CHAPTER 1 Anatomy
Introduction
Dissection anatomy, indeed, had its fullest expression from the late eighteenth to the early twentieth century (Mascagni, Gray, Sobotha, Testut, etc.) when all the aspects of dissection anatomy where definitively studied (Fig. 1.1). In the past 50 years anatomical dissection has been little used to investigate venous anatomy, probably because of the assumption that there is nothing new to discover (but also because it is more and more difficult to find cadavers for this purpose). Meanwhile, most anatomical, clinical and surgical textbooks describe the superficial veins of the lower limb as a simple ‘tree’ formed by a few constant and recognizable veins, though clinical experience often shows anomalies and variations with respect to the classical anatomical description or even the complete absence of some of these veins. Furthermore, usually studies in the field of limb veins concern subjects with varicose pathology and rarely subjects with a normal venous system.
Confirming this, the official Anatomical Terminology (Nomina Anatomica)1 includes only a limited number of veins and does not take into account their numerous variations. Inadequacy of official anatomy has caused many authors to name single veins independently or even after the author’s name, which, in the absence of an accepted interpretation frame has added some confusion. The nomenclature consensus statement of 2001 at the Rome UIP World Congress was organized with the purpose of solving this problem (see Table 1.1).2
Table 1.1 Summary of important changes in nomenclature of lower extremity veins
Old Terminology | New Terminology |
---|---|
Femoral vein | Common femoral vein |
Superficial femoral vein | Femoral vein |
Sural veins | Sural veins Soleal veins Gastrocnemius veins (medial and lateral) |
Hunterian perforator | Midthigh perforator |
Cockett’s perforators | Paratibial perforator Posterior tibial perforators |
May’s perforator | Ankle lateral and medial perforators |
Gastrocnemius point | Intergemellar perforator |
Modified from Sherman RS: Ann Surg 130:218, 1949.
Ultrasound imaging makes it easy to observe the veins of the lower limb, unlike anatomical dissection and phlebography. Examination is non-invasive, repeatable and relatively low in cost. Veins can be observed at full distension, with the patient in a standing position, so that, unlike with anatomical dissection, their real volumetric relationship with the surrounding tissue is readily appreciated. Ultrasound images show not only the veins (as contrast phlebography does), but their relation to surrounding anatomical structures, in particular muscle and fascial layers. This allows precise anatomical identification of the observed veins (Fig. 1.2). Therefore, USI is a unique tool for the study of vein anatomy (US dissection) and makes it possible to verify data obtained from anatomical dissections. In addition, DS allows the detection of blood flow in the observed veins with assessment of their function and involvement in venous pathology. Interestingly, USI was first employed for the clinical identification of pathologically changed veins. Later it was used for collecting data on normal vein anatomy.3
Nomenclature
Nomenclature used throughout the textbook conforms to that developed at the Venous Consensus Conference Classification in 1994.4 In addition, the newest revisions of nomenclature and definitions are used, which were developed at the Nomenclature Congress in Rome in 2001 (Table 1.1).2,5 The long saphenous vein is referred to by the English-Latin term great (GSV). The short saphenous vein is referred to using the English-Latin translation small (SSV), avoiding the term ‘lesser’ as the L could be confused with the term ‘long’. Veins that ‘perforate’ the fascia are termed perforator veins. Veins that connect to other veins within a fascial plane are referred to as communicating veins. The principal deep vein of the thigh is termed the superficial femoral vein, now properly called the femoral vein. The superficial femoral vein actually has turned out to be a potentially lethal misnomer. It has been found that the use of this term is hazardous to patients suspected of having deep venous thrombosis. Many primary care physicians have not been taught and are not aware of the fact that the superficial femoral vein is actually a deep vein of the thigh and that acute thrombosis in this vessel is potentially life threatening.6
General Considerations
The veins of the lower limbs are traditionally described as consisting of two systems: one within the muscular compartment and its fascia, the deep system, and one superficial to the deep fascia, the superficial compartment (Fig. 1.3).
The lower limb deep venous system is found inside the muscles within the muscular fascia. This allows it to feel the effects of the tonus variations during contraction–relaxation, being the only structure able to vary its volume.7 The superficial veins are in an extrafascial position with respect to the muscles, although the most important (i.e. saphenous veins) are found with superficial fascia duplication.
The lower legs’ deep venous system cannot be seen as an independent entity, separated from the superficial veins. The venous function’s first purpose is to organize the anti-gravity blood backflow to the right heart, taking advantage of it’s volume capacity (three times as much as in arteries), it’s low pressure and it’s compliance, so that the reservoir (the interstitium, depending on lymphatics) may not be involved.7 Other primary important functions, although more localized, are tissue drainage and thermoregulation. These three functions are assured in all different body positions and activity, otherwise ‘venous insufficiency’ occurs.8
Tissue drainage and the maintenance of volume flow are based on valvular and, more importantly, muscle function. Both systems strictly integrate with the venous reservoir function, the respiratory function and the filling ‘vis-a-tergo’ due to the capillary network.7
Venous backflow represents about 10% of the total flow at rest, but increases heavily during dynamic conditions due to the physiologic alternate contraction–relaxation of the flexor–extensor muscles. These act as a peristaltic pump and as a dynamic reservoir, conditioning either the squeezing (contraction) or the distension (relaxation) of the deep veins (with action on the venous sole of the foot and, above all, ankle joint movement of particular amplitude conditioning the most important calf pump (Fig. 1.4).
Deep vein communications, mutual or with superficial veins, are extremely frequent so that the postural and the rest phases may address the venous backflow through less resistant pathways, typically the deep veins in the physiologic situation.7
Deep Venous System
The structure of the deep venous system is shown in Figure 1.5.9 There are at least two deep veins for each of the three arteries (anterior and posterior tibial arteries and peroneal artery), mutually communicating by transverse bridges (like a ladder). The extremely rich muscular plexus (also connected to the superficial veins) drains into these axial veins placed parallel to arteries. At the foot, axial veins are prevalent in the plantar region, where the first pump mechanism is present (Léjars sole) (Fig. 1.6).10–12
At the soleus and gastrocnemius sites the veins are even larger in number and arranged in a spiral shape, due to the longitudinal excursion amplitude of the muscles between contraction and relaxation. This creates a volume reservoir (pump chamber), and the relative muscles (soleus and gastrocnemius) are responsible for both movement/standing position as well as pump function (the second and most important pump). This system is correctly termed the calf muscle pump or peripheral heart (see Fig. 1.4).12
In contrast, posterior deep compartment veins (posterior tibial and peroneal) and anteroexternal compartment veins (anterior tibial) are rectilinear, as the surrounding muscles lean against the bones and have a limited shortening during contraction.7
At the knee and thigh, deep leg veins flow into the collecting system (popliteal-femoral veins). They run in the popliteal crease and adductors canal, and are not enwrapped by a muscular layer as the blood flow to the abdominal cavity has not been held back by compression.7 The other thigh veins (profunda femoris and circumflex) are still deep intramuscular veins. The popliteal vein is also connected by anonymous muscular veins to the profunda femoris and the sciatic nerve vein, creating a natural bypass when obstruction occurs to the femoral vein (thrombosis, extrinsic compression, bone fracture).13 Thanks to this autonomy, the femoral vein is used as an alternative conduit when other more accessible superficial veins are unavailable (see Fig. 1.5).9
The common femoral vein collects the backflow of the lower limb and sends it to the pelvis (iliac veins and inferior vena cava), where aspiration pleurodiaphragmatic forces prevail, together with vis-a-tergo of the renal veins. The common femoral vein in particular receives the GSV below the inguinal ligament where it becomes the external iliac vein. A potential alternative way of discharge in this area is due to the obturator vein (normally draining part of the muscles of the medial thigh) and the sciatic vein, often not macroscopically evident (first embryonic vein, secondarily replaced by the femoropopliteal axis, which can be activated in certain conditions). Together with the superficial veins they can contribute to limb drainage in case of femoral thrombosis by their connection to the hypogastric vein (see Fig. 1.5). However, the same system may be the cause of varices when endopelvic hypertension is transmitted to the superficial limb veins. The sciatic vein may also be involved in congenital venous malformations, typically Klippel-Trenaunay syndrome.13
Anatomy of the Superficial Veins
The most important superficial veins are the GSV and the SSV. It is generally thought that the term saphenous is derived from the Greek word saphenes, meaning evident, but it could also come from the Arabic words el safin, which mean hidden or concealed.14 Of course, these terms were important in the practice of blood letting.
Great saphenous vein
This vein begins on the dorsum of the foot as a dorsal venous arch and internal marginal vein. It passes anterior (10–15 mm) to the medial malleolus, crosses the tibia at the distal third and runs along the tibial internal edge. At the knee the vein it bends posteriorly, running around the condilus femoralis, in contact with the anterior edge of the sartorius muscle, then ascends in the anteromedial thigh, crosses the sartorius and adductor brevis and enters the Scarpa triangle to empty into the common femoral vein (Fig. 1.7).9,15 This termination point is referred to as the saphenofemoral junction (SFJ) but is also known as the crosse, which is the French description for its appearance as a shepherd’s crook. The average diameter of a normal GSV is 3.5–4.5 mm (range 1–7 mm).16
Two large tributaries in the upper third of the thigh – the posteromedial and anterolateral tributaries – join the GSV proximally. These veins usually enter the GSV before it dives posteriorly to penetrate the deep fascia at the fossa ovalis. Both the medial and lateral superficial thigh veins may be so large that they are mistaken for the GSV itself.17 A variable number of perforators connect the GSV to the femoral, posterior tibial, gastrocnemius and soleal veins.18
Small saphenous vein
The SSV is the most prominent and physiologically important superficial vein below the knee (Fig. 1.8).9 Like the GSV, the SSV has a thick wall and usually measures 3 mm in diameter when normal.19 It begins at the lateral aspect of the foot and ascends posterior to the lateral malleolus as a continuation of the dorsal venous arch. It continues up the calf between the gastrocnemius heads to the popliteal fossa, where it usually enters the popliteal vein.
The termination of the SSV is quite variable, usually occurring in the popliteal vein, as stated above. However, in 27% to 33% of the population, it terminates above the level of the popliteal fossae, either directly into the GSV or into other deep veins. In 15.3% of patients, the SSV communicates with the popliteal vein, then continues terminating in the GSV. In 9% to 10%, the SSV empties into the GSV or the deep veins below the popliteal fossae.9,20 The SSV may also join the GSV in the thigh through an oblique epifascial vein (the Giacomini vein), or it may continue up under the membranous fascia of the thigh as the femoropopliteal vein, joining the deep veins in the thigh at various locations (Fig. 1.9).21–23
Like the GSV, the SSV runs on or within the deep fascia, usually piercing the deep fascia just below the flexor crease of the knee as it passes into the popliteal fossa.24 Gross incompetence of the SSV usually occurs only in areas where the SSV and its tributaries are superficial to the deep fascia, on the lateral calf and lower third of the leg behind the lateral malleolus. The SSV often receives substantial tributaries from the medial aspect of the ankle, thereby communicating with the medial ankle perforators. The SSV may also receive a lateral arch vein that courses along the lateral calf to terminate in the SSV distal to the popliteal fossa. It may also connect directly with the GSV.
Other superficial veins and collateral veins
The superficial collateral or communicating venous network consists of many longitudinally, transversely and obliquely oriented veins. These originate in the superficial dermis, where they drain cuticular venules. These veins are normally of lesser diameter, but when varicose they can dilate to more than 1 cm. They are thin walled and are more superficial than the superficial fascia that covers the saphenous trunks. They drain into deep veins through the saphenous veins, directly through perforating veins or through anastomotic veins in the abdominal, perineal and gluteal areas.25 Therefore, collateral veins may become varicose either in combination with truncal varicose veins or independently (Fig. 1.10).15
Although many collateral veins are unnamed, some prominent or consistent superficial veins are, for example, the Giacomini vein, which connects the proximal GSV to the SSV. This vein has been found by duplex examination in 70% of limbs with chronic venous insufficiency.26 Other examples include the lateral anterior accessory saphenous vein (AASV), which runs from the lateral knee to the SFJ, the anterior crural veins, which run from the lower lateral calf to the medial knee, and the infragenicular vein, which drains the skin around the knee. Geniculate perforators, although small, may contribute significant reflux (see Figs 1.7, 1.8).
A lateral subdermal plexus of reticular veins, first described by Albanese et al,27 has its origin through perforating veins at the lateral epicondyle of the knee (Fig. 1.11). It has been speculated that it represents a remnant of the embryonic superficial venous system that fails to involute. This system of veins has its importance in the development of telangiectasia. These veins may become varicose even in the absence of truncal varicosities.
Duplex ultrasound anatomy
The most striking progress in the knowledge of venous anatomy for phlebologists is related to the easy visibility of the fascial sheets by DUS imaging. This DUS anatomical ‘dissection’ has offered the key for interpretation of these variations providing a simple universal language for the easy identification of veins (Fig. 1.12).28,29
The result is that leg veins are not just ‘deep’ or ‘superficial’, but are arranged in three levels: deep (beneath the aponeurotic fascia), intermediate (between the aponeurotic fascia and the superficial fascia) and subcutaneous (between the superficial fascia and the skin) (Fig. 1.13).8,28
In the early nineteenth century two French anatomists, Cruveilhier30 and Bayle,31 described for the first time that both saphenous veins lie in the deeper compartment of the subcutaneous space and are covered, for their entire length, by the superficial fascia. All other superficial veins (tributaries or collaterals of the saphenous) run into the superficial compartment, between the superficial fascia and the skin, in what is a true subcutaneous position. Despite evidence from anatomical dissection (Fig. 1.14),32 the importance of the superficial fascia as an anatomical classification marker had been largely ignored until DUS became an established tool for venous investigation of leg vein anatomy.29
It was proposed to name the interfascial compartment in which the GSV runs the ‘saphenous compartment’, and the superficial fascia that covers it, ‘saphenous fascia’ (Fig. 1.15).28 The superficial fascia is a marker for distinguishing the two levels of superficial veins. A few constant, and named, superficial veins run through specific intrafascial compartments (intermediated veins), covered by fascial sheet, and belong to the intermediate level. These intrafascial veins are (Fig. 1.16):3,33
These veins are longitudinal ‘blood transfer’ vessels of major importance in understanding varicose hemodynamics. Their position inside the close fibroelastic ensheathing and adventitial anchoring may explain the absence of varicosity in these veins (they enlarge but do not become varicose).34 A pump mechanism during muscular contraction can also be another explanation with caliber reduction due to the fascial compression effect enhancing blood flow8 (Fig. 1.17); similar, but less efficient to what happens in the deep compartment.
Every vein running superficially to the fascial sheet should be considered a collateral or tributary vein (Fig. 1.18). Its identification is consequently of paramount importance when treatment must be provided in a varicose condition. Varicose veins typically belong to this superficial layer.3,8
With a thorough understanding of this scheme, all possible venous anatomical variations may be correctly understood.35
Duplex Ultrasound Markers for Vein Identification
The veins of the intermediate level have constant relationships with the surrounding anatomical structures – fascial sheets, muscles, bones, deep vessels – which are easily recognized by DUS and are therefore ultrasound ‘markers’ for vein identification.3,33 It is from these markers that the following ultrasound identification signs derive.
The ‘eye’ sign
Bailly first described, in 1993, the ‘eye’ sign as the ultrasound marker for identification of the GSV in the thigh.29 This sign is due to the fact that the superficial fascia is echo-lucent and easily observed by USI. In transverse scan the compartment in which the GSV runs resembles an Egyptian eye, where the saphenous lumen is the iris, the superficial fasci, the superior eyelid and the aponeurotic fascia the inferior eyelid (see Fig. 1.15). The description of the ‘saphenous eye’ could well be considered the beginning of ultrasound vein anatomy. The eye sign is always present and allows immediate and certain identification of the saphenous vein and its separation from parallel running subcutaneous collaterals.
The ‘alignment’ sign
This sign, also suggested by Bailly,37 helps recognize and distinguish the AASV from the GSV.36,38 In the upper third of the thigh on transverse scan into the ‘eye’ there are often two veins instead of one: the GSV and the AASV. The latter lies anterior (lateral) to the GSV39–41 and is identified by its subfascial position and by the fact that in transverse scan it lies over (is aligned with) the common femoral vessels (artery and vein) (Fig. 1.19A). In addition to the alignment sign, in some cases the AASV has, in transverse scan, its own ‘eye’8 (Fig. 1.19B). However, it is the alignment sign that shows that in some cases the only vein visible in the ‘eye’ is the ASV, while the GSV is not visible (absent or hypoplastic) (Fig. 1.19C).38
The tibia-gastrocnemius angle sign
This sign allows one to recognize the GSV below the knee, where fascial sheets are often so close to each other that the intrafascial compartment in which the GSV runs may be difficult to recognize.36,42 In such cases the GSV is distinguished from other closely running veins by its position, on a transverse scan, in the angle formed by tibial and medial gastrocnemius muscle (Fig. 1.20A, B). This sign allows one to demonstrate, when the angle is empty, that in this area the GSV is absent or hypoplastic (Fig. 1.20C).
The small saphenous compartment sign
The proximal portion of the SSV lies between the medial and lateral heads of gastrocnemius muscle, while its frequent thigh extension (TE) lies between the semitendinous muscle (medially) and long head of the biceps muscle (laterally). The intermuscular grooves, along which these two veins run, are covered by a thick fascial sheet and appears as a characteristic triangle-shaped compartment on a transverse scan (Fig. 1.21A, B).43,44 This triangle-shaped compartment is always present and allows immediate and certain identification of the SSV/TE and distinguishes it from parallel subcutaneous and deep collaterals. Distal to the gastrocnemius muscle the fascial sheet is still present (Fig. 1.21C), albeit less evident as it is thinner as it approaches the ankle and the marginal vein over the foot indicating that it is the SSV. As for the GSV, it courses inside a specific compartment for its entire length.3
Relationship between saphenous veins and collaterals
The GSV is often accompanied by parallel veins of different lengths. They can be so large that they can be wrongly confused with the GSV itself or ‘double’ or duplicate saphenous veins. In fact these parallel veins are collaterals that pierce the superficial fascia to get out of the saphenous compartment and run subcutaneously at a more superficial level than the GSV (Fig. 1.22).3,28,35 The relationship between the saphenous trunk and these subcutaneous collaterals could be schematized into three anatomical patterns with specific ultrasound appearance45 (Fig. 1.23):
Great Saphenous Vein
The GSV begins anterior to the medial malleolus as the continuation of the medial marginal foot vein and then ascends along the medial aspect of the tibia and thigh to empty into the common femoral vein in the groin. The GSV lies for its entire length (Fig. 1.24) in a compartment delimited by the aponeurotic and the superficial (saphenous) fascia. On transverse scan this compartment appears as an ‘eye’ (see Fig. 1.15).3,28,29,33,35,36 This ‘eye’ is readily visible in the thigh, but may be difficult to recognize in very thin subjects, and in some areas such as the knee and ankle.42
Saphenofemoral junction
The GSV has a constant (terminal or ostial) valve, which is usually well visible at its junction (although separated by 1–2 mm from the ostium) with the femoral vein. Another valve (preterminal valve) can be found about 2 cm distal to it, at the distal border of the SFJ area. Between the two valves the GSV is joined by constant tributaries divided into proximal and distal (Fig. 1.25).3,46
The proximal collaterals are, from lateral to medial: the superficial iliac vein, superficial epigastric vein and superficial pudendal vein. They may have different individual anatomical arrangements. They drain venous blood from the abdominal wall and pudendal areas. Their clinical importance is relevant when they feed a retrograde flow of the GSV in the presence of a competent terminal valve. This situation has been reported in 28% to 52% of cases of GSV reflux47 (Fig. 1.26) and may preclude the need for direct GSV treatment in many cases.
The superficial external pudendal artery (immediately identified by color duplex) is intimately associated with the GSV at the SFJ, where it may bifurcate to enclose the GSV (Fig. 1.27).
The distal collaterals, lateral and medial, may be relatively large. The lateral collateral – the AASV – is present in 40% of subjects as a well-distinguished vein (see Fig. 1.19B). In most cases the AASV joins the GSV within 1 cm of the SFJ, and there is typically a lymph node in the angle between the GSV and the AASV before they merge (see Fig. 1.19A).
The medial collateral joins the GSV at a variable distance from the SFJ, often distal to the preterminal valve. The medial collateral may be the continuation of a large vein coming through the posterior thigh from the SSV as the Giacomini vein. The lymph node that is consistently found between the GSV and the AASV merger may have a large and incompetent central vein, sometimes becoming a source of reflux into the thigh and leg varicose veins.47
Arrangement of the GSV and its subcutaneous collaterals in the thigh
Based on the ‘eye’ sign, the following anatomical patterns were observed in 610 consecutive limbs with and without varicose veins3 (Fig. 1.28):
Arrangement of the GSV and its subcutaneous collaterals at the knee
At the knee the vein anatomy is sometimes difficult to assess because of the presence of multiple collaterals and perforators clustered into a limited space.42 In addition, the superficial fascia creating the saphenic eye may be difficult to recognize. However, the GSV can still be identified by its position in the angle formed by the tibia bone and gastrocnemius muscle (T-G angle)29 (see Fig. 1.20A,B,C).
On transverse scan, and based on this sign, in a series of 500 consecutive limbs with and without varicose veins, the arrangement of the GSV and the collateral veins (CVs) along its middle portion (between the distal third of the thigh and the proximal third of the leg) presented the following patterns (Fig. 1.29):
These data show that in one-third of subjects the middle portion of the GSV is absent (or hypoplastic) for a variable length (types D and E). In subjects with this pattern, varicose veins were present in 56% of cases, while in subjects where the GSV is present in its entire length (types A, B and C) varicose veins were present in 34% of cases. This may indicate that the subcutaneous vein which assumes the role of a saphenous vein becomes more readily varicose, probably because it is not protected by the superficial fascia. In such cases the anatomical pattern classified as types D and E might be a predisposing factor for varicose veins.42
T vein
A very constant tributary vein (known as the T vein), has been described.48 It runs horizontally from the lateral aspect of the leg just distal to the knee and joins the GSV at the same level in a right-angle fashion (see Fig. 1.7), running inside the fascial compartment for a long distance (Fig. 1.30). It is consistently fed by reflux varicose veins, which are clinically visible in the paratibial region and/or in the lateral aspect of the leg.
The anterior accessory saphenous vein
When two veins are present in the proximal third of the great saphenous compartment, the medially situated vein is the GSV while the laterally placed vein is the AASV.33,38–41 The AASV is recognized and distinguished from the GSV by the ‘alignment’ sign37 (see Fig. 1.19A), and may also have its own ‘eye’ (see Fig. 1.19B).3
The anatomy of the AASV was studied by transverse DUS of the saphenous compartment of the thigh in 172 consecutive limbs. In 48% of cases only the GSV was present while in 41% both GSV and AASV were present. In these cases the AASV was invariably thinner than the GSV (average 2.4 vs 4.0 mm). Proximally, the ASV joined the GSV close to the SFJ and only rarely (in 3% of cases) terminated directly into the femoral vein. Following the vein backwards, the AASV pierced the superficial fascia and exited the saphenous compartment at a distance of 7–30 cm (average 16 cm), continuing distally as a subcutaneous collateral in an anterolateral direction in 72% of cases, in a medial or anterior direction in 11%, and as more branches and in more directions in 11%. In 6% of cases the AASV joined the GSV without leaving the saphenous compartment (Fig. 1.31).38
In the remaining 11% of the 172 observed limbs only one vein was observed in the proximal part of the saphenous compartment, but according to the ‘alignment’ sign this vein was the AASV, while there was no GSV in the expected position (saphenous aplasia) (Fig. 1.19C). In these cases, 18–20 cm distally from the SFJ the AASV curved medially and continued downwards along the typical course of the GSV.38
The AASV is of clinical importance because in patients with varicose veins it may be the only proximal reflux source while the GSV remains competent3,46 (Fig. 1.32), or alternatively, both GSV and ASV may be incompetent. In a retrospective study of 1450 varicose limbs the AASV was involved in 14% (203/1450) of cases (M. Cappelli, personal communication, 2000). The possible patterns of GSV and/or AASV incompetence at the SFJ are described elsewhere.33
Small Saphenous Vein
The SSV begins behind the lateral malleolus as a continuation of the lateral marginal foot vein. It ascends up the posterior aspect of the calf to empty into the popliteal vein. The SSV lies for its entire length in an intrafascial compartment delimited deeply by the aponeurotic (muscular) fascia and superficially by the superficial fascia. The distal part of this compartment appears on transverse scan, especially in fat legs, as an ‘eye’ similar to that of the GSV in the thigh. The proximal part of the compartment is typically of a triangular shape and is delimited by the medial and lateral heads of the gastrocnemius muscle and the superficial fascia that stretches over the intermuscular groove (Figs 1.12B, 1.21).3,36 The SSV may occasionally be duplicated, with two (or even three) veins of various lengths running into the saphenous compartment.
Saphenopopliteal junction
The saphenopopliteal junction (SPJ) is situated, typically, within 5 cm proximal to the popliteal crease. In some cases the SSV merges with the gastrocnemius vein before joining the popliteal vein (Fig. 1.33). This has been found in 26% of cases in a series of 83 consecutive limbs with incompetent and dilated SSV.49 Another point of surgical interest is that the SSV may join the popliteal vein at different sites on its circumference. In the above-mentioned series the SPJ was lateral in 42%, posteromedial in 30%, posterior in 15%, posterolateral in 12% and even anterolateral in 1% of cases.49 Astonishingly, in normal subjects it has been observed that in about 50% of cases the SSV has no popliteal junction at all (see next paragraph), or is just a tiny vessel (Fig. 1.34).50 In some cases, the SSV may be hypoplasic or absent.
Thigh extension of the SSV
In 1873 Giacomini described in detail the thigh extension (TE) of the SSV (Fig. 1.35).51,52 Further anatomical dissections confirmed that the SSV extends into the thigh in about 50% of cases; in a third of these the SSV joins the popliteal vein (Fig. 1.36A) and then continues up into the thigh, while in the remaining two-thirds of cases the SSV continues into the thigh without any connection with the popliteal vein53 (Figs 1.34, 1.36B). The anatomy of the TE of the SSV (also known as vein of Giacomini or femoropopliteal vein) has been confirmed by USI. The distal portion of the TE is recognized on DUS by its intrafascial position into a triangle-shaped compartment that resembles the GSV compartment and is delimited by the semitendinous muscle (medially), the long head of the biceps muscle (laterally) and the superficial fascia that stretches over the intermuscular groove (see Figs 1.9, 1.16, 1.21A). Proximally, the TE may join the GSV at various distance from the SFJ as intersaphenous thigh anastomosis (the ‘true’ Giacomini vein); continue straight up into the gluteal area as a single vein or be divided into many deep and superficial branches; join the deep femoral veins as a posterior or posterolateral thigh perforator; or divide into many muscular or subcutaneous branches of the posterior thigh. In many cases the proximal ending of the TE is a combination of the above-mentioned terminations. The possible variants of proximal termination of the TE are presented in Figure 1.35. The TE may transmit reflux from the incompetent SFJ and/or groin, gluteal and thigh perforators and/or collaterals to the SSV, or, vice versa, from the incompetent SPJ up to the GSV and/or varicose veins of the posterior thigh (Fig. 1.37A, B).3,33
Arrangement of the SSV and its collaterals
As with the GSV the subcutaneous collaterals of the SSV/TE are recognized because they pierce the superficial fascia to enter the saphenous compartment. One particular superficial collateral of the SSV deserves separate description. It is the so-called ‘popliteal area vein’ and was described by Dodd.54 This vein runs subcutaneously along the posterior aspect of popliteal area, calf and leg, sometimes parallel to the SSV, and typically has a separate junction with the popliteal vein, usually lateral to the SPJ (Fig. 1.38).3,55
Foot Veins
The arrangement of the superficial veins in two layers separated by the superficial fascia is also present in the foot and can be demonstrated by DUS. The dorsal venous arch and the medial and lateral marginal veins are the anatomical origin of the great and small saphenous veins, respectively, and are similarly placed under the superficial fascia (Fig. 1.39). The collateral veins on the dorsum of the foot are the continuation of the subcutaneous collaterals of the leg and are also subcutaneous (Fig. 1.40).56
Perforating veins
Perforating veins were first described in 1803 by Van Loder.57,58 They occur from the ankle to the groin, connecting the superficial veins to the deep veins; they ‘perforate’ the aponeurotic fascia, giving them their name. The fascial point of perforation is always visible with USI (Fig. 1.41).
The average number of perforating veins per leg has been found to be as great as 15559 or as few as 64.60 They are not distributed regularly along the limb’s surface but increase in density from proximal to distal in a 1 : 2 : 8 proportion between the thigh, the leg, and the foot.61
Sixty percent of perforating veins, always the ones that are more important and named, are accompanied by an artery59 (Fig. 1.42); and usually contain one to three one-way valves, depending on their length (see Fig. 1.41). These one-way valves can be thought of as check valves, which serve to prevent high venous pressure (from muscle contraction) from being transmitted to the superficial veins. Normally, perforating veins are thin walled, varying in diameter from less than 1 mm to 2 mm.62 They may also be valveless, especially when less than 1 mm in diameter.63 In such cases, their competence is maintained by their oblique orientation through muscle, or by the ‘S’ shape that they display (Fig. 1.43).
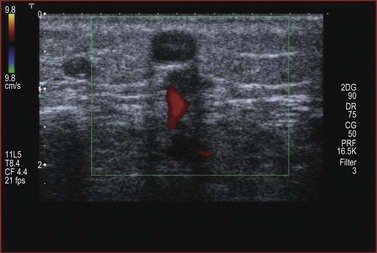
Figure 1.42 Perforators are usually accompanied by an artery easily visible by duplex and color ultrasound.
With muscular contraction, the deep fascia is tightened and the S curves are compressed. This puts the perforator veins under tension, closes the vein and prevents blood from escaping from the deep veins of the calf muscle pump into the superficial veins. Although variable in location, a number of perforating veins occur with marked regularity (Table 1.2).
Table 1.2 Distribution of incompetent perforator veins on 901 lower limbs
Percentage of Limbs with Incompetent Veins | ||
---|---|---|
Perforator Veins | Right Limbs | Left Limbs |
Saphenofemoral junction | 100.00 | 100.00 |
Saphenopopliteal junction | 15.0 | 15.5 |
Mid-Hunterian perforator | 7.0 | 6.7 |
Genicular perforator | 2.9 | 1.6 |
Lateral thigh perforators | 1.8 | 1.3 |
13.5-cm midcalf Cockett | 15.9 | 17.3 |
18.5-cm midcalf Cockett | 34.3 | 35.2 |
24-cm midcalf Cockett | 20.0 | 19.6 |
30-cm midcalf Cockett | 13.0 | 12.7 |
35-cm midcalf Cockett | 6.6 | 7.0 |
40-cm midcalf Cockett | 4.2 | 3.1 |
Calf perforators (other) | 12.0 | 11.2 |
Gastrocnemius/peroneal muscle perforator | 25.0 | 24.0 |
Anterior tibialis/peroneal perforator | 3.1 | 2.9 |
Lateral tibial perforators | 0.02 | 0.04 |
Lateral foot perforators | 2.0 | 2.4 |
Medial foot perforators | 3.5 | 2.9 |
Modified from Sherman RS: Ann Surg 130:218, 1949.
The most important perforators in the thigh are known as the Hunterian and the Dodd perforator(s), which are located in the medial thigh. These connect the GSV to the femoral vein in the middle third of the medial thigh (Hunterian) and the lower third of the thigh (Dodd).64 Incompetence of the midthigh (Hunterian) perforator is a common cause for medial thigh varicose veins in patients with a competent SFJ.
Perforating veins have also been described in the foot. Raivio65 has documented more than 40. One is situated about 2.5 cm below the inferior tip of the medial malleolus. A second occurs approximately 3.5 cm below and anterior to the medial malleolus. The other two are on an arc approximately 3 cm anterior to and below the medial malleolus. Perforating veins in the foot are valveless or have one-way valves that are reversed to allow blood to flow from the deep to the superficial veins.66 The great number of perforating veins and venous anastomoses of the foot allows for their safe removal.
Venous valvular system
Fabricius of Aquapendente (1533–1620) is credited with being the first to detail the anatomy of veins and their valves, in Padua in 1579. He suggested that valves ‘… insure a fair distribution of the blood … prevent distention … and stop blood from flooding into the limb …’.67 However, a more recent historical review credits the Parisian Charles Estienne with mentioning venous valves in 1545 and Lusitanus and Cannano publicly demonstrating valves in Ferrera, Italy in 1555.68
The valves appear as translucent, thin structures that vibrate with blood flow. Numerous bicuspid valves appear down to vein diameters less than 100 micrometers (µm).69 Recent studies demonstrate valves in venules as small as 40 µm in diameter.70,71
Studies of the embryologic development of veins show that the number of venous valves decreases in utero with fetal maturity. It has been suggested that this disappearance continues, albeit at a reduced but variable rate, during childhood, adolescence and adult life.72,73
A morphologic study of normal saphenous veins removed from cadavers has revealed an average of 8.7 valves, with 6.3 of these appearing above the knee and 2.4 below the knee.74 Aging in itself does not appear to decrease the number of venous valves of the leg, nor does the number of venous valves appear to differ between men and women.75
The number of venous valves has been found to be fewer in varicose veins than in normal veins. Valvular insufficiency occurs in undamaged valves as well as damaged valves. Competent venous valves withstand pressures of up to 3 atmospheres.76 Therefore, for incompetence to occur, the valve annulus dilates to render the valves incompetent. This observation is supported by investigations that reveal no difference in viscoelastic behavior in perivalvular vein wall tissue.77 Chronic venous dilation may lead to sclerosis. It is postulated that this is caused by turbulent blood flow.78 However, sinus wall and valvular defects have been found in autopsy studies in up to 90% of adults without apparent varicosities.79 Therefore, valve and valvular sinus abnormalities, at best, comprise only one factor in the development of varicose veins. A full explanation of the pathophysiologic significance of valvular deficiency and dysfunction is addressed in Chapter 3.
Nerves of the Leg of Phlebologic Interest
The peroneal branch of the SuN (nervus cutaneus lateralis surae – NCLS) originates from the common peroneal nerve. This nerve comes down laterally to the popliteal fossa along the biceps femoris muscle to the head of peroneus. During this course it sends a ‘communicating branch’, the NCLS, directed distally and medially, to join the NCMS to complete the SuN (Fig. 1.44).
This typical anatomical arrangement (Fig. 1.45), has great variations. The two branches can run independently.80 The point of contact with the SSV may be found by DUS at different levels of the calf. This point has been called the ‘risk point’ because possible nerve injury during VV treatments81 is more probable from this point distally.82
The SaN takes origin from the femoral nerve 2 cm below the inguinal ligament, comes down along the adductor canal following the femoral artery, continues behind the sartorius muscle, becoming superficial at the knee where it runs between the sartorius and gracilis muscle tendons. At this point the nerve is visible by DUS behind the GSV and in deeper position. Progressively the SaN becomes superficial and runs anterior to the GSV, coming in close contact with the vein (Fig. 1.46) at about 2–3 cm below and medial to the tibial tuberosity. From this point down the nerve follows the GSV extending to the foot. It is also possible to identify the ‘risk point’ for the SaN (Fig. 1.47).83–86
Histology
Vein walls
The first part of the venous system consists of the venule, which serves as a collecting tube for capillaries. The cutaneous microcirculation is organized as two horizontal plexuses. One is situated 1–1.5 mm below the skin surface. The other is at the dermal subcutaneous junction. Arterioles ascending into these layers and venules descending are paired while they connect the two plexuses. The arterial capillaries form dermal papillary loops and at the dermal subcutaneous junction collecting veins contain bicuspid valves oriented to prevent retrograde flow of blood.87 The venule is approximately 20 µm in diameter and consists of an endothelium surrounded by a fibrous tissue composed of a thin layer of collagenous fibers. The venule increases in diameter, with smooth muscle cells appearing within the fibrous sheath when the diameter is approximately 45 µm. At a diameter of 200 µm, the muscular layer becomes better defined. At a clinically recognizable diameter consistent with small phlebectasia (venectasia), the vessels are composed of a thick media with myocytes. Collagenous fibers are clearly organized into bundles, and elastic fibers can be observed.88 Larger diameters contain elastic fibers and a more organized structure.
Telangiectasias commonly seen in the skin of lower extremities can be explained by abnormalities in the organization and ultrastructure of the cutaneous microvasculature rather than by neovascularization. The telangiectasias seen in essential telangiectasia and in scleroderma are clearly a dilation of the postcapillary venules of the upper horizontal plexus.89
Microscopically, the normal young internal saphenous vein is a musculofibrous conduit with both passive and active functions. The normal vein is slightly oval, with the short axis perpendicular to the skin. In response to an increase in intraluminal pressure, the diameter increases and the vein loses its oval appearance. Veins tend to assume an elliptical shape, particularly at low transmural pressures. These qualities of shape deformability allow veins to change volume with very little force, thus aiding their role as a high-capacitance system.90,91 With continued increases in venous pressure or progression of varicose disease, the vein increases in both length and diameter and becomes tortuous. Whether normal or varicose, the saphenous vein is composed of three tunics: intima, media and adventitia.
The intima is a thin structure consisting of a layer of endothelial cells and a deep fenestrated basement membrane bounded by a thin, fragmented elastic lamina.92 The central portion of the cell containing the nucleus bulges into the lumen and, on its free surface, exhibits multiple small microvilli. Although endothelial cells are easily destroyed by chemical and physical insults, they demonstrate a marked capacity for regeneration.93
The media is composed of three layers of muscle bundles. The inner layer consists of small bundles of longitudinally arranged muscle fibers. Loose connective tissue and small elastic fibrils separate the muscle bundles.92 The middle layer is composed of wide bundles of smooth muscle in a circular orientation. The muscle bundles may be separated by thin or thick layers of elastic fibrils.92 In addition, the outer layer is quite variable, being composed of longitudinal muscle bundles spread out through thick fibrous tissue. The outermost cells of the circular layer interdigitate with the innermost cells of the longitudinal layer to improve contractile efficiency.94
The amount of muscle within the vein wall is not uniform throughout the venous system. There is an increasing smooth muscle content from the proximal to the distal and the deep to the superficial veins.95 The obvious functional importance is to counteract hydrostatic pressure. In addition, the greatest extent of circular muscle occurs at the level of insertion of the valve leaflets. This composition helps to prevent valvular dilatation and incompetence and is the last region to dilate in a varicose vein.96 This area is known to be dilated in primary valvular insufficiency.
The adventitia is the thickest portion of the vein wall. It is primarily composed of collagen, with interlacing fibers oriented in longitudinal, spiral, and circular fashions.93 In larger vessels of the thigh, a considerable network of elastic fibers occurs and stretches from valve to valve.97 The collagen layer merges with the perivenous connective tissue and contains the vasa vasorum and adrenergic nerve fibers.98,99 The vasa vasorum provides the arteriovenous circulation in the wall of the blood vessel. These vessels arise as branches from arterioles present in perivenous connective tissue that are fed by neighboring arteries.100 Venous capillaries of the vasa vasorum form venules that empty into veins running in the loose perivenous connective tissue. As discussed in Chapter 3, alterations of the vasa vasorum may lead to the development of arteriovenous fistulas.
Venous valves
Venous valves are composed of a thin layer of collagen and a variable amount of smooth muscle covered on both surfaces by endothelium.101 An increase in muscle fibers is found at the base of the valve cusp running circumferentially and longitudinally for a variable distance along the length of the valve cusp.97,102 Elastic fibers extend along the whole length of the cusp and lie close to the endothelium. Collagen fibers are concentrated at the base, thinning out toward the free edge of the cusp. The valve is avascular and thus dependent on humeral blood for its oxygen supply.103,104
Fegan97 proposes that the muscle fibers play an active role in regulating blood flow. Through an evaluation of anatomical dissection of multiple valves, he believes that contraction of the circular muscles at the base of the valve reduces the vein diameter, and contraction of the longitudinal fibers shortens and thickens the cusp. This type of coordinated muscle action maintains tone in the vein wall in the face of increased pressure from retrograde blood flow.
The valve cusp changes with age.105 In the parietal layer, collagen becomes thicker and denser with an increase in the elastic lamellae. The luminalis develops deep, narrow depressions. The vein wall at the valve sinus thickens with an increase in adipose tissue, muscle cells and connective tissue. These changes produce less flexibility of the valve cusp, which may produce abnormal blood flow currents and eddies that could lead to valvular incompetence.
Vein wall variation
The composition of vein walls varies with the type and location of the veins. Depending on their location, veins assume many different functions, and the muscular content of the vein wall varies accordingly. They are used as pumps and reservoirs and must withstand variations in gravitational and intravascular pressure demands. The percentage of smooth muscle increases with distal locations. Veins in the lower extremities are the only veins that are composed of more than 40% smooth muscle, with veins in the foot having 60–80% smooth muscle compared with 5% in axillary veins.95 However, the hydrostatic pressure within the vein also correlates with smooth muscle content and superficial veins have more smooth muscle than deep veins.106 The differences in vein wall content may affect sclerotherapy treatment, as described in Chapter 7.
The function of the vein wall collagen is to prevent overdistension, whereas elastin produces elastic recoil. With advancing age, multiple changes may occur in the vessel wall. The intima thickens, increasing and disorienting elastic fibers.92 The media develops a more disorganized arrangement of muscle bundles and hypertrophy of the outer muscular layer. Elastic fibers become more irregular and dystrophic and the elastic lamina becomes more fragmented, atrophic, thin and irregular.92 The adventitia becomes increasingly fibrous. Thus the lack of an organized elastic support and smooth muscle degeneration in an aged vein render it more susceptible to pressure-induced distension.
Some histologic studies demonstrate that fibrotic wall changes are a common finding in the GSV in all age groups without venous disorders.106 The incidence of fibrotic change increases from 25% to 50% in the population under 40 years of age to 100% in those over 70 years of age.
Other studies have confirmed the fact that varicose saphenous veins have significantly larger wall areas and larger amounts of collagen. This is true more so in the proximal GSV compared with the distal GSV. Also there is excess smooth muscle and elastin in varicose veins proximally compared with distally. This has suggested to some that varicose veins are a dynamic response to venous hypertension. Others believe that the vein wall in varicose disease is thinned rather than dynamically responsive.107
In saphenous veins subjected to biopsy during arterial bypass surgery, intimal thickening has been found to be common. Smooth muscle hyperplasia, elastosis and fibrosis contribute to this intimal thickening. In addition, medial longitudinal muscle hypertrophy is seen.108
Venules
Venules in the upper and mid dermis usually run in a horizontal orientation. The diameter of the postcapillary venule ranges from 12 mm to 35 mm. Collecting venules range from 40 mm to 60 mm in the upper and mid dermis and enlarge to become 100 to 400 mm in diameter in the deeper tissues.109 One-way valves are found at the subcutis (dermis)–adipose junction on the venous side of the circulation.70 Valves are usually found in the area of anastomosis of small to large venules and also within larger venules unassociated with branching points. The free edges of the valves are always directed away from the smaller vessel and toward the larger and serve to direct blood flow towards the deeper venous system. The structure of these valves is identical to that of the valves found in deep and larger veins.
Postcapillary venules are composed of endothelial cells covered by a basement membrane, some collagen fibers, and, rarely, smooth muscle cells. Collecting veins in the deep dermis gradually receive more muscle cells until they become veins with a continuous muscle coat (see Fig. 1.48).110,111
Telangiectasias
Histologic examination of simple telangiectasias demonstrates dilated blood channels in a normal dermal stroma with a single endothelial cell lining, limited muscularis, and adventitia.112 Therefore, such vessels probably evolve from capillaries or early venules.
Blue-to-red arborizing telangiectasias of the lower extremities are probably dilated venules, possibly with intimate and direct connections to underlying larger veins of which they are direct tributaries.113–115 Electron microscopic examination of ‘sunburst’ varicosities of the leg has demonstrated that these vessels are widened cutaneous veins.88 They are found 175 to 382 µm below the stratum granulosum. The thickened vessel walls are composed of endothelial cells covered with collagen and muscle fibers. Elastic fibers are also present. Electron microscopy reveals an intercellular collagenous dysplasia, lattice collagen and some matrix vesicles. These findings suggest that telangiectatic leg veins, like varicose veins, have an alteration of collagen metabolism of their walls. Therefore, like varicose veins, these veins are dysplastic.
Alternatively, arteriovenous anastomoses may result in the pathogenesis of telangiectasias. These were demonstrated by de Faria and Moraes115 in 1 of 26 biopsy specimens of leg telangiectasias.
Skin biopsy of more unusual forms of telangiectasia, such as unilateral nevoid telangiectasia, may show an accumulation of mast cells.116 In these cases permanent vasodilation may be induced by the chronic release of one or more products of mast cells, particularly heparin.117,118
Innervation
Innervation of the vein plays an important part in the regulation of venous tone. Different stimuli are known to produce venous constriction: pain, emotion, hyperventilation, deep breathing, Valsalva’s maneuver, standing and muscular exercise.119 Although muscular veins have little or no sympathetic innervation, cutaneous veins are under hypothalamic thermoregulatory control and have both α- and β-adrenergic receptors.120 Because the outermost media and adventitia contain the nerve endings, myogenic conduction contributes to the neurogenic activation by coordinating venous contraction.102,121 Even in the outer layers of the media, the separation of muscle cells from nerve endings is rarely less than 1000 Å (0.1 nm).122 Therefore, an intact smooth muscle layer is important in vein physiology.
Venous constriction and dilation occur through both central and local nervous stimuli.123 This may be problematic when veins are used as arterial conduits. One report describes spasms of a vein graft 14 months after operation, causing anginal symptoms.124 Localized cooling provides both a potentiation of adrenergic stimulation and a direct stimulus for venous smooth muscle contraction;125,126 venoconstriction is reduced by warming.126 Venoconstriction also occurs with infusions of norepinephrine (noradrenaline),127 epinephrine (adrenaline), phenylephrine, serotonin and histamine.128 Veins dilate in response to phenoxybenzamine, phentolamine, reserpine, guanethidine, barbiturates and many anesthetic agents.129 Therefore, circulating adrenergic and pharmacologic substances influence vein diameter and this may explain why central mechanisms may also be responsible for venous tone. Evidence for a central sympathetic control of venoconstriction has been demonstrated by the failure of such venoconstriction to occur with the tilting of sympathectomized patients.130 Even the stress of mental arithmetic or unpleasant thoughts has been shown to activate adrenergic nerves connected to cutaneous veins.119 Veins may become more distensible during sleep because of a change in either respiration or nerve stimulation.131 This is one reason for recommending continuous compression of sclerotherapy-treated veins during the endosclerotic stages after treatment (see Chapter 8).
Local chemical changes produced through exercise also provide for the distribution of blood flow in accordance with local metabolic needs. Venous smooth muscle is also sensitive to endothelium-derived vasoconstrictor substances and peptides such as endothelin.124 Finally, the increasing smooth muscle content from proximal to distal veins, and a thicker muscular media in superficial veins compared with muscular deep veins, supports the physiologic concept of increasing venous contractility in the distal venous system.
1 Federative Commitee on Anatomical Terminology. Terminologia Anatomica. Stuttgart – New York: Thieme, 1998.
2 Caggiati A, Bergan JJ, Gloviczki P, et al. Nomenclature of the veins of the lower limbs: an international interdisciplinary consensus statement. J Vasc Surg. 2002;36:416.
3 Ricci S, Georgiev M. Ultrasound anatomy of the superficial veins of the lower limb. J Vasc Technol. 2002;26:183.
4 Eklof B, Rutherford RB, Bergan JJ, et al. American Venous Forum International Ad Hoc Committee for Revision of the CEAP Classification: Revision of the CEAP classification for chronic venous disorders: consensus statement. J Vasc Surg. 2004;40:1248.
5 Allegra C, Antignani P-L, Bergan JJ, et al. The ‘C’ of CEAP: suggested definitions and refinements: an International Union of Phlebology conference of experts. J Vasc Surg. 2003;37:129.
6 Bundens WP, Bergan JJ, Halasz NA, et al. The superficial femoral vein: a potentially lethal misnomer. JAMA. 1995;274:1296.
7 Pieri A, Gatti M, Santini M, et al. Ultrasound anatomy of the deep veins of the lower limb extremity. J Vasc Technol. 2002;26:201.
8 Franceschi C, Zamboni P. Principles of hemodynamics. New York: Nova Science Publishers Inc.; 2009.
9 Kubik S. Das Venensystem der unteren Extremitat. Eine anatomische Ubersicht-Anwendungsmoglichkeiten fur die Praxiz. Der informierte Arzt. 1985;4:31.
10 Lejars C. Les veines de la plante du pied chez l’homme et les grands animaux, Arch Physiol. XXII, Ser.V TomeII/I, 1890, p 89–103.
11 Gillot C. La semelle de Lejars. Phlébologie. 1993;46:173.
12 Tibbs DJ, Sabiston DC, Davies MG, et al. Varicose veins, venous disorders, and lymphatic problems in the lower limbs. Oxford University Press; 1997.
13 Caggiati A. Anatomia clinica del circolo venoso degli arti inferiori, Ch 4. In: Agus G: Chirurgia delle varici, EDRA 2006, Milano.
14 Caggiati A, Bergan JJ. The saphenous vein: derivation of its name and its relevant anatomy. J Vasc Surg. 2002;35:172.
15 Somjen GM. Anatomy of the superficial venous system. Dermatol Surg. 1995;21:35.
16 Berry SM, Susman B, Ibrahim IM, et al. Determination ‘good’ saphenous vein for use in in-situ bypass grafts by real-time, B-mode imaging. J Vasc Technol. 1988;12:184.
17 Browse NL, Burnand G, Thomas ML. Diseases of the veins: pathology, diagnosis, and treatment. London: Edward Arnold; 1988.
18 Sherman RS. Varicose veins: anatomy re-evaluation of Trendelenburg tests and operating procedure. Surg Clin North Am. 1964;44:1369.
19 Kupinski AM. The lesser saphenous vein: an under-utilized arterial bypass conduit. J Vasc Technol. 1987;11:145.
20 Dodd H, Cockett FB, editors. The pathology and surgery of the veins of the lower limb, 2nd ed, Edinburgh: Churchill Livingstone, 1976.
21 Hoffman HM, Staubesand J. Die venosen Abflussverhaeltnisse des Musculus triceps surae. Phlebologie. 1991;20:164.
22 Georgiev M, Myers K, Belcaro G. The thigh extension of the lesser saphenous vein: from Giacomini’s observations to ultrasound scan imaging. J Vasc Surg. 2003;37:558.
23 Georgiev M. The femoropopliteal vein: ultrasound anatomy, diagnosis, and office surgery. Dermatol Surg. 1996;22:57.
24 Caggiati A. Fascial relationships of the short saphenous vein. J Vasc Surg. 2001;34:241.
25 Taylor GI, Caddy CM, Watterson PA, Crock JG. The venous territories (venosomes) of the human body: experimental study and clinical implications. Plast Reconstr Surg. 1990;86:185.
26 Delis KT, Knaggs AL, Khodabakhsh P. Prevalence, anatomic patterns, valvular competence, and clinical significance of the Giacomini vein. J Vasc Surg. 2004;40:1174.
27 Albanese AR, Albanese AM, Albanese EF. The lateral subdermic venous system of the legs. Vasc Surg. 1969;3:81.
28 Caggiati A, Ricci S. The long saphenous vein department. Phlebology. 1997;12:107.
29 Bailly M. Carthographie CHIVA. In: Encyclopedie Médico-chirurgicale, Paris 43–161-B, 1993. p 1–4.
30 Cruveilhier J. Traité d’anatomie descriptive, 2°, Tome troisième. Paris: Ancienne Maison Béchet Jeune; 1843.
31 Bayle, Hollard, Lauth: Trattato elementare d’Anatomia generale, descrittiva e topografica, Napoli: Federico Stikler, 1843. p 279.
32 Thomson H. The surgical anatomy of the superficial and perforating veins of the lower limb. Ann R Coll Surg Engl. 1979;61:198.
33 Ricci S, Georgiev M, Goldman MP. Anatomical bases of ambulatory phlebectomy, Ch 2. In: Taylor ED, editor. Ambulatory phlebectomy. Boca Raton: Taylor & Francis, 2005.
34 Zamboni P, Cappelli M, Marcellino MG, et al. Does a saphenous varicose vein exist? Phlebology. 1997;12:74.
35 Lemasle P, Baud JM, Lefebvre-Villardebo M, Uhl JF. Proposition d’une définition échographique de la grande saphène et des saphènes accessoires à l’étape crural. Phlébologie. 1996;49:279.
36 Cavezzi A, Labropoulos N, Partsch H, et al. Duplex ultrasound investigation of the superficial veins and perforators in chronic venous disease of the lower limbs, part II: Anatomy. Eur J Vasc Endovasc Surg. 2006;31:288.
37 Bailly M, Nasso C, Ballo M. Ecografia venosa ed esperienza CHIVA. Flebologia. 1997;8:117.
38 Ricci S, Georgiev M, Cappelli M. Définition de la veine saphène accessoire antérieure et de son role dans la maladie variqueuse. Phlébologie. 2004;57:135.
39 Sherman RS. Varicose veins. Further findings based on anatomic and surgical dissections. Ann Surg. 1949;130:218.
40 Shah DM, Chang BB, Leopold PW, et al. The anatomy of the greater saphenous venous system. J Vasc Surg. 1986;3:273.
41 Kupinski AM, Evans SM, Khan AM, et al. Ultrasonic characterization of the saphenous vein. Cardiovasc Surg. 1993;1:513.
42 Ricci S, Cavezzi A. Echo-anatomy of long saphenous vein in the knee region: proposal for a classification in five anatomical patterns. Phlebology. 2002;16:111.
43 Georgiev M. The femoropopliteal vein: ultrasound anatomy, diagnosis and office surgery. Dermatol Surg. 1996;22:57.
44 Caggiati A. Fascial relationships of the short saphenous vein. J Vasc Surg. 2001;34:241.
45 Ricci S, Caggiati A. Echoanatomical patterns of the long saphenous vein in patients with primary varices and in healthy subjects. Phlebology. 1999;14:54.
46 Pieri A, Vannuzzi A, Duranti A, et al. Ròle central de la valvule pré-ostiale de la veine saphène interne dans la genèse des varices tronculaires des membres inférieurs. Phlébologie. 1995;48:227. plus errata Phlébologie 48:V, VI, 1995
47 Cavezzi A, Carigi V, Collura M. Colour flow duplex scanning as a preoperative guide for mapping and for local anaesthesia in varicose vein surgery. Phlebology. 2000;15:24.
48 Zamboni P, De Palma M, Carandina S, et al. The ‘T’ vein of the leg. Dermatol Surg. 2004;30:750.
49 Lemasle PH, Uhl JF, Lefebre-Vilardebo M, et al. Confrontation écho-chirurgicale de la terminaison de la saphéne externe dans le cadre de la chirurgie d’exerèse. Résultats préliminaires. Phlébologie. 1995;48:321.
50 Van der Stricht J. La petite veine saphène existe-t-elle. Phlébologie. 2001;3:309.
51 Giacomini C. Osservazioni anatomiche per servire allo studio della circolazione venosa delle estremità inferiori. Giornale della reale Accademia di Medicina di Torino. 1873;13:109.
52 Georgiev M, Myers K, Belcaro G. Giacomini’s observations ‘on the superficial veins of the abdominal limb and principally the external saphenous’. Int Angiol. 2001;20:225.
53 Hoffman HM, Staubesand J. Die venosen abflussverhaeltnisse der musculus triceps surae. Phlebologie. 1991;20:164.
54 Dodd H. The varicose tributaries of the popliteal vein. Br J Surg. 1965;52:350.
55 De Palma M, Carandina S, Mazza P, et al. Perforator of the popliteal fossa and short saphenous vein insufficiency. Phlebology. 2005;20:170.
56 Ricci S. Phlébectomie des varices du pied, 53. Phlébologie, 2000.
57 Von Loder JC. Anatomische Tafeln zur Beforderung der Kenntniss des menschichen Korpers. Waimar. 1794.
58 Caggiati A, Mendoza E. The discovery of perforating veins. Ann Vasc Surg. 2004;18:502.
59 Van Limborgh J. L’anatomie du systeme veineux de l’extremite inferieure en relation avec la pathologie variqueuse. Folia Angiol. 1961;8:240.
60 Thompson H. The surgical anatomy of the superficial and perforating veins of the lower limb. Ann R Coll Surg Engl. 1979;61:198.
61 Thulesius O, et al. Blood flow in perforating veins of the lower extremity. In: May R, Partsch H, Staubesand J, editors. Perforating veins. Munich: Urban & Schwarzenberg, 1981.
62 Bjordal RI. Circulation patterns in incompetent perforating veins in the calf and in the saphenous system in primary varicose veins. Acta Chir Scand. 1972;136:251.
63 Sarin S, Scurr JH, Coleridge Smith PD. Medial calf perforators in venous disease: the significance of outward flow. J Vasc Surg. 1992;16:40.
64 Dodd H. The varicose tributaries of the superficial femoral vein passing into Hunter’s canal. Postgrad Med J. 1959;35:18.
65 Raivio EVL. Untersuchungen uber die venen der unteren extremitaten mit besonderer Berucksichtigung der gegenseitigen Verbindungen zwischen den oberflachlichen und tiefen Venen. Ann Med Exp Fenn. 1948;26(Suppl):1.
66 Askar O, Kassem KA, Aly SA. The venographic pattern of the foot. J Cardiovasc Surg. 1975;16:64.
67 Laufman H. Surgery: the veins. Austin, Texas: Silvergirl; 1986.
68 Scultetus AH, Villavicencio JL, Rich NM. Facts and fiction surrounding the discovery of the venous valves. J Vasc Surg. 2001;33:435.
69 Aharinejad S, Lametschwandtner A. Microvascular corrosion casting in scanning electron microscopy: techniques and applications. New York: Springer-Verlag; 1992.
70 Braverman IM, Keh-Yen A. Ultrastructure of the human dermal microcirculation. IV. Valve-containing collecting veins at the dermal-subcutaneous junction. J Invest Dermatol. 1983;81:438.
71 Dunn RM, Fudem GM, Walton RL, et al. Free flap valvular transplantation for refractory venous ulceration. J Vasc Surg. 1994;19:523.
72 Myers TT. Varicose veins. In Allen EV, Barker NW, Hines EA, editors: Peripheral vascular diseases, 3rd ed, Philadelphia: Saunders, 1962.
73 Thurner J, Mar R. Probleme der phlobopathologie mit besonderer berucksichtigung der phlebosklerose. Zentralbl Fuer Phlebologie. 1967;6:404.
74 Ortega F, Sarmiento L, Mompeo B, et al. Morphological study of the valvular distribution in the long saphenous vein. Phlebology. 1994;9:59.
75 Kosinski C. Observation on the superficial venous system of the lower extremity. J Anat. 1926;60:131.
76 Last RJ. Anatomy: regional and applied, 6th ed. Edinburgh: Churchill Livingstone; 1978.
77 Psaila JV, Melhuish J, Conboy V, et al. Do varicose veins have abnormal viscoelastic properties? In: Davy A, Stemmer R, editors. Phlébologie ’89. Blanche, France: John Libbey Eurotext; 1989:77-79.
78 Cotton LT. Varicose veins: gross anatomy and development. Br J Surg. 1961;48:589.
79 Eger SA, Wagner FBJr. Etiology of varicose veins. Postgrad Med. 1949;6:234.
80 Payen B. Rappel anatomique de la veine saphène externe. Phlébologie. 1985;38:453.
81 Sam RC, Silverman SH, Bradbury AW. Nerve injuries and varicose vein. Eur J Vasc Endovasc Surg. 2004;27:113.
82 Ricci S, Moro L, Antonelli Incalzi R. Ultrasound imaging of the sural nerve: ultrasound anatomy and rationale for investigation. Eur J Vasc Endovasc Surg. 2010;39:636-641.
83 Ricci S, Moro L, Antonelli Incalzi R. Visualisation ultrasonique des nerfs du membre inférieur d’intérêt phlébologique. Phlébologie. 2010;63:110.
84 Holme JB, Holme K, Sorensen LS. The anatomic relationship between the long saphenous vein and the saphenous nerve. Acta Chir Scand. 1988;154:631.
85 Price C. The anatomy of the saphenous nerve in the lower leg with particular reference to its relationship to the long saphenous vein. J Cardiovasc Surg. 1990;31:294.
86 Garnjobst W. Injuries to the saphenous nerve following operations for varicose veins. Surg Gynecol Obstet. 1964;119:359.
87 Braverman IM. The cutaneous microcirculation: ultrastructure and microanatomic organization. Microcirculation. 1997;4:329.
88 Wokalek H, Vanscheidt W, Martay K, Leder O. Morphology and localization of sunburst varicosities: an electron microscopic and morphometric study. J Dermatol Surg Oncol. 1989;15:149.
89 Braverman IM. Ultrastructure and organization of the cutaneous microvasculature in normal and pathologic states. J Invest Dermatol. 1989;93(Suppl 2):2S.
90 Moreno AH, Katz AI, Gold LD, Reddy RV. Mechanics of distention of dog veins and other very thin-walled tubular structures. Circ Res. 1970;20:1069.
91 Strandness DEJr, Thiele BI. Selected topics in venous disorders: pathophysiology, diagnosis, and treatment. New York: Futura; 1981.
92 Bouissou H, Julian M, Piraggi M, Louge L. Vein morphology. Phlebology. 1988;3(Suppl 1):1.
93 Farber EM, Bates EE. Pathologic physiology of stasis dermatitis. Arch Dermatol. 1954;70:653.
94 Rhodin Johannes AG. Histology: a text and atlas. New York: Oxford University Press; 1974.
95 Kugelgen AV. Uber das Verhaltnis von Ringmuskulatur und Innendruck in menschlichen grossen Venen. Zeitschr Zellforsch. 1955;43:168.
96 Barrow DW. The clinical management of varicose veins, 2nd ed. New York: Hoeber-Harper; 1957.
97 Fegan G. Varicose veins: compression sclerotherapy. London: William Heinemann; 1967.
98 Vanhoutte PM. The role of systemic veins: an update. Phlebology. 1988;3(Suppl 1):13.
99 Ehinger B, Falck B, Sporrong B. Adrenergic fibers to the heart and to peripheral vessels. Bibl Anat. 1996;8:35.
100 O’Neill JP. The effects on venous endothelium of alterations in blood flow through the vessels in vein walls, and the possible relation to thrombosis. Ann Surg. 1947;126:270.
101 Edwards JE, Edwards AE. The saphenous valves in varicose veins. Am Heart J. 1940;19:338.
102 Butterworth DM, Rose SS, Clarki P, et al. Light microscopy, immunohistochemistry and electron microscopy of the valves of the lower limb veins and jugular veins. Phlebology. 1992;7:27.
103 Hamer JD, Malone PC, Silver IA. The PO2 in venous valve pockets: its possible bearing on thrombogenesis. Br J Surg. 1981;68:166.
104 Saphir O, Lev M. Venous valvulitis. Arch Pathol. 1952;53:456.
105 Saphir O, Lev M. The venous valve in the aged. Am Heart J. 1952;44:843.
106 Leu HJ, Vogt M, Pfrunder H. Morphological alterations of nonvaricose and varicose veins. Basic Res Cardiol. 1979;74:435.
107 Travers JP, Brookes CE, Evans J, et al. Assessment of wall structure and composition of varicose veins in reference to collagen, elastin, and smooth muscle content. Eur J Vasc Endovasc Surg. 1996;11:230.
108 Varty K, Jones L, Porte KE, et al. A quantitative study of long saphenous vein morphology in patients undergoing arterial surgery. Phlebology. 1995;10:90.
109 Miani A, Rubertsi U. Collecting venules. Minerva Cardioangiol. 1958;41:541.
110 Benninghoff, quoted by Moretti G. In: Jadassho J, editor. Handbuch der haut und geschlechtskrangheiten. Berlin: Springer-Verlag, 1968.
111 Bargmann W. Histologie und mikroskopische anatomie des meerschen. Stuttgart: Georg Thieme; 1956.
112 Goldman MP, Bennett RG. Treatment of telangiectasia: a review. J Am Acad Dermatol. 1987;17:167.
113 Bean WB. Vascular spiders and related lesions of the skin. Springfield, Ill: Charles C Thomas; 1958.
114 Bodian EL. Sclerotherapy. Semin Dermatol. 1987;6:238.
115 de Faria JL, Moraes IN. Histopathology of the telangiectasias associated with varicose veins. Dermatologia. 1963;127:321.
116 Fried SZ, Lynfield L. Unilateral facial telangiectasia macularis eruptiva perstans. J Am Acad Dermatol. 1987;16:250.
117 Azizkhan RG, Azizkhan JC, Zetter BR, Folkman J. Mast cell heparin stimulates migration of capillary endothelial cells in vitro. J Exp Med. 1980;152:931.
118 Folkman J. Regulation of angiogenesis: a new function of heparin. Biochem Pharmacol. 1985;34:905.
119 Shepherd JT. Reflex control of the venous system. In: Bergan JJ, Yao JST, editors. Venous problems. Chicago: year Book, 1978.
120 Kaiser GA, Ross JJr, Braunwald E. Alpha and beta adrenergic receptor mechanisms in the systemic venous bed. J Pharmacol Exp Ther. 1964;144:156.
121 Johansson B, Ljung B. Role of myogenic propagation in vascular smooth muscle response to vasomotor nerve stimulation. Acta Physiol Scand. 1968;73:501.
122 Speden RN. Excitation of vascular smooth muscle. In: Bülbring E, et al, editors. Smooth muscle. Baltimore: Williams & Wilkins, 1970.
123 Vanhoutte PM. Venous wall and venous disease. In: Vanhoutte PM, editor. Return circulation and norepinephrine: an update. Paris: John Libbey Eurotext, 1991.
124 Maleki M, Manley JC. Venospastic phenomena of saphenous vein bypass grafts: possible causes for unexplained postoperative recurrence of angina or early or late occlusion of vein bypass grafts. Br Heart J. 1989;62:57.
125 Vanhoutte PM, Shepherd JT. Thermosensitivity and veins. J Physiol (Paris). 1970;63:449.
126 Vanhoutte PM, Shepherd JT. Effect of temperature on reactivity of isolated cutaneous veins of the dog. Am J Physiol. 1970;218:187.
127 Wood JE, Eckstein JW. A tandem forearm plethysmography for study of acute responses of the peripheral veins of man: the effect of environmental and local temperature change and the effect of pooling blood in the extremities. J Clin Invest. 1958;37:41.
128 Thulesius O, Gjores JE. Reactions of venous smooth muscle in normal men and patients with varicose veins. Angiology. 1974;25:145.
129 Mellander S. Operative studies on the adrenergic neuro-hormonal control of resistance and capacitance blood vessels in the cat. Acta Physiol Scand. 1960;50:5.
130 Peterson LH. Some characteristics of certain reflexes which modify the circulation in man. Circulation. 1950;2:351.
131 Watson WE. Distensibility of the capacity blood vessels of the human hand during sleep. J Physiol (Lond). 1962;161:392.