CHAPTER 130 Anatomy of the Vestibular System
The semicircular canals are arranged as a set of three orthogonal sensors (Fig. 130-1)—that is, each canal is at approximately right angles to the other two. Each canal is maximally sensitive to rotations that lie in the plane of that canal. The result of this arrangement is that the three canals can uniquely specify the direction and amplitude of any arbitrary head rotation. Each of the canals acts as an integrating accelerometer; the necessary stimulus for the canal is an angular acceleration, but the information that is encoded by the firing of the afferent nerve fiber is more closely related to angular velocity. Finally, the canals are organized into functional pairs wherein both members of the pair lie in the same plane. Any rotation in that plane is excitatory to one of the members of the pair and inhibitory to the other. Although in the horizontal system the two horizontal canals form a functional pair, the situation is more complex in the vertical system. Here, the anterior canal on one side is parallel and coplanar with the posterior canal on the opposite side; for example, the right anterior canal and the left posterior canal form a functional pair.
The vestibular system forms the basis for many fundamental reflexes: the vestibulocollic reflex (head stabilization), vestibulospinal reflex (control of upright posture) and vestibuloocular reflex (retinal image stabilization). The last of these, the vestibuloocular reflex, has been studied far more extensively than the others and is the best understood; this reflex forms the basis for most clinical testing (e.g., calorics, rotation tests). See also Chapters 163 and 164 for clinical applications.
Embryology of the Vestibular Apparatus
The development of the inner ear is a complex process that starts at the beginning of the 4th week and is completed at about 25 weeks of gestational age. By that time, the vestibular apparatus has achieved adult form and size. A brief description of this process of development in human embryos follows. Further details can be found by consulting more detailed references.1–3 An excellent description of the molecular aspects of development can be found in Post,4 and a review by Kelley5 discusses these molecules in relation to potential hair cell regeneration (see also Chapters 1 and 2).
When the human embryo reaches the seven-somite stage (about 22 days), surface ectoderm overlying the future site of the inner ear (at about the level of the first occipital somite) thickens to form the otic placode. The otic placode invaginates into the mesenchyme, forming an otic pit. At about 30 days, the otic pit becomes pinched off, forming the otic vesicle or otocyst (see Fig. 130-1). Concurrently at about 4 weeks, a portion of the neural crest migrates to the vicinity of the otic vesicle and becomes the acousticofacial ganglion. The geniculate ganglion soon migrates away from this cluster of neurons, leaving the vestibulocochlear ganglion in close proximity to the otic vesicle.
The saccular chamber differentiates by expansion and coiling of the cochlear duct. This duct becomes separated from the sacculus by a narrowing of the duct at its dorsal end to form the ductus reuniens. While morphogenesis proceeds within the otocyst, histogenesis of the sensory epithelium is also occurring. The arrival of afferent endings in the epithelium precedes hair cell differentiation.6,7 In the 3rd week, a common macula, or specialized neuroepithelium, appears. Its upper part becomes the utricular macula and crista ampullaris of the superior and lateral semicircular ducts, and its lower part becomes the saccular macula and crista ampullaris of the posterior semicircular duct. At 9 weeks, the hair cells in the vestibular end organs are well differentiated, and they exhibit typical synapses with nerve endings. The maculae reach adult form at about 14 to 16 weeks; the cristae, at about 23 weeks; and the organ of Corti, at about 25 weeks. The mesoderm surrounding the membranous labyrinth becomes the bony otic capsule, or bony labyrinth. The membranous labyrinth is suspended in fluid (perilymph) within the bony labyrinth by a loose connective tissue termed periotic tissue.
Overall Organization of Labyrinth: Relationship to Skull and Cochlea
The vestibular apparatus is enclosed within a bony labyrinth, the vestibule, in the petrous portion of the temporal bone. The vestibular end organs include three semicircular canals, each oriented in a different plane, and two maculae, one roughly in the horizontal plane (the utriculus) and one in the vertical plane (the sacculus). There are two vertical semicircular canals, the anterior (also known as the superior) and posterior canals, and one horizontal (also known as the lateral) canal. The vertical canals are oriented roughly at 45 degrees in relation to the sagittal plane, and the horizontal canal is tilted upward about 30 degrees anteriorly from the horizontal plane (Fig. 130-2). The five vestibular end organs, along with the cochlea, are contained within an endolymph-filled membranous labyrinth (the endolymphatic space), which is itself contained in the perilymph-filled bony labyrinth (the perilymphatic space) (Fig. 130-3).
The vestibule is situated between the internal auditory meatus anteromedially and the middle ear cavity laterally (Fig. 130-4). The entrance to the mastoid antrum (the aditus ad antrum) is just lateral to the horizontal semicircular canal. The cochlea sits anterior to the vestibule and is connected to the vestibule by the narrow ductus reuniens (Fig. 130-5; see Fig. 130-3). Posterior and lateral to the vestibule are the mastoid air cells. Directly medial is the posterior cranial fossa, into which the endolymphatic duct and sac extend beneath the dura.
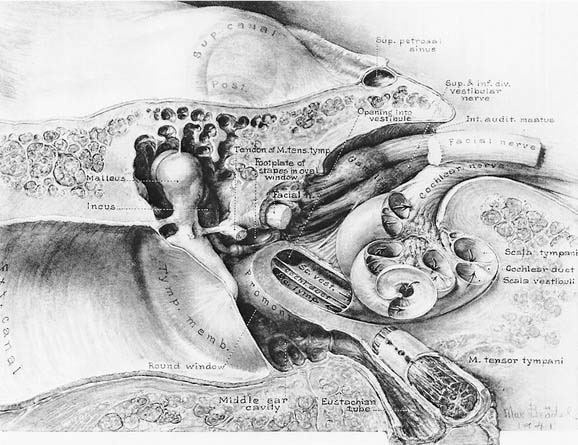
Figure 130-4. Course of vestibulocochlear nerve within the auditory meatus.
(From Brodel M. Three Unpublished Drawings of the Anatomy of the Human Ear. Philadelphia: Saunders; 1946.)
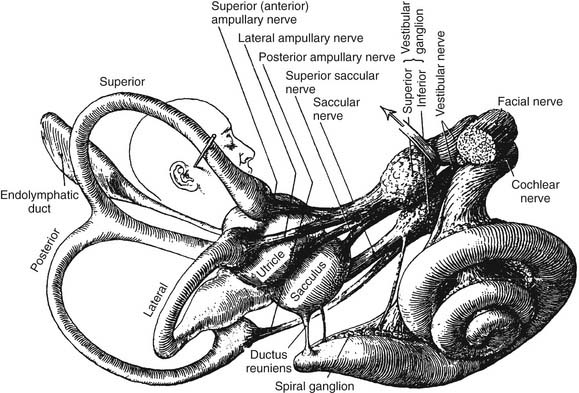
Figure 130-5. Distribution of vestibular nerve to end organs.
(From Brodel M. Three Unpublished Drawings of the Anatomy of the Human Ear. Philadelphia: Saunders; 1946.)
The seventh (facial and intermediate) and eighth (vestibulocochlear) cranial nerves emerge from the brainstem laterally at the cerebellopontine angle. They enter the vestibule and cochlea from the internal auditory meatus (see Fig. 130-4), located medial to a point midway between the cochlea and vestibule. The facial nerve lies anterior and dorsal to the vestibulocochlear nerve. The two nerves separate just inside the meatus, and the facial and intermediate nerves continue laterally in their own canal, past the vestibule. When past the vestibule, the facial nerve makes a 90-degree turn inferiorly to exit the temporal bone through the stylomastoid foramen. The vestibulocochlear nerve splits into a vestibular division, which turns posteriorly to supply the vestibule, and a cochlear division, which turns anteriorly to supply the cochlea.
The vestibular (Scarpa’s) ganglion sits at the bottom of the internal auditory meatus. It has two parts, the superior vestibular ganglion and the inferior vestibular ganglion (see Fig. 130-5). Large ganglion cells in the superior and inferior ganglia provide afferent innervation to the central regions of the cristae and maculae, and small ganglion cells innervate the peripheral regions of these end organs (the regional specialization within the end organs is discussed in more detail subsequently). A nerve branch is associated with each ganglion. The superior (or anterior) vestibular nerve supplies the anterior and horizontal cristae and the utricular macula. The inferior (or posterior) vestibular nerve supplies the posterior canal and saccular macula. There are also a few small branches that anastomose between the larger divisions of CN VIII. One such small branch is Voit’s anastomosis, which runs from the superior vestibular nerve to the anterosuperior part of the sacculus. Another is the vestibulocochlear (Oort’s) anastomosis, which runs from the inferior vestibular nerve to the cochlear nerve and carries cochlear efferents (see later). In addition, some intermediate nerve fibers cross to the vestibular nerve proximal to the superior vestibular ganglion, and others cross back to the intermediate nerve distal to Scarpa’s ganglion. It has been suggested that this facial-vestibular anastomosis carries parasympathetic innervation to the vestibular labyrinth; for details on these and other small fiber connections, see Lindemann.8
Efferent Innervation of Peripheral Vestibular Apparatus
The efferent supply to the vestibular end organs arises from a small group of about 200 neurons lateral to the abducens nucleus and the genu of the facial nerve, the so-called group e (see later).9 These neurons project ipsilaterally and contralaterally (Fig. 130-6). The contralateral pathway crosses the midline at the level of the facial genu and joins the ipsilateral pathway; both pass ventral to the vestibular nucleus. At this point, they are joined by cochlear efferents originating from the superior olivary complex (olivocochlear bundle). All of the efferents enter the vestibular nerve, coursing through the middle of the nerve in a small distinct bundle. At the end organs, these few fibers branch profusely to innervate the entire sensory epithelium. More recent work suggests that the ipsilaterally projecting efferents supply the central regions of the crista, whereas the contralaterally projecting efferents supply the peripheral zone.10 The efferent fibers terminate as highly vesiculated boutons, making synaptic contacts with hair cells and afferent fibers.11,12 For an excellent review of the neurochemistry of the vestibular efferent system, see Goldberg and colleagues.13
Autonomic Supply of Peripheral Vestibular Apparatus
Postganglionic sympathetic fibers from neurons in the superior cervical ganglion also innervate the vestibular end organs. They are of two types: perivascular and nonvascular. The nonvascular sympathetic fibers run among the myelinated afferent fibers. The terminals of these nonvascular sympathetic fibers are found as free endings near the cells of Scarpa’s ganglion, distal to the ganglion and underneath the sensory epithelium. They do not seem to penetrate the basement membrane into the sensory epithelium and do not seem to have a direct effect on the hair cells or afferent fibers.14 Their functional role remains unexplored, along with the functional role of any parasympathetic innervation of the vestibular sensory epithelium.
Blood Supply to Vestibular End Organs
The main blood supply to the vestibular end organs is through the internal auditory (labyrinthine) artery, which arises most often (45%) from the anterior cerebellar artery, superior cerebellar artery, or basilar artery, according to a radiographic study by Wende and coworkers.15 Shortly after entering the inner ear, the labyrinthine artery divides into two branches: the anterior vestibular artery and the common cochlear artery (Fig. 130-7). The anterior vestibular artery provides the blood supply to most of the utriculus and the superior and horizontal ampullae, and some blood to a small portion of the sacculus. The common cochlear artery forms two divisions, the proper cochlear (or spiral modiolar) artery and the vestibulocochlear artery. The latter divides into a cochlear ramus and a vestibular ramus, also known as the posterior vestibular artery. The posterior vestibular artery is the source of the blood supply to the posterior ampulla, the major part of the sacculus, and parts of the body of the utriculus and horizontal and superior ampullae.
Anatomy of End Organs
At the dilated end of each semicircular duct is the ampulla. It contains the neuroepithelium (crista ampullaris), the cupula, supporting cells, connective tissue, blood vessels, and nerve fibers. The crista is a saddle-shaped, raised section of the wall that extends across the floor of the ampulla at right angles to its long axis. The crista has been found to be divided into central (near the apex) and peripheral (on the slope) zones based on the morphology and physiology of vestibular afferents supplying the different regions (see later).16,17 The shape of the crista facilitates maximal packing of the specialized mechanoreceptor hair cells.
The hair cells and the supporting cells are modified columnar epithelial cells that have microvilli on their apical surfaces. In the hair cells, many of these microvilli are elongated to form stereocilia, which are grouped in an organ pipe–like arrangement (Fig. 130-8). In addition, each hair cell has a single long kinocilium, a true cilium showing the 9 + 2 arrangement of microtubules. This kinocilium is longer than the stereocilia and eccentrically located, imparting a certain polarization to the hair cell that has important functional implications (see Chapter 163). In the cristae, the kinocilium on each hair cell is located on one end of the cell (Fig. 130-9). In the horizontal cristae, the kinocilia are located on the side of the hair cell that is closest to the utriculus. In the vertical cristae, the kinocilia are located on the side of the hair cell furthest from the utriculus, the canalicular side. The entire hair bundle extends upward into the cupula (Fig. 130-10).
The cupula is a gelatinous mass, consisting of mucopolysaccharides within a keratin meshwork,18 which extends from the surface of the cristae to the roof and lateral walls of the membranous labyrinth, forming a fluid-tight partition. There is a distinct subcupular space in the region of the cupula overlying the apex of the center of the crista (see Fig. 130-10). This subcupular space is believed to provide space for freedom of movement and more sensitive responses to endolymph flow for the stereocilia on the hair cells in the central zone. The space may be artifactual and due to fixation methods commonly used to prepare tissue for histology. The specific gravity of the cupula is approximately 1, which is about the same as that of the endolymph.19 This matching of the specific gravity of the cupula and the endolymph is necessary to prevent the cupula from floating upward in certain head positions and causing an enduring nystagmus. Disruption of this match in specific gravity is likely the cause of postalcoholic nystagmus.
A torsion pendulum model is used to describe the mechanics of cupular and endolymph displacement.20 For a description of the mathematic details of the response of canal afferents, see Goldberg and Fernández.21 More details are also given in Chapter 163.
Similar to the cristae ampullares, the maculae of the utriculus and sacculus consist of neuroepithelium, supporting cells, blood vessels, and nerve fibers. The utricular macula is oriented in the horizontal plane, and the saccular macula is oriented in the vertical plane (Fig. 130-11). Cilia from the hair cells in both maculae extend upward into their respective otolithic membranes (Fig. 130-12)—gelatinous membranes analogous to the cupula. In the upper surface of the otolithic membranes, the otoliths (or otoconia) are embedded. Otoliths are inorganic crystalline deposits composed of calcium carbonate or calcite.22 They vary in size from 0.5 to 30 µm, with most being about 5 to 7 µm.8 The specific gravity of the otolithic membrane is much higher than that of the endolymph, about 2.71 to 2.94.19 Within the otolithic membrane is the striola,23 a specialized central region that has a snowdrift-like appearance. The striola is identifiable as a thin stripe running down the center of the otolith membranes of both maculae. In the striola, the otoliths are very small (about 1 µm), and the thickness of the otolithic membranes is either reduced, as in the utricular macula, or increased, as in the saccular macula. These regional differences in the otolithic membranes are paralleled by morphologic and physiologic differences in the afferent fibers supplying the hair cells in the underlying sensory epithelium.24–26
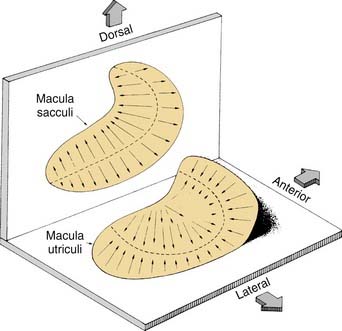
Figure 130-11. Orientation of two maculae. Utricular macula is in horizontal plane, and saccular macula is in vertical plane.
(From Barber HO, Stockwell CW. Manual of Electronystagmography. St. Louis: Mosby-Year Book; 1976.)
The kinocilia on the hair cells in the maculae are also dynamically polarized, but the pattern of polarization is much more complex than in the cristae (see Figs. 130-9 and 130-11). In the utricular macula, the kinocilia are oriented so that they point toward the striola, whereas in the saccular macula, the kinocilia point away from the striola. Because both maculae are curved areas, and the striolae are curved lines, the arrangement is so complex (see Fig. 130-11) that static head tilts in any direction cause some hair cells to be excited and others to be inhibited in one or both of the otolithic organs. The stimulus is encoded by means of stimulation of hair cells in the appropriate sector of the macula.
The otolith organs are sensitive not only to gravity, but also to other linear acceleration forces, such as forward motion and bobbing movements of the head during walking. Static head tilts are represented by a vector and the afferent response predicted by a trigonometric function based on the tilt angle. One would expect a linear model based on this simple relationship; however, there is a response asymmetry in that the excitatory response is larger than the inhibitory response. Consequently, the equation describing this force-response relationship is by necessity nonlinear (for more mathematical details, see Fernández and Goldberg27,28).