CHAPTER 27 Anatomic Reconstruction of the Posterolateral Corner
Injuries to the posterolateral corner (PLC) of the knee have received increased attention in recent years, with its elusive diagnosis, and potential surgical approaches to guide repair and/or reconstruction are still highly debated. Most injuries to the PLC occur in combination with disruption of the posterior cruciate ligament (PCL) or, to a lesser extent, the anterior cruciate ligament (ACL).1–8 Failure to identify and treat these combined injuries has been reported to cause premature failure of cruciate ligament reconstruction, yet despite numerous surgical advancements enhancing cruciate ligament repair, results regarding PLC reconstruction remain far less predictable.9–17 Perhaps secondary to its complex, often variable anatomy, lack of consensus guiding repair and/or reconstruction, or absence of current methods to assess treatment success, much controversy remains regarding optimal recognition and treatment of these injuries.18,19
Common causes of PLC injury include sports-related trauma, motor vehicle accidents, and falls.1,4,20 Both contact and noncontact injuries have been recounted.21 Injury of the PLC in combination with other ligamentous injuries occurs by a number of different mechanisms, typically involving a combination of knee hyperextension, external rotation, and varus rotation.2,3,7,8,22–25 Posterolateral instability can also follow knee dislocation, and careful physical examination is required to rule out associated neurovascular complication. The incidence of neurovascular damage, most commonly involving the peroneal nerve, have been estimated to be from 12% to 29% following injuries to the PLC.26–27
Clinical manifestations of posterolateral corner insufficiency most notably include functional instability with the knee in extension. This is apparent with stair activity, slopes, or activities that require pivoting or change of direction.28 Gait analysis may reveal a varus or hyperextension thrust, partially compensated for by walking with a slightly flexed knee.3 Stress testing may reveal abnormal tibial external rotation, varus instability, as well as pathologic anterior or posterior translation with concomitant ACL or PCL injuries.1–4,6,20–22,25,29–32
Isolated injury to the PLC is uncommon, and a high degree of suspicion must exist to recognize these often subtle clinical findings skillfully. DeLee and colleagues,4 in a comprehensive review of 735 knees with ligamentous injuries, found that only 1.6% of these subjects had acute isolated posterolateral deficiency. The greatest opportunity to achieve optimal results has been attributed to early repair, usually within 3 weeks from the date of injury, with the goal of obtaining anatomic repair of all injured structures.6,29,33 Stannard and associates18,19 have recommended augmentation, even at the time of primary repair, because of poor success rates. With respect to chronic injuries (more than 3 weeks postinjury), direct repair of individual structures is impractical, and invariably requires reconstructive techniques that should ideally mimic intrinsic anatomic function. Extensive scar tissue formation, secondary physiologic changes to involved structures, and potential alterations in weight-bearing and force-loading kinematics make this a formidable challenge, a monumental task for even the best of surgical technicians.
Numerous techniques to repair or augment the structures of the posterolateral corner have been described, but poor outcomes have been reported in 9% to 37% in clinical series.5,18,19,34 Previous accounts have included advancement and recession of the lateral collateral ligament and arcuate complex, biceps tenodesis, augmentation of the biceps femoris or iliotibial band, autogenous or allograft reconstruction through a transfibular tunnel, and use of a transtibial tunnel technique.9,13,17,35–38 All are performed in an attempt to recreate native fibular (lateral) collateral ligament function, considered the primary restraint to varus loads at full knee extension.
Advancement procedures have also been advocated, premised on tightening PLC structures by proximal relocation on the femur or by distal advancement on the tibia. However, these procedures have traditionally been nonanatomic and/or nonisometric, overconstraining knee motion or creating residual pathologic laxity; both can lead to suboptimal function and potential graft failure with repeated cycling.6,9,13,15,18,19 Furthermore, this technique is dependent on good residual tissue for advancement. Thus, in the absence of bony avulsion or with midsubstance ruptures that create tissue ends not amenable to anatomic repair, most authors recommend anatomic reconstruction. Unfortunately, despite many techniques having been advocated, a glaring lack of objective data exists to support these immeasurable claims.17,35–37
Newer techniques used for reconstruction are based on the creation of dual femoral tunnels that attempt to approximate the anatomic footprints of the popliteus and lateral collateral ligament (LCL) on the lateral femoral condyle.26 Similarly, current transfibular techniques describe a tunnel made in a strictly anterior to posterior direction in the fibula, along with a single isometric femoral tunnel providing two limbs to address varus laxity and abnormal increases in tibial external rotation. Moreover, patients with marked varus alignment and/or a lateral thrust in the stance phase of gait may require a valgus-producing tibial osteotomy prior to ligament reconstruction to re-create more conventional mechanical alignment, enhance repair kinematics, and decrease overall risk of graft failure.9,39
ANATOMY
The anatomic features of the PLC are extremely complex, considerably variable, and likely represent the least understood functional compartment of the knee. Inconsistent terminology, confusing nomenclature, and demonstrable changes in anatomic relationships, specifically evolutionary alterations regarding structures associated with the fibular head, have caused much deliberation concerning its organization.40–42 Terry and LaPrade have provided much clarity to this exposure through cadaveric dissection and study.43
The key elements include the fibular (lateral) collateral, popliteus, and popliteofibular ligaments (Fig. 27-1) Functioning as a unit, these structures, along with associated capsular components, prevent abnormal posterior translation, varus rotation, and coupled external rotation of the tibia.24,31,44,45 In addition, by preventing abnormal translation and rotation, the PLC has a secondary function of protecting the cruciate ligaments from abnormally high tensile loads, which can potentially disrupt the normal healing process following cruciate repair, leading to premature graft failure.3,14,46
The PLC is commonly depicted in terms of its soft tissue arrangement, remarkably defined within the context of the layer concept by Seebacher and coworkers47 and the three-fascia incision technique popularized by Terry and LaPrade43 (Figs. 27-2 and 27-3). The most superficial layer, or layer I, contains the lateral fascia, iliotibial band, and biceps femoris tendon. The middle layer includes the quadriceps retinaculum, patellofemoral ligaments, and patellomeniscal ligament, whereas the deepest layer, or layer III, contains the lateral joint capsule, popliteal muscle-tendon unit, and fibular collateral ligament. The fabellofibular and arcuate ligaments, which are variable in terms of their size and contributions to stability, also reside in the deepest layer.
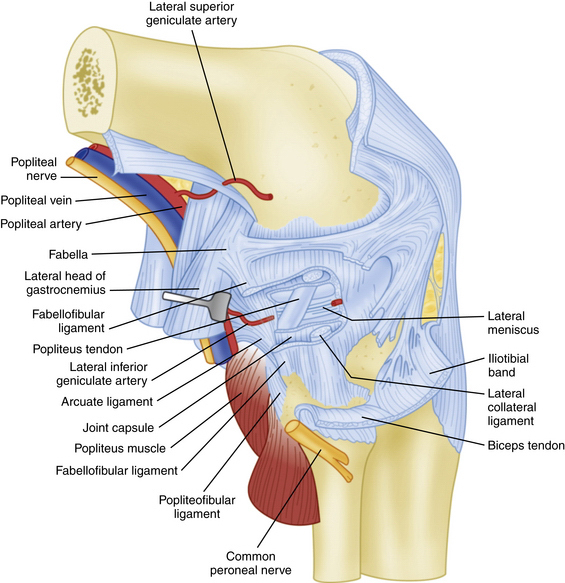
FIGURE 27-3 Lateral view of the posterolateral corner demonstrating relationships of the anatomic structures within the three layers of the knee.
The PLC can also be divided into primary and secondary stabilizers. Primary stabilizers include the LCL and popliteus complex, whereas less prominent contributors include the arcuate complex, posterolateral capsule, and various connections to the lateral meniscus.2,23,46 Similarly, these primary stabilizers can be broken down into static and dynamic stabilizers, with the popliteus tendon complex considered a prime example. The dynamic component includes the popliteus muscle and tendon unit proper, controlling the screw-home mechanism; the static component includes several branching ligamentous structures that insert into the tibia, fibula, and lateral meniscus, respectively.
The strength of the posterolateral compartment can be viewed as a composite effort by its constituents, but the main contributors include the fibular collateral ligament, tendinoaponeurotic portion of the popliteus muscle, and posterolateral capsule. The fibular collateral ligament (FCL) originates just proximal and posterior to lateral femoral epicondyle, slightly anterior to the femoral attachment of the lateral head of the gastrocnemius tendon. It follows an oblique course to insert on the lateral aspect of the fibular head, anterior and distal to the tip of the fibular styloid process (Fig. 27-4). It provides stability against varus loads, most notably at terminal knee extension, and as a secondary restraint to combined external rotation and posterior displacement of the knee. It is a distinct entity from the joint capsule and, unlike the medial collateral ligament, has no attachments to its corresponding meniscus.3,44,45,48
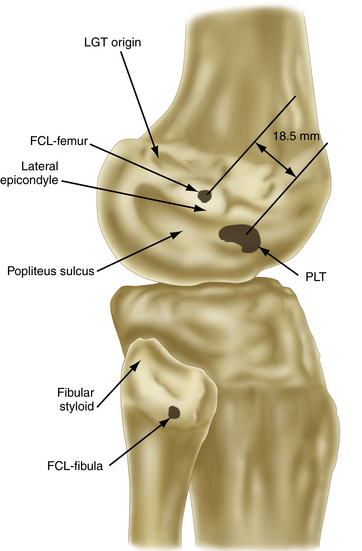
FIGURE 27-4 Relevant insertion site anatomic relationships of the LCL, popliteus tendon, and popliteofibular ligament.
The popliteus muscle arises from the posteromedial proximal tibia, just above the soleal line, extending superiorly and laterally to form a tendinous structure as it courses through the popliteal hiatus. It then inserts on the lateral femoral condyle, anterior and distal to the LCL origin, resisting pathologic external rotation of the tibia from 20 to 130 degrees and excessive varus rotation from 0 to 90 degrees. It also sends fibers to the posterior horn of the lateral meniscus, helping to restrict its excessive forward displacement as the knee assumes a more extended position.49
The popliteofibular ligament originates near the popliteus musculotendinous junction and courses distally and laterally, attaching to the medial aspect of the fibular styloid process.48–50 Anatomic studies have shown it to be present 93% to 100% of the time.23,24 It can be easily be differentiated from the arcuate ligament by the orientation of its fibers, with the proximal third coursing obliquely and fusing with the popliteal tendon, and the remaining distal fibers running vertically, similar to the pattern of the LCL (Fig. 27-5). It helps prevent excessive posterior translation, varus angulation, and primary and coupled tibial external rotation.43 Remarkably, it is as wide as or even wider than the popliteus tendon proper.
The arcuate ligament forms a Y-shaped pattern that begins distally on the styloid process of the fibula, with the medial and lateral limbs both extending proximally. The lateral limb coalesces with the lateral joint capsule and the medial limb attaches to the posterior capsule of the knee.21,23,26,47,48 Studies have shown it to be present in only 24% of cadaveric specimens, whereas the equally as variable fabellofibular ligament has been found to be present in approximately 68%.49 Both act in complementary fashion to stabilize the PLC further when present.
Biomechanics
To understand the PLC fully, one must review the complex interplay between the static and dynamic stabilizers of the knee. Numerous biomechanical studies implementing selective ligament cutting protocols have demonstrated that injury to the PLC results in increased primary external rotation, primary varus rotation, primary posterior translation, and coupled external rotation.3,8,51 In terms of varus rotational stability, the PLC acts as a restraint at lesser degrees of knee flexion, with maximal restraint at 30 degrees.46 Regarding external rotational instability, sectioning of the LCL and PLC capsule has been shown to result in greater posterolateral rotary instability than isolated sectioning of either structure alone.31,44,45 Concerning posterior translational instability, the PLC resists posterior translation at lesser degrees of knee flexion with maximal restraint at 30 degrees, whereas the PCL resists posterior tibial translation at higher degrees of knee flexion, with maximal restraint at 90 degrees.52
Forces on the intact ligaments of the knee after PLC injury can be substantial. The PCL experiences significant increases in forces between 45 to 90 degrees of knee flexion after complete sectioning of the PLC and tibial varus or external rotation.53 Harner and colleagues12 have found a significant increase in the force on a PCL reconstruction graft in PLC-deficient knees, with the PCL graft rendered almost ineffective and, as expected, predisposing it to failure. Combined PCL and PLC injuries have also been shown to produce increased contact pressures in the patellofemoral joint when compared to isolated PCL injuries alone.54 Similarly, tension on the ACL is also increased with PLC injury, particularly with tibial internal rotation in the range of 0 to 20 degrees of knee flexion.
PATIENT EVALUATION
PLC injuries can be subtle and are easily missed if a high degree of suspicion for injury does not exist. Symptoms invariably depend on timing and severity of injury, degree of associated instability, and other sustained defects. Injuries are often classified as grade I, II, or III sprains, corresponding to minimal, partial, or complete tearing, respectively. Quantification of the degree of joint opening in response to varus stress is also commonly used, with a score of 3+ describing an opening of more than 10 mm, with a soft or no appreciable end point.3,