Analyzers
A Analyzers operating on the polarographic principle (Clark electrode) (Figures 37-1 and 37-2)
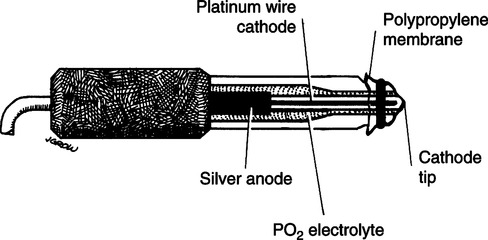
1. The basic overall chemical reaction occurring in electrode system is
< ?xml:namespace prefix = "mml" />
Buy Membership for Pulmolory and Respiratory Category to continue reading. Learn more here