprecipitate or platelets during the procedure to promote clotting and successful sclerosis. The use of ethanol for sclerosis or embolization can also elicit a coagulation disturbance that resembles disseminated intravascular coagulation. There is a statistical relationship between the amount of ethanol administered and the degree of coagulation disturbance elicited.86 Additional cryoprecipitate or fresh frozen plasma transfusions are given only in severe cases, because the coagulopathy is generally not symptomatic and resolves within 5 days. However, major surgery should be deferred until the coagulation parameters have normalized.
It is important to be aware of the potential morbidity associated with embolization procedures. Type and crossmatch should be performed for all children scheduled for embolization in anticipation of possible major hemorrhage. Embolization therapy has been associated with multiple potentially devastating outcomes, including stroke, cerebral abscess, bleeding, cyanosis, and pulmonary embolism.87 Postprocedural mortality is reported to be 5% to 6%.88 It is important to document full return of neurologic status after the child recovers and the trachea is extubated. Vascular malformations involving the airway are particularly challenging (Fig. 45-13). The anesthesiologist and radiologist should review the imaging studies (usually MRI) before the procedure. The scans should be evaluated for patency of the nares, nasopharynx, hypopharynx, and oropharynx, as well as for an uncompromised trachea, carina, and bronchi. MRI will also confirm that there is no malformation in the nares or nasopharynx, which could be damaged or bleed during intubation. Most interventional radiology suites are not situated in the OR. If there is any potential for airway compromise, difficulty in attaining a mask airway, or failure to intubate, the airway should be secured in the OR before transport to the radiology suite. In general, an otolaryngologist or another specialist skilled in bronchoscopy and tracheotomy should be present in the OR prepared to perform bronchoscopy (fiberoptic or rigid) or tracheostomy should the airway be lost.
Intravascular Contrast Media
The use of intravascular contrast media is ubiquitous in interventional radiology and cardiac catheterization. It is important that the anesthesiologist be aware of the risks and potential side effects of these agents. Almost all life-threatening reactions occur immediately or within 20 minutes of contrast agent administration. Low-osmolality ionic and nonionic contrast media are associated with a reduced incidence of adverse events, especially non–life-threatening events compared with high-osmolality contrast media. Serious contrast reactions are estimated at 1 to 2 per 1000 studies using high-osmolality contrast media versus 1 to 2 per 10,000 studies with low osmolality contrast media.89,90 Adverse reactions include nausea, vomiting, hypotension, urticaria, bronchospasm, anxiety, chills, fever, facial flushing, seizures, pulmonary edema, and cardiovascular collapse. Anaphylactic shock is the most worrisome of all contrast reactions and may occur as early as 1 minute and as late as several hours after administration of the contrast material. Although adverse reactions to intravascular contrast media are unpredictable, some risk factors are identifiable. These include children who suffer from allergies or an atopic disease, children with asthma, significant cardiovascular disease, and paraproteinemia, and children with prior contrast reactions have an increased incidence of adverse reactions.91 Children who have been identified as being at increased risk of a contrast reaction should be premedicated with corticosteroids and antihistamines. The American College of Radiology suggests that corticosteroid premedication, along with low osmolality contrast media, should be used for those at risk.91,92
Because the contrast agent is hypertonic relative to plasma, attention needs to be paid to the risk of a triphasic response: initial but transient (30 to 60 seconds) hypotension, followed by hypertension as the contrast material draws fluid intravascularly, followed by a hyperosmotic diuresis with the potential for hypotension. Equilibration with the extracellular fluid compartment occurs within 10 minutes, heralded by the onset of diuresis. For this reason, special attention should be paid when administering iodine contrast to any child with a history of congestive heart failure. The initial increase in blood volume may precipitate cardiovascular compromise. Renal failure from contrast media can occur with high- and low-osmolality contrast media. Although the cause of the acute renal failure is not known, risk factors include preexisting renal insufficiency (serum creatine 1.5 mg/dL or greater), diabetes mellitus, dehydration, cardiovascular disease, hypertension, and hyperuricemia. For these at-risk children, good hydration must be maintained, along with careful follow-up of renal function.93 It should be noted that those with paraproteinemias (multiple myeloma, Waldenström macroglobulinemia) are predisposed to irreversible renal failure from protein precipitation in renal tubules, which is preventable with proper hydration.94
Endoscopic Procedures
Gastrointestinal endoscopy is a common procedure for pediatric gastroenterologists. Both upper and lower endoscopies involve significant stimulation, which requires deep sedation or general anesthesia in infants and children. A range of sedation and anesthesia options may be used for both simple95,96 and more complex procedures such as foreign body removal, endoscopic retrograde cholangiopancreatography (ERCP), and percutaneous endoscopic gastrostomy (PEG) placement.97 The choice of deep sedation versus general anesthesia (with or without a tracheal tube) depends on the medical condition of the child, the risks associated with the specific procedure, and the anticipated duration. Upper esophagogastroduodenoscopy (EGD) in infants (less than 10 kg) generally requires tracheal intubation to avoid airway compression caused by the endoscope pressing the trachealis muscle into the trachea. However, few definitive data exist on this issue (few studies include infants less than 1 year of age). At Dartmouth Hitchcock Medical Center, we have found that if the endoscopist is willing to use the smallest available pediatric scopes, successful sedation or anesthesia without a tracheal tube is possible for infants as small as 6 to 7 kg. In some cases an LMA may be used to secure the airway and the cuff deflated to allow passage of the endoscope and then reinflated once the scope has entered the esophagus. It is advised to hold on to either a tracheal tube or an LMA during the procedure to reduce the risk of the endoscopist dislodging it during their manipulations.
Many techniques may be employed successfully to maintain adequate conditions for upper and lower gastrointestinal endoscopy. Although all anesthesia-delivery areas must meet ASA standards, scavenging and ventilation in endoscopy suites may not always be effective in allowing the safe use of inhalational anesthetics. In these cases, total intravenous anesthesia can substitute for potent inhalation agents.98–100 Over the last several years, various combinations of intravenous agents have been described for the delivery of sedation and anesthesia in the endoscopy suite for children. As a general rule, propofol is used either alone,101 in combination with an opioid (fentanyl or remifentanil),102,103 or with ketamine.104 Outcomes after deep sedation or anesthesia with propofol and inhalational anesthesia were similar.99 However, the time to awakening was more rapid after the inhalational anesthetic, the incidence of agitation on emergence was less, and the time to hospital discharge earlier after propofol.
Upper endoscopies have the inherent risk of apnea, laryngospasm, bronchospasm, and airway obstruction. Most problems resolve after withdrawal of the endoscope and positive-pressure ventilation with a face mask.105 In rare situations, tracheal intubation may be required to complete the procedure or resolve an airway issue. In young infants, abdominal distention secondary to air introduced into the stomach may impair diaphragmatic excursion, leading to hypoventilation. Little published evidence exists on which to base age-related practice in endoscopy sedation and analgesia, experience led several groups to select all infants up to 6 months of age as the age interval for which general anesthesia with tracheal intubation is required, because of a greater frequency of respiratory complications.95,96 When extubating the tracheas in these infants, be wary that residual air in the stomach can impair effective ventilation and contribute to desaturation or O2 dependence in the recovery room. Thus it is important to remind the endoscopist to suction any residual air from the stomach before removing the endoscope.
Few large studies have reported outcomes after pediatric gastrointestinal endoscopy. The Clinical Outcomes Research Initiative (CORI) is a national registry of endoscopic procedures that was started in 1995. The PEDS-CORI is the pediatric component that was started in 1999. A 2007 report collected the complications from pediatric upper endoscopy involving 10,236 encounters from 13 different institutions over 4 years.106 Overall, there was a 2.3% incidence of complications (of any kind). Of these, 79.9% were cardiopulmonary, 18% were gastrointestinal complications, and 5.9% were other complications, including prolonged sedation, drug reactions, or rash. Not surprisingly, children who developed complications were younger and had a greater ASA physical status. General anesthesia was associated with a reduced overall complication rate (1.2%) compared with that in the IV sedation group (3.7%). Although these data were not controlled or randomized, it does shed some insight into the complication rates associated with pediatric sedation outside the OR.
Acknowledgment
We wish to thank Leira P. Mason and Babu V. Koka for their prior contributions to this chapter.
Coté CJ, Wilson S. Guidelines for monitoring and management of pediatric patients during and after sedation for diagnostic and therapeutic procedures: an update. Pediatrics. 2006;118:2587–2602.
Cravero JP, Beach ML, Blike GT, et al. The incidence and nature of adverse events during pediatric sedation/anesthesia with propofol for procedures outside the operating room: a report from the Pediatric Sedation Research Consortium. Anesth Analg. 2009;108:795–804.
Kanal E, Borgstede JP, Barkovich AJ, et al. American College of Radiology White Paper on MR Safety: 2004 update and revisions. AJR Am J Roentgenol. 2004;182:1111–1114.
Shellock FG, Crues JV. MR procedures: biologic effects, safety, and patient care. Radiology. 2004;232:635–652.
1 Cravero JP. Risk and safety of pediatric sedation/anesthesia for procedures outside the operating room. Curr Opin Anaesthesiol. 2009;22:509–513.
2 Cote CJ, Wilson S. Guidelines for monitoring and management of pediatric patients during and after sedation for diagnostic and therapeutic procedures: an update. Pediatrics. 2006;118:2587–2602.
3 Mohr JJ, Barach P, Cravero JP. Microsystems in healthcare. VI. Designing safety into microsystems. Joint Comiss J Quality Safety. 2003;29:401–408.
4 Gozal D, Drenger B, Levin PD. A pediatric sedation/anesthesia program with dedicated care by anesthesiologists and nurses for procedures outside the operating room. J Pediatr. 2004;145:47–52.
5 Bell C, Sequeira M. Non operating room anesthesia for children. Curr Opin Anesthesiol. 2005;18:271–276.
6 Adams K, Pennock N, Phelps B, Rose W, Peters M. Anesthesia services outside of the operating room. Pediatr Nurs. 2007;33:232–237.
7 Alspach D, Falleroni M. Monitoring patients during procedures conducted outside the operating room. Int Anesthesiol Clin. 2004;42:95–111.
8 Standards for basic anesthetic monitoring. Committee of origin: Standards and Practice Parameters. Approved by the ASA House of Delegates on Oct. 21, 1986, and last amended on Oct. 20, 2010, with an effective date of July 1. http://www.asahq.org/For-Members/Standards-Guidelines-and-Statements.aspx, 2011. Available at (accessed Oct. 21, 2012)
9 Koskinen MF. Magnetic resonance imaging MRI site planning and economics. Med Instrum. 1985;19:244–247.
10 Bell C, Sequeira PM. Nonoperating room anesthesia for children. Curr Opin Anaesthesiol. 2005;18:271–276.
11 The Joint Commission. Anesthesia and sedation: the Joint Commission resources. Oakbrook Terrace, Ill: The Joint Commission; 2001.
12 Schulte-Uentrop L, Goepfert MS. Anesthesia or sedation for MRI in children. Curr Opin Anesthesiol. 2010;23:513–517.
13 Elitsur Y, Blankenship P, Lawrence Z. Propofol sedation for endoscopic procedures in children. Endoscopy. 2000;32:788–791.
14 Ibrahim A-S, Mack D. Propofol and remifentanil for deep sedation in children undergoing gastrointestinal endoscopy. Pediatr Anesth. 2007;17:460–463.
15 Cravero JP, Blike GT, Surgenor SD, Jensen J. Development and validation of the Dartmouth operative conditions scale. Anesth Analg. 2004;100:1614–1621.
16 Tait AR, Pandit UA. Use of the laryngeal mask airway in children with upper respiratory tract infections: a comparison with endotracheal intubation. Anesth Analg. 1998;86:706–711.
17 Ferrari LR, Goudsouzian NG. The use of the laryngeal mask airway in children with bronchopulmonary dysplasia. Anesth Analg. 1995:310–313.
18 Fujii Y, Saitoh Y, Tanaka H, Toyooka H. Cardiovascular responses to tracheal extubation or LMA removal in children. Can J Anaesth. 1998;45:178–181.
19 Heard C, Burrows F, Johnson K, et al. A comparison of dexmedetomidine-midazolam with propofol for maintenance of anesthesia in children undergoing magnetic resonance imaging. Anesth Analg. 2008;107:1832–1839.
20 Blike GT, Christoffersen K, Cravero JP, Andeweg SK, Jensen J. A method for measuring system safety and latent errors associated with pediatric procedural sedation. Anesth Analg. 2005;101:48–58.
21 Practice advisory on anesthetic care for magnetic resonance imaging: a report by the American Society of Anesthesiologists Task Force on Anesthetic Care for Magnetic Resonance Imaging. Anesthesiology. 2009;110:459–479.
22 Schmidt U, Eikermann M. Organizational aspects of difficult airway management: think globally act locally. Anesthesiology. 2011:114.
23 Hata T, Todd MM. Cervical spine considerations when anesthetizing patients with Down syndrome. Anesthesiology. 2005;102:680–685.
24 Donnelly LF, Frush DP, Frush KS. Aspirated contrast material contributing to respiratory arrest in a pediatric trauma patient. AJR Am J Roentgenol. 1998;171:471–473.
25 Trulzsch DV, Penmetsa A, Karim A, Evans DA. Gastrografin-induced aspiration pneumonia: a lethal complication of computed tomagraphy. South Med J. 1992;85:1255–1256.
26 Tuladhar R, Patole S, Whitehall J. Gastrografin aspiration in a neonate with tracheoesophageal fistula. J Paediatr Child Health. 2000;36:94–95.
27 Lim-Dunham JE, Narra J, Benya EC, Donaldson JS. Aspiration after administration of oral contrast material in children undergoing abdominal CT for trauma. AJR Am J Roentgenol. 1997;169:1015–1018.
28 Ziegler MA, Fricke BL, Donnelly LF. Is administration of enteric contrast material safe before abdominal CT in children who require sedation? Experience with chloral hydrate and pentobarbital. Am J Roentgenol. 2003;180:13–15.
29 Shulkin BL. PET imaging in pediatric oncology. Pediatr Radiol. 2004;34:199–204.
30 Seo JH, Holland K, Rose D, et al. Multimodality imaging in the surgical treatment of children with nonlesional epilepsy. Neurology. 2011;76:41–48.
31 Gaillard WD, Weinstein S, Conry J, et al. Prognosis of children with partial epilepsy: MRI and serial 18FDG-PET. Neurology. 2007;68:655–659.
32 Ollenberger GP, Byrne AJ, Berlangieri SU, et al. Assessment of the role of FDG PET in the diagnosis and management of children with refractory epilepsy. Eur J Nucl Med Mol Imaging. 2005;32:1311–1316.
33 Krieger MD, McComb JG. The role of stereotactic radiotherapy in the management of ependymomas. Childs Nerv Syst. 2009;25:1269–1273.
34 Foy AB, Wetjen N, Pollock BE. Stereotactic radiosurgery for pediatric arteriovenous malformations. Neurosurg Clin N Am. 2010;21:457–461.
35 Coffey RJ, Lunsford LD, Flickinger JC. The role of radiosurgery in the treatment of malignant brain tumors. Neurosurg Clin N Am. 1992;3:231–244.
36 Stokes MA, Soriano SG, Tarbell NJ, et al. Anesthesia for stereotactic radiosurgery in children. J Neurosurg Anesthesiol. 1995;7:100–108.
37 Latham GJ, Greenberg RS. Anesthetic considerations for the pediatric oncology patient. 1. A review of antitumor therapy. Paediatr Anaesth. 2010;20:295–304.
38 Harris EA. Sedation and anesthesia options for pediatric patients in the radiation oncology suite. Int J Pediatr. 2010:870–921.
39 Weiss M, Frei M, Buehrer S, et al. Deep propofol sedation for vacuum-assisted bite-block immobilization in children undergoing proton radiation therapy of cranial tumors. Paediatr Anaesth. 2007;17:867–873.
40 Buehrer S, Immoos S, Frei M, Timmermann B, Weiss M. Evaluation of propofol for repeated prolonged deep sedation in children undergoing proton radiation therapy. Br J Anaesth. 2007;99:556–560.
41 Anghelescu DL, Burgoyne LL, Liu W, et al. Safe anesthesia for radiotherapy in pediatric oncology: St. Jude Children’s Research Hospital Experience, 2004-2006. Int J Radiat Oncol Biol Phys. 2008;71:491–497.
42 Cravero JP, Beach ML, Blike GT, et al. The incidence and nature of adverse events during pediatric sedation/anesthesia with propofol for procedures outside the operating room: a report from the Pediatric Sedation Research Consortium. Anesth Analg. 2009;108:795–804.
43 Shukry M, Ramadhyani U. Dexmedetomidine as the primary sedative agent for brain radiation therapy in a 21-month old child. Paediatr Anaesth. 2005;15:241–242.
44 Pradhan DG, Sandridge AL, Mullaney P, et al. Radiation therapy for retinoblastoma: a retrospective review of 120 patients. Int J Radiat Oncol Biol Phys. 1997;39:3–13.
45 Barnes PD, Krasnokutsky M. Imaging of the central nervous system in suspected or alleged nonaccidental injury, including the mimics. Top Magn Reson Imaging. 2007;18:53–74.
46 Levine D, Barnes PD, Robertson RR, Wong G, Mehta TS. Fast MR imaging of fetal central nervous system abnormalities. Radiology. 2003;229:51–61.
47 Barnes PD. Imaging of the central nervous system in pediatrics and adolescence. Pediatr Clin North Am. 1992;39:743–776.
48 Zimmerman RA, Bilaniuk LT, Pollock AN, et al. 3.0 T versus 1.5 T pediatric brain imaging. Neuroimaging Clin N Am. 2006;16:229–239. ix
49 Chaudhary N, Davagnanam I, Ansari SA, et al. Imaging of intracranial aneurysms causing isolated third cranial nerve palsy. J Neuroophthalmol. 2009;29:238–244.
50 Rea D, Brandsema JF, Armstrong D, et al. Cerebral arteriopathy in children with neurofibromatosis type 1. Pediatrics. 2009;124:e476–e483.
51 Kanal E, Barkovich AJ, Bell C, et al. ACR guidance document for safe MR practices: 2007. AJR Am J Roentgenol. 2007;188:1447–1474.
52 Boutin RD, Briggs JE, Williamson MR. Injuries associated with MR imaging: survey of safety records and methods used to screen patients for metallic foreign bodies before imaging. AJR Am J Roentgenol. 1994;162:189–194.
53 Chaljub G, Kramer LA, Johnson RF, 3rd., et al. Projectile cylinder accidents resulting from the presence of ferromagnetic nitrous oxide or oxygen tanks in the MR suite. AJR Am J Roentgenol. 2001;177:27–30.
54 Hartnell GG, Spence L, Hughes LA, et al. Safety of MR imaging in patients who have retained metallic materials after cardiac surgery. AJR Am J Roentgenol. 1997;168:1157–1159.
55 Patel MR, Albert TS, Kandzari DE, et al. Acute myocardial infarction: safety of cardiac MR imaging after percutaneous revascularization with stents. Radiology. 2006;240:674–680.
56 Shellock FG. Reference Manual for Magnetic Resonance Safety, Implants and Devices. Los Angeles: Biomedical Research Publishing Group; 2006.
57 Food and Drug Administration, Center for Devices and Radiological Health MR Product Reporting Pogram, Washington, DC, Food and Drug Administration, 2012, http://www.fda.gov/Safety/MedWatch/default.htm. accessed Oct. 21 Available at
58 Achenbach S, Moshage W, Diem B, et al. Effects of magnetic resonance imaging on cardiac pacemakers and electrodes. Am Heart J. 1997;134:467–473.
59 Loewy J, Loewy A, Kendall EJ. Reconsideration of pacemakers and MR imaging. Radiographics. 2004;24:1257–1267. discussion 67-8
60 Fontaine JM, Mohamed FB, Gottlieb C, Callans DJ, Marchlinski FE. Rapid ventricular pacing in a pacemaker patient undergoing magnetic resonance imaging. Pacing Clin Electrophysiol. 1998;21:1336–1339.
61 Martin ET, Coman JA, Shellock FG, et al. Magnetic resonance imaging and cardiac pacemaker safety at 1.5-Tesla. J Am Coll Cardiol. 2004;43:1315–1324.
62 Brummett RE, Talbot JM, Charuhas P. Potential hearing loss resulting from MR imaging. Radiology. 1988;169:539–540.
63 U.S. Department of Health and Huyman Services, Food and Drug Administration: Guidelines for Evaluating Electromagnetic Exposure Risk for Trials of Clinical NMR Systems. Washington, DC, 1982.
64 Bryan YF, Templeton TW, Nick TG, Szafran M, Tung A. Brain magnetic resonance imaging increases core body temperature in sedated children. Anesth Analg. 2006;102:1674–1679.
65 Kussman BD, Mulkern RV, Holzman RS. Iatrogenic hyperthermia during cardiac magnetic resonance imaging. Anesth Analg. 2004;99:1053–1055. table of contents
66 Mason KP, Burrows PE, Dorsey MM, Zurakowski D, Krauss B. Accuracy of capnography with a 30 foot nasal cannula for monitoring respiratory rate and end-tidal CO2 in children. J Clin Monit Comput. 2000;16:259–262.
67 Shellock FG, Slimp GL. Severe burn of the finger caused by using a pulse oximeter during MR imaging. AJR Am J Roentgenol. 1989;153:1105.
68 Yoshikawa K, Davies A. Safety of ProHance in special populations. Eur Radiol. 1997;7(Suppl 5):246–250.
69 Murphy KJ, Brunberg JA, Cohan RH. Adverse reactions to gadolinium contrast media: a review of 36 cases. AJR Am J Roentgenol. 1996;167:847–849.
70 Belik J, Musey J, Trussell RA. Continuous infusion of glucagon induces severe hyponatremia and thrombocytopenia in a premature neonate. Pediatrics. 2001;107:595–597.
71 Soriano SG, Sethna NF, Scott RM. Anesthetic management of children with moyamoya syndrome. Anesth Analg. 1993;77:1066–1070.
72 Nomura S, Kashiwagi S, Uetsuka S, et al. Perioperative management protocols for children with moyomoya disease. Child’s Nervous System. 1999;17:270–274.
73 Millar C, Bissonnette B, Humphreys RP. Cerebral arteriovenous malformations in children. Can J Anesth. 1994;41:321–331.
74 Coldwell DM, Stokes KR, Yakes WF. Ebolotherapy: agents clinical applications, and techniques. RadioGraphics. 1994;14:623–643.
75 Kjellin IB, Boechat MI, Vinuela SJ, Westra SJ, Duckwiler GR. Pulmonary emboli following therapeutic embolization of cerebral arterivenous malformations in children. Pediatr Radiol. 2000:279–283.
76 Bryan YF, Hoke LK, Taghon TA, et al. A randomized trial comparing sevoflurane and propofol in children undergoing MRI scans. Paediatr Anaesth. 2009;19:672–681.
77 McFarlan CS, Anderson BJ, Short TG. The use of propofol infusions in paediatric anaesthesia: a practical guide. Paediatr Anaesth. 1999:9209–9216.
78 Cravero J, Surgenor S, Whalen K. Emergence agitation in paediatric patients after sevoflurane anaesthesia and no surgery: a comparison with halothane. Paediatr Anaesth. 2000;10:419–424.
79 Murray DJ, Schmid CM, Forbes RB. Anesthesia for magnetic resonance imaging in children: a low incidence of protracted post-procedure vomiting. J Clin Anesth. 1995;7:232–236.
80 Miller RE, Chernish SM, Brunelle RL, Rosenak BD. Double-blind radiographic study of dose response to intravenous glucagon for hypotonic duodenography. Radiology. 1978;127:55–59.
81 Do YS, Yakes WF, Shin SW. Ethanol embolization of arteriovenous malformation: interim results. Radiology. 2005;235:674–682.
82 Berenguer B, Burows PE, Zurakowski D, Miliken JB. Sclerotherapy of craniofacial venous malformations: complications and results. Plast Reconstr Surg. 1999;104:1–11.
83 Burrows PE, Mason KP. Percutaneous treatment of low flow vascular malformations. J Vasc Interv Radiol. 2004;15:431–445.
84 Yakes W. Cardiopulmonary collapse: sequelae of ethanol embolotherapy [abstract]. Radiology. 1993;189:145.
85 Aronoff DM, Roshon M. Severe hemorrhage complicating the Klippel-Tenaunay-Weber syndrome. South Med J. 1998;91:1073–1075.
86 Mason KP, Neufeld EJ, Karian VE, et al. Coagulation abnormalities in pediatric and adult patients after sclerotherapy or embolization of vascular abnormalities. Am J Roentgenol. 2001;177:1359–1363.
87 Faughnan ME, Thabet A, Mel-Zahav M, et al. Pulmonary arteriovenous malfomations in children: outcomes of transcatheter embolotherapy. J Peds. 2004;145:826–831.
88 Weon YC, Yoshida Y, Sachet M, et al. Supratentorial cerebral arteriovenous fistulas in children: review of 41 cases with 63 non choroidal single-hole AVF’s. Acta Neurochir (Wien). 2005;147:17–31.
89 Caro JJ, Trindade E, McGregor M. The risks of death and of severe nonfatal reactions with high- vs. low-osmolality contrast media: a meta-analysis. Am J Roentgenol. 1991;156:825–832.
90 Spring DB, Bettmann MA, Barkan HE. Deaths related to iodinated contrast media reported spontaeously to the U. S. Food and Drug Administration, 1978-1994: effect of the availability of low-soosmolality contrast media. Radiology. 1997;204:333–337.
91 Manual on Contrast Media Version 5.0. Reston, Va: American College of Radiology, 2004.
92 Seymour R, Halpin SF, Hardman JA. Corticosteroid pretreatment and nonionic contrast media. Acta Neurochir (Wien). 1995;164:508–509.
93 Aspelin P, Aubry P, Fansson SG, et al. Nephrotoxic effects in high-risk patients undergoing angiography. N Engl J Med. 2003;348:491–499.
94 McCathy CS, Becker JA. Miltiple myeloma and contrast media. Radiology. 1992;183:519–521.
95 Bouchut JC, Gadard J, Lachaux A, Diot N. Deep sedation for upper gastrointestinal endoscopy in children. J Pediatr Gastroenterol. 2001;32:108.
96 Koh JL, Black DD, Leatherman IK, Harrison RD, Schmitz ML. Experience with an anesthesiologist interventional model for endoscopy in a pediatric hospital. J Pediatr Gastroenterol Nutr. 2001;33:314–318.
97 Wengrower D, Gozal D, Gozal Y, et al. Complicated endoscopic pediatric procedures using deep sedation and general anesthesia are safe in the endoscopy suite. Scand J Gastroenterol. 2004;39:283–286.
98 Gilger MA. Sedation for pediatric GI endoscopy. Gastrointest Endosc. 2007;65:211–212.
99 Kaddu R, Bhattacharya D, Metriyakool K, Thomas R, Vasundhara R. Proporol compared with general anesthesia for pediatric GI endoscopy: is propofol better? Gastrointest Endosc. 2002;55:27–32.
100 Schwarz SM, Lightdale JR, Liacouras CA. Sedation and anesthesia in pediatric endoscopy:one size does not fit all. J Pediatr Gastroenterol Nutr. 2007;44:295–297.
101 Barbi E, Petaros P, Badina L, et al. Deep sedation with propofol for upper gastrointestinal endoscopy in children, administered GBY specially trained pediatricians: a prospective case series with emphasis on side effects. Endoscopy. 2006;38:368–375.
102 Abu-Shahwan I, Mack D. Propofol and remifentanil for deep sedation in children undergoing gastrointestinal endoscopy. Paediatr Anaesth. 2007;17:460–463.
103 Michaud L. Sedation for diagnostic upper gastrointestinal endoscopy: a survey of the Fancophone Pediatric Hepatology, Gastroenterology, and Nutrition Group. Endoscopy. 2005;37:167–170.
104 Tosun Z, Aksu R, Guler G, et al. Propofol-ketamine vs. propofol fentanyl for sedation during pediatric upper gastrointestinal endoscopy. Paediatr Anaesth. 2007;10:983–988.
105 Amournyotin S, Aanpreung P, Prakarnrattana U, et al. Experience of intravenous sedation for pediatric gastrointestinal endoscopy in a large tertiary referral center in a developing country. Paediatr Anaesth. 2009;19:784–791.
106 Thakkar K, El-Serag HB, Mattek N, Gilger MA. Complications of pediatric EGD: a 4 year experience in PEDS-CORI. Gastrointest Endosc. 2007:65.