150 Anemia and Red Blood Cell Transfusion in Critically Ill Patients
Anemia is a common problem in critically ill patients admitted to intensive care units (ICUs).1 Indeed, in a recent cross-sectional study, 29% of patients had a hemoglobin concentration below normal values, and 37% required a red blood cell (RBC) transfusion.2 Allogeneic RBC transfusions are complex biological products prepared from individual blood donations and are unique in many respects when compared with other health interventions. Decisions concerning the use of RBC transfusion in the treatment of anemia and hemorrhage require a clear understanding of the risks and benefits of both the condition and its treatment. Although we have developed a much clearer appreciation of the infectious and immunomodulatory risks of RBC transfusion over the past 2 decades, the risks of anemia in many clinical settings and the benefits of RBC transfusion are still inadequately characterized. We presume that the most significant risk associated with anemia is the harm resulting from the decrease in oxygen-carrying capacity and plasma volume. Development of adverse health consequences from anemia will in part depend on the capacity of the individual patient to compensate for these changes. The benefit of transfusion refers to the capacity of RBCs to correct these risks and possibly provide additional benefits such as increasing oxygen delivery to supranormal ranges. Such a framework highlights the concept of tradeoffs of risks and benefits. With the exception of patients who refuse blood for religious reasons, it is impossible outside a randomized clinical trial to distinguish clearly between these competing risks and benefits to patients.
Natural History of Uncorrected Anemia
Numerous laboratory experiments indicate that extreme hemodilution is well tolerated in healthy animals. Animals subjected to acute hemodilution tolerate decreasing hemoglobin concentrations down to 50 to 30 g/L, with ischemic electrocardiographic changes and depressed ventricular function, respectively, occurring at these levels of hemoglobin concentration.3 However, acute hemodilution is less well tolerated in experimental animal models of coronary stenosis, with ischemic electrocardiographic changes and depressed cardiac function occurring at hemoglobin concentrations between 70 and 100 g/L. Human data regarding the limits of anemia tolerance are inadequate and often conflicting. Leung et al.4 found electrocardiographic changes that may have been indicative of myocardial ischemia in 3 of 55 conscious resting volunteers subjected to acute isovolemic hemodilution to a hemoglobin concentration of 50 g/L.
While providing insight into the human physiologic response to acute anemia, the experimental data mentioned are of limited applicability to the perioperative setting, where many of the factors that influence oxygen consumption—muscle activity, body temperature, heart rate, sympathetic activity, metabolic state—are altered. Instead we need to determine the risk of withholding RBC transfusions in the perioperative setting. From a systematic review completed for the Canadian Guidelines on Red cells, Hébert and associates5 identified numerous reports of severe anemia being well tolerated in surgical patients.6,7 Additional reports or case series8,9–11 describe successful outcomes in patients with chronic anemia due to renal failure. Finally, descriptive studies in patients refusing red blood cell transfusion12–1415 and from regions experiencing limited blood supplies16,17 have demonstrated that patients can survive surgical interventions with hemoglobin levels as low as 45 g/L.
In examining some of these studies in more detail, there appears to be an association between preoperative hemoglobin concentrations, intraoperative estimated blood loss, and postoperative mortality.13,14 Indeed, there were no reported deaths in more than 100 patients undergoing major elective surgery when preoperative hemoglobin concentrations were above 80 g/L and the estimated blood loss was less than 500 mL. In a single-center series of 542 Jehovah’s Witness patients undergoing a cardiac surgical procedure, the overall mortality rate was 10.7%; only 2.2% of the deaths observed were considered to be a direct consequence of anemia. More recently, Viele and Weiskopf 7 identified 134 Jehovah’s Witness patients with a hemoglobin concentration less than 80 g/L or a hematocrit below 24% who were treated for various medical and surgical conditions without the use of blood or blood components. There were 50 reported deaths, 23 of which were attributed primarily or exclusively to anemia (defined as deaths with hemoglobin concentration < 50 g/L). For those patients who died of their anemia, 60% were older than 50 years. However, in 27 survivors with hemoglobin concentration below 50 g/L, 65% were younger than 50. Although publication bias must be kept in mind in examining these data, young healthy patients may survive without transfusion at hemoglobin concentrations in the range of 50 g/L. From these data, it is clear that extreme anemia is often tolerated in the perioperative setting but also appears to increase the risk of death. However, these observations should not be interpreted as support for a restrictive or conservative transfusion strategy, especially because most of the literature related to tolerance of anemia has not explored patient characteristics that predispose patients to adverse outcomes from moderate to severe anemia.
Anemia in High-Risk Groups
A number of risk factors for adverse outcomes associated with anemia have been identified in clinical practice guidelines18–20 and reviews.21–23 Anemia is thought to be less tolerated in older patients, in the severely ill, and in patients with clinical conditions such as coronary, cerebrovascular, or respiratory disease. However, the clinical evidence confirming that these factors are independently associated with an increased risk of adverse outcome is lacking. One small case-control study following high-risk vascular surgery suggests an increase in postoperative cardiac events with increasing severity of anemia.24 In perioperative25 and critically ill patients,26 two large cohort studies have documented that increasing degrees of anemia were associated with a disproportionate increase in mortality rate in the subgroup of patients with cardiac disease. In 1958 Jehovah’s Witness patients,25 the adjusted odds of death increased from 2.3 (95% confidence interval [CI], 1.4-4.0) to 12.3 (95% CI, 2.5-62.1) as preoperative hemoglobin concentrations declined from the range of 100 to 109 g/L to the range of 60 to 69 g/L in patients with cardiac disease (Figure 150-1). There was no significant increase in mortality in noncardiac patients with comparable levels of anemia. In a separate study of critically ill patients,26 those with cardiac disease and hemoglobin concentrations less than 95 g/L also had a trend toward an increased mortality rate (55% versus 42%; P = .09) as compared with anemic patients with other diagnoses. Although both cohort studies were retrospective in nature and may not have controlled for a number of important confounders, the evidence suggests that anemia increases the risk of death in patients with significant cardiac disease.
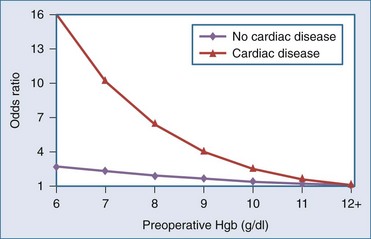
Figure 150-1 Adjusted odds ratio for mortality by cardiovascular disease and preoperative hemoglobin (Hgb).
(Adapted from Carson JL, Spence RK, Poses RM, Bonavita G. Severity of anemia and operative mortality and morbidity. Lancet 1988;1:727–9.)
Severity of illness also appears to be a risk factor in critically ill patients.13,26 Two retrospective studies document that degree of blood loss contributes to perioperative mortality.13,26 However, there are no studies examining the independent contributions of age, cerebrovascular disease, and respiratory disease to an increased mortality risk in anemic patients. This relationship may well be complex, given that age and cerebrovascular disease are risk factors associated with coronary artery disease. Smoking-related respiratory diseases may have similar associations to cardiac disease. Therefore, the association between anemia and increased rates of adverse outcomes in these patients can best be described as speculative at this time.
Risks and Benefits of Transfusion
Five large observational studies that were specifically designed to compare clinical outcomes at varying hemoglobin concentrations in transfused and nontransfused patients have been conducted in various clinical settings. In the first of these, Hébert and colleagues26 used a combined retrospective and prospective cohort design to examine 4470 critically ill patients admitted to 6 Canadian tertiary-level ICUs during 1993. After controlling for disease severity, there remained a trend toward increased mortality when hemoglobin concentrations were less than 95 g/L in patients with cardiac diagnoses (ischemic heart disease, arrhythmia, cardiac arrest, or cardiac and vascular surgical procedures). Furthermore, analysis of a subgroup of 202 patients with anemia, an Acute Physiology and Chronic Health Evaluation (APACHE) II score above 20, and a cardiac diagnosis revealed that transfusion of 1 to 3 units or 4 to 6 units of RBCs was associated with a significantly lower mortality rate as compared with those patients who did not receive a transfusion (55% [no transfusions] versus 35% [1-3 units] or 32% [4-6 units]; P = .01).
Wu et al.27 retrospectively studied Medicare records of 78,974 patients older than age 65 who were hospitalized with a primary diagnosis of acute myocardial infarction. The authors then categorized patients according to their admitting hematocrit. Although anemia, defined in the study as a hematocrit less than 39%, was present in nearly half the patients, only 3680 patients received an RBC transfusion. Lower admission hematocrit values were associated with increased 30-day mortality rate, with a mortality rate approaching 50% among patients with a hematocrit of 27% or lower who did not receive an RBC transfusion. Unfortunately, this study had no data on nadir hemoglobins and their relationship to mortality. Interestingly, RBC transfusion was associated with a reduction in 30-day mortality for patients who received at least one RBC transfusion if their admitting hematocrit was less than 33%, whereas RBC transfusion was associated with increased 30-day mortality for patients whose admitting hematocrit values were 36.1% or higher. These associations were present even when adjustments were made for clinical patient factors including APACHE II scores, location of myocardial infarction, and presence of congestive heart failure; as well as treatment factors including use of reperfusion therapies, aspirin, and β-adrenergic blockade.
In the only study exclusively focusing on the perioperative period, Carson and associates28 attempted to determine the effect of perioperative transfusion on 30- and 90-day postoperative mortality with a retrospective cohort study involving 8787 patients with hip fractures undergoing repair between 1983 and 1993 in 20 different U.S. hospitals. This was a large, high-risk, elderly (median age 80.3 years) population with extensive coexisting disease and with an overall 30-day mortality rate of 4.6%. A total of 3699 patients (42%) received a perioperative transfusion within 7 days of the surgical repair. After controlling for trigger hemoglobin concentrations, cardiovascular disease, and other risk factors for death, the results suggested that patients who had hemoglobin concentrations as low as 80 g/L and did not receive transfusion were no more likely to die than those with similar hemoglobin concentration levels who received a transfusion. (With hemoglobin concentrations less than 80 g/L, nearly all patients received a transfusion, so investigators were unable to draw conclusions about the effect of transfusion at these lower hemoglobin concentrations levels.) However, as the authors point out, despite the large sample size, inadequate power may still explain the inability to detect a reduction in mortality related to transfusion, and they estimated that the study would need to be 10 times larger to detect a 10% difference in 30-day mortality with 80% power.
Vincent et al.29 completed a prospective observational cross-sectional study involving 3534 patients admitted to 146 western European ICUs during a 2-week period in November 1999. Of these patients, 37% received an RBC transfusion during their ICU admission, with the overall transfusion rate increasing to 41.6% over a 28-day period. For those patients who received a transfusion, the mean pretransfusion hemoglobin concentration was 84 ± 13 g/L. In an effort to control for confounding factors created by illness severity and the need for transfusion, these investigators used a strategy of matching transfused and nontransfused patients based on their propensity to receive a transfusion, thereby defining two well-balanced groups (516 patients in each group) to determine the influence of RBC transfusions on mortality. Using this approach, the associated risk of death was increased instead of decreased by 33% for patients who received a transfusion compared to similar patients who did not receive blood. However, as pointed out in the accompanying editorial,30 the results may have differed if the propensity scores were derived separately for categories of pretransfusion hemoglobin concentrations (e.g., <80, 80-100, and >100 g/L) instead of hemoglobin concentrations at ICU admission. For example, if one were to consider groups of patients with a pretransfusion hemoglobin concentration of less than 60 g/L, it is unlikely that the observed 33% increase in mortality would hold true, or blood transfusion would never be recommended.
Numerous observational studies have examined the relationship between RBC transfusions and morbidity and mortality in critically ill patients. These studies have been recently summarized in a systematic review by Marik and Corwin31; 45 studies (including the previous 4 studies) involving 272,596 trauma, surgery, cardiac, and ICU patients were identified. Overall, 42 studies showed an increase in at least one of the outcomes of interest. Of the 18 studies that reported the relationship between RBC transfusions and mortality, 17 found an association between RBC transfusion and mortality, with a pooled OR of 1.7; 95% CI, 1.4-1.9. While these findings suggest that the risks associated with RBC transfusion may outweigh the benefits, the authors suggest caution in interpreting these data. Multivariate analysis was used in many of the observational studies to control for other clinical variables, but it is not possible to control for all factors, especially the fact that blood transfusion itself is a marker for severity of illness. The complex interrelationship between disease severity, number of transfusions, and degree of anemia may result in a spurious association reported between increased mortality risk and anemia or RBC transfusion. Thus, randomized controlled trials are required to definitively determine the relationship between RBC transfusions and mortality.
Unfortunately, as evidenced by a recent systematic review, there is a paucity of clinical trials comparing restrictive to liberal transfusion studies to examine the efficacy of RBC transfusion. Carson et al.32 (Figure 150-2) were able to identify only 10 randomized clinical trials of adequate methodological quality in which different RBC transfusion triggers were evaluated. Included were a total of 1780 surgery, trauma, and ICU patients enrolled in trials conducted over the past 40 years. The transfusion triggers evaluated in these trials varied between 70 and 100 g/L. Data on mortality or hospital length of stay were available in only 6 of these trials. Conservative (low hemoglobin) transfusion triggers were not associated with an increase in mortality rate; on average, the rate of mortality was one-fifth lower (relative risk [RR], 0.80; 95% CI, 0.63-1.02) with conservative as compared with liberal transfusion triggers. Likewise, cardiac morbidity and length of hospital stay did not appear to be adversely affected by the lower rate of RBC transfusions. There were insufficient data on potentially relevant clinical outcomes such as stroke, thromboembolism, multiorgan failure, delirium, infection, and delayed wound healing to perform any pooled analysis. The Carson review28 stated there were insufficient data to address the full range of risks and benefits associated with different transfusion thresholds, particularly in patients with coexisting disease. They also noted that their meta-analysis was dominated by a single trial: the Transfusion Requirements in Critical Care (TRICC) trial,33 which enrolled 838 patients and was the only individual trial identified that was adequately powered to evaluate the impact of different transfusion strategies on mortality and morbidity.
The TRICC Study33 documented an overall non-significant trend toward decreased 30-day mortality (18.7 versus 23.3%; P = .11) and significant decreases in mortality among patients who were less acutely ill (8.7 versus 16.1%; P = .03) in the group treated using a hemoglobin transfusion trigger of 70 g/L compared with a more liberally transfused group that received 54% more RBC transfusions. The investigators also noted that the 30-day mortality rates were significantly lower with the restrictive transfusion strategy among patients who were less acutely ill (APACHE II scores less than 20) and among patients who were younger than 55 years of age (Figure 150-3).
A number of additional questions arose from the TRICC trial. The investigators were particularly interested in the risks and benefits of anemia and transfusion in patients with cardiovascular disease and in patients attempting to wean from mechanical ventilation. In the first of these subgroup analyses,34 357 patients (43%) were identified with cardiovascular disease. Of these, 160 had been in the restrictive RBC transfusion group and 197 in the liberal transfusion group. The two groups were fairly equally balanced with regard to baseline characteristics and concurrent therapies, with a few exceptions: there was less frequent diuretic use in the restrictive group (43% versus 58%; P < .01), and the use of epidural anesthetics was greater in the restrictive group (8% versus 2%; P < .01). Overall, in this subgroup analysis, there was no significant difference in the mortality rate between the two treatment groups. However, there was a non-significant (P = .3) decrease in overall survival rate in the restrictive group for patients with confirmed ischemic heart disease, severe peripheral vascular disease, or severe comorbid cardiac disease.
The subgroup analysis of patients receiving mechanical ventilation was limited to 713 (85% of the 838 patients in the TRICC trial who required invasive mechanical ventilatory support).35 Of these, 357 had been in the restrictive RBC transfusion group and 356 in the liberal group. The mean duration of mechanical ventilation was 8.3 ± 8.1 days in the restrictive group and 8.8 ± 8.7 days in the liberal group (P = .48). Ventilator-free days were 17.5 ± 10.9 and 16.1 ± 11.4 in the restrictive and liberal RBC transfusion groups, respectively (P = .09); 82% of the patients in the restrictive transfusion group were considered successfully weaned and extubated for at least 24 hours, compared with 78% in the liberal group (P = .19). Among the 219 patients who required mechanical ventilation for more than 7 days, there were no differences in the time to successful weaning (Figure 150-4). The independent effects of RBC transfusions and hemoglobin concentration were also examined. Each additional transfusion was associated with an increased duration of mechanical ventilation (RR, 1.10; 95% CI, 1.14-1.06; P < .01) after adjusting for the effect of age, APACHE II score, and comorbid illnesses. Hemoglobin concentrations did not influence the duration of mechanical ventilation (RR, 0.99; 95% CI, 1.01-0.98; P = .45). Complications including pulmonary edema and acute respiratory distress syndrome (ARDS) were increased in patients in the liberal strategy group.
Recently, two other large randomized clinical trials have compared liberal and restrictive transfusion strategies in different populations. Lacroix and colleagues36 undertook a non-inferiority trial comparing a restrictive (7.0 g/L) and a liberal (9.5 g/L) transfusion threshold in 648 pediatric ICU patients. There were no differences in new or progressive multiple-organ dysfunction (12% in both groups; absolute RR, 0.4%; 95% CI, −4.6 to 5.4) or the number of deaths (14 in each group).
Even though three large randomized controlled trials have been completed, a number of questions remain to be answered. One of the most important questions is why the liberal RBC transfusion strategy failed to improve 30-day mortality rate and rates of organ failure in critically ill patients. It is conceivable that the greater number of allogeneic RBC units in the liberal group significantly depressed host immune responses35,37 or resulted in altered microcirculatory flow as a consequence of prolonged storage times.
Subsequent to the publication of the TRICC trial, a study by Rivers et al.38 documented that the use of early goal-directed care based on a mixed central venous saturation (ScvO2) decreased mortality from 46.5% in the control group to 30.5% in the goal-directed therapy group (P = .009). As one of the many interventions in patients with early septic shock, hematocrits were increased to greater than 30% if the ScvO2 fell to less than 70%. As a consequence of goal-directed therapy, 64% of patients, compared to 18.5% of the control group, received RBC transfusions (P < .0001). The significant differences in patient populations studied by Rivers and colleagues and the TRICC trial may account for the apparently conflicting results between the studies. Results from the early goal-directed therapy study should be reproduced and better understood. In the interim, they highlight the need for further studies in subpopulations of critically ill patients.
Alternatives to Transfusion
Decreased RBC production is one of the causes of anemia observed in the critically ill. Indeed, critical illness is characterized by blunted erythropoietin production and response.39 This blunted erythropoietin response observed in critically ill patients appears to result from inhibition of the erythropoietin gene by inflammatory mediators.40,41 It has also been shown that these same inflammatory cytokines directly inhibit RBC production by the bone marrow and may produce distinct abnormalities of iron metabolism.42,43 In patients with multiple organ failure, recombinant human erythropoietin therapy (600 units/kg) has been shown to stimulate erythropoiesis.44 Similarly, in a small randomized placebo-controlled trial (160 patients), therapy with recombinant human erythropoietin resulted in an almost 50% reduction in RBC transfusions compared to patients treated with a placebo.45 Erythropoietin was given at a dose of 300 units/kg daily for 5 days followed by every-other-day dosing until ICU discharge. Despite receiving fewer RBC transfusions, patients in the recombinant human erythropoietin group had a significantly greater increase in hematocrit.
Recently the efficacy of recombinant human erythropoietin in critically ill patients was evaluated in two similar large randomized controlled trials involving a total of 2762 patients.46,47 In the first trial, recombinant human erythropoietin was given weekly at a dose of 40,000 units. All patients received three weekly doses, and patients who remained in the ICU on study day 21 received a fourth dose. Treatment with recombinant human erythropoietin resulted in a 10% reduction in the number of patients receiving any RBC transfusions. The authors reported a 60.4% rate of transfusions following randomization in the placebo group, as compared with 50.5% in the recombinant human erythropoietin group (OR, 0.67; 95% CI, 0.54-0.83; P < .0004) and a 20% reduction in the total number of RBC units transfused in patients receiving recombinant human erythropoietin (P < .001). All clinical outcomes including mortality rates, rates of organ failure, and lengths of stay in the ICU and the hospital were comparable between groups (all P values > .05). A second large trial in 1460 critically ill patients randomly allocated patients to receive either 40,000 units/wk versus a placebo for a maximum of 3 weeks. This confirmatory trial did not find a significant decrease in mortality (OR, 0.72; 95% CI, 0.51-1.02). A systematic review incorporating results from 9 trials in 3326 critically ill patients documented a decrease in transfusions without affecting mortality rates (OR, 0.86; 95% CI, 0.71-1.05).48 These studies and the systematic review45–49 demonstrate that recombinant human erythropoietin therapy in critically ill patients can decrease in RBC transfusions and increase hemoglobin levels. This is consistent with the hypothesis that the anemia in critically ill patients is similar to the anemia of patients with chronic disease and is characterized at least in part by a relative erythropoietin deficiency.50 However, given the high costs of erythropoietin and the lack of clinical benefit demonstrated in the randomized controlled trial, its use is not recommended as a blood conservation strategy in routine practice.
Conclusions
Despite the frequent use of RBC transfusions, only three large randomized trials have examined RBC administration in postoperative and critically ill patients. Together, they consistently document that a restrictive transfusion strategy is safe and minimizes red cell use in critically ill adults and children as well as postoperative patients. However, there is insufficient evidence in early septic shock or in patients with a myocardial infarction or acute coronary syndromes. In addition, most transfusion practice guidelines published prior to completion of the TRICC trial32–34 are now dated and warrant expert opinion by solid evidence in diverse clinical settings. Still, high-quality clinical evidence is not yet available for many decisions related to RBC transfusions. In terms of alternatives, human recombinant erythropoietin should not be widely used in critically ill patients. We anticipate that risks and benefits of red cells and alternatives will be better elucidated in the coming years.
Recommendations
Key Points
1 Liano F, Garcia-Martin F, Gallego A, et al. Easy and early prognosis in acute tubular necrosis: A forward analysis of 228 cases. Nephron. 1989;51:307-313.
2 Vincent JL, Baron J-F, Reinhart K, et al. Anemia and blood transfusion in critically ill patients. JAMA. 2002;288:1499-1507.
3 Wilkerson DK, Rosen AL, Sehgal LR, et al. Limits of cardiac compensation in anemic baboons. Surgery. 1988;103:665-670.
4 Leung JM, Weiskopf RB, Feiner J, et al. Electrocardiographic ST-segment changes during acute, severe isovolemic hemodilution in humans. Anesthesiology. 2000;93:1004-1010.
5 Expert Working Group. Guidelines for red blood cell and plasma transfusion for adults and children: Report of the expert working group. Can Med Assoc J. 1997;156(Suppl 11):S1-S24.
6 Bayer WL, Coenen WM, Jenkins DC, Zucker ML. The use of blood and blood components in 1,769 patients undergoing open-heart surgery. Ann Thorac Surg. 1980;29:117-122.
7 Viele MK, Weiskopf RB. What can we learn about the need for transfusion from patients who refuse blood? The experience with Jehovah’s Witnesses. Transfusion. 1994;34:396-401.
8 Graves CL, Allen RM. Anesthesia in the presence of severe anemia. Rocky Mountain Med J. 1974;67:35-40.
9 Slawson KB. Anaesthesia for the patient in renal failure. Br J Anaesth. 1972;44:277-282.
10 Aldrete JA, Daniel W, O’Higghins JW, et al. Analysis of anesthetic-related morbidity in human recipients of renal homografts. Anesth Analg. 1971;50:321-329.
11 Samuel JR, Powell D. Renal transplantation: Anesthetic experience of 100 cases. Anaesthesia. 1970;25:165-176.
12 Gollub S, Bailey CP. Management of major surgical blood loss without transfusion. JAMA. 1966;198:149-152.
13 Carson JL, Spence RK, Poses RM, Bonavita G. Severity of anaemia and operative mortality and morbidity. Lancet. 1988;1:727-729.
14 Spence RK, Carson JA, Poses R, et al. Elective surgery without transfusion: Influence of preoperative hemoglobin level and blood loss on mortality. Am J Surg. 1990;159:320-324.
15 Ott DA, Cooley DA. Cardiovascular surgery in Jehovah’s Witnesses: Report of 542 operations without blood transfusion. JAMA. 1977;238:1256-1258.
16 Fullerton WT, Turner AG. Exchange transfusion in treatment of severe anaemia in pregnancy. Lancet. 1962;282:75-78.
17 Alexiu O, Mircea N, Balaban M, Furtunescu B. Gastro-intestinal haemorrhage from peptic ulcer: An evaluation of bloodless transfusion and early surgery. Anaesthesia. 1975;30:609-615.
18 Audet AM, Goodnough LT. Practice strategies for elective red blood cell transfusion. Ann Intern Med. 1992;116:403-406.
19 American Society of Anesthesiologists Task Force on Blood Component Therapy. Practice guidelines for blood component therapy. Anesthesiology. 1996;84:732-747.
20 Consensus Conference (National Institutes of Health). Perioperative red blood cell transfusion. JAMA. 1988;260:2700-2703.
21 Welch HG, Meehan KR, Goodnough LT. Prudent strategies for elective red blood cell transfusion. Ann Intern Med. 1992;116:393-402.
22 Cane RD. Hemoglobin: How much is enough? Crit Care Med. 1990;18:1046-1047.
23 Crosby ET. Perioperative haemotherapy: I. Indications for blood component transfusion. Can J Anesth. 1992;39:695-707.
24 Nelson AH, Fleisher LA, Rosenbaum SH. Relationship between postoperative anemia and cardiac morbidity in high-risk vascular patients in the intensive care unit. Crit Care Med. 1993;21:860-866.
25 Carson JL, Duff A, Poses RM, et al. Effects of anaemia and cardiovascular disease on surgical mortality and morbidity. Lancet. 1996;348:1055-1060.
26 Hebert PC, Wells G, Tweeddale M, et al. Does transfusion practice affect mortality in critically ill patients? Am J Respir Crit Care Med. 1997;155:1618-1623.
27 Wu WC, Rathore SS, Wang Y, et al. Blood transfusion in elderly patients with acute myocardial infarction. N Engl J Med. 2001;345:1230-1236.
28 Carson JL, Duff A, Berlin JA, et al. Perioperative blood transfusion and postoperative mortality. JAMA. 1998;279:199-205.
29 Vincent JL, Baron J-F, Reinhart K, et al. Anemia and blood transfusion in critically ill patients. JAMA. 2002;288:1499-1507.
30 Hebert PC, Fergusson DA. Red blood cell transfusions in critically ill patients. JAMA. 2002;288:1525-1526.
31 Marik PE, Corwin HL. Efficacy of red blood cell transfusion in the critically ill: a systematic review of the literature. Crit Care Med. 2008 Sep;36(9):2667-2674.
32 Carson JL, Hill S, Carless P, et al. Transfusion triggers: A systematic review of the literature. Trans Med Rev. 2002;16:187-199.
33 Hebert PC, Wells G, Blajchman MA, et al. A multicenter, randomized, controlled clinical trial of transfusion requirements in critical care. N Engl J Med. 1999;340:409-417.
34 Hebert PC, Yetisir E, Martin C, et al. Is a low transfusion threshold safe in critically ill patients with cardiovascular disease? Crit Care Med. 2001;29:227-234.
35 Bordin JO, Heddle NM, Blajchman MA. Biologic effects of leukocytes present in transfused cellular blood products. Blood. 1994;84:1703-1721.
36 Lacroix J, Hébert PC, Hutchison JS, Hume HA, Tucci M, Ducruet T, et al. TRIPICU Investigators; Canadian Critical Care Trials Group; Pediatric Acute Lung Injury and Sepsis Investigators Network. Transfusion strategies for patients in pediatric intensive care units. N Engl J Med. 2007 Apr 19;356(16):1609-1619.
37 van de Watering LM, Hermans J, Houbiers JG, et al. Beneficial effects of leukocyte depletion of transfused blood on postoperative complications in patients undergoing cardiac surgery: A randomized clinical trial. Circulation. 1998;97:562-568.
38 Rivers E, Nguyen B, Havstad MA, et al. Early goal-directed therapy in the treatment of severe sepsis and septic shock. N Engl J Med. 2001;345:1368-1377.
39 Rodriguez RM, Corwin HL, Gettinger A, et al. Nutritional deficiencies and blunted erythropoietin response as causes of the anemia of critical illness. J Crit Care. 2001;16:36-41.
40 Frede S, Fandrey J, Pagel H, et al. Erythropoietin gene expression is suppressed after lipopolysaccharide or interleukin-1 beta injections in rats. Am J Physiol. 1997;273:t-71.
41 Jelkmann W. Proinflammatory cytokines lowering erythropoietin production. J Interferon Cytokine Res. 1998;18:555-559.
42 Means RTJr, Krantz SB. Progress in understanding the pathogenesis of the anemia of chronic disease. Blood. 1992;80:1639-1647.
43 Krantz SB. Pathogenesis and treatment of the anemia of chronic disease. Am J Med Sci. 1994;307:353-359.
44 Gabriel A, Kozek S, Chiari A, et al. High-dose recombinant human erythropoietin stimulates reticulocyte production in patients with multiple organ dysfunction syndrome. J Trauma. 1998;44:361-367.
45 Corwin HL, Gettinger A, Rodriguez RM, et al. Efficacy of recombinant human erythropoietin in the critically ill patient: A randomized, double-blind, placebo-controlled trial. Crit Care Med. 1999;27:2346-2350.
46 Corwin HL, Gettinger A, Pearl RG, et al. Efficacy of recombinant human erythropoietin in critically ill patients: A randomized controlled trial. JAMA. 2002;288:2827-2835.
47 Corwin HL, Gettinger A, Fabian TC, May A, Pearl RG, Heard S, et al. EPO Critical Care Trials Group. Efficacy and safety of epoetin alfa in critically ill patients. N Engl J Med. 2007 Sep 6;357(10):965-976.
48 Zarychanski R, Turgeon AF, McIntyre L, Fergusson DA. Erythropoietin-receptor agonists in critically ill patients: a meta-analysis of randomized controlled trials. CMAJ. 2007 Sep 25;177(7):725-734.
49 Silver MJ, Bazzan A, Corwin H, et al. A randomized double blind placebo controlled trial of recombinant human erythropoietin in long term acute care patients. Crit Care Med. 2003;31:A167.
50 Corwin HL, Krantz SB. Anemia of the critically ill: “Acute” anemia of chronic disease. Crit Care Med. 2000;28:3098-3099.