5 Analgesia, Sedation, and Neuromuscular Blockade
Pearls
• Analgesia entails assessment and treatment of pain; sedation entails assessment and treatment of agitation; neuromuscular blockade entails pharmacologic inhibition of voluntary muscle movement.
• Analgesia often improves agitation, but does not necessarily provide sedation; sedation often improves pain, but does not necessarily provide analgesia; neuromuscular blockade provides neither analgesia nor sedation, and should never be induced without first ensuring adequate levels of both analgesia and sedation.
• Most critically ill children experience some degree of pain and agitation: optimal nursing care of critically ill children asks how best to provide appropriate analgesia and sedation, not whether analgesia or sedation should be provided.
• Pain and agitation are usually dynamic processes. Nurses should perform appropriate assessments to monitor pain, agitation, and effectiveness of interventions; reassess patients regularly using consistent tools and modify interventions as needed.
• Change in pharmacologic analgesia and sedation to different agents, or to different routes of administration, requires equipotent conversion to ensure ongoing efficacy and to prevent inadequate or excessive dosing.
• All opioid analgesics and many systemic sedatives can induce physiologic tolerance, and should be weaned gradually after prolonged administration to prevent withdrawal. Appropriate use of these agents does not cause addiction, and they should not be avoided because of such concern.
Introduction
Pain and agitation are common in critically ill patients. As our understanding of these processes in critically ill children has evolved, we now recognize the vital importance of appropriate analgesia and sedation in such patients.48 In 1986, the World Health Organization first published its Analgesic Ladder for the management of cancer pain (Fig. 5-1). This paradigm and others like it are now widely accepted as guidelines for analgesia and sedation in all patients. Despite such advances, considerable progress remains to be made. Caregiver education should enhance awareness of the crucial need for appropriate analgesia and sedation in critically ill children. Practice patterns must continue to change to include appropriate analgesia and sedation as essential components of pediatric critical care. Ongoing research must continue to explore the nature of these complex processes and their optimal management.
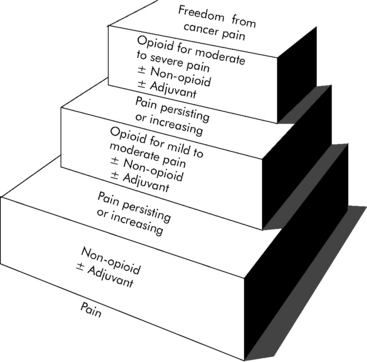
(With permission from: Cancer pain relief and palliative care in children. Companion volume to: Cancer pain relief, with a guide to opioid availability. Geneva 1998, World Health Organization. Available from: http://whqlibdoc.who.int/publications/9241545127.pdf. Accessed October 23, 2010.)
All pharmacologic agents used to provide analgesia, sedation, and neuromuscular blockade have potential side effects and complications, particularly a risk of respiratory depression and cardiovascular compromise. Risk may be higher in pediatric patients, especially when they are developmentally immature or medically fragile.54 Ongoing patient monitoring is essential.
Anatomy, physiology, and embryology of pain
Nociception
Nociception is the normal process through which pain is experienced (Fig. 5-2). Nerve pathways underlying pain sensation in humans are fully developed at term (see Anatomy, Physiology and Embryology of Pain in the Chapter 5 Supplement on the Evolve Website). Most pain in critically ill children is nociceptive in nature and tends to respond to conventional antinociceptive analgesics.
Perception refers to the complex and poorly understood process by which pain becomes a conscious experience. Perception includes the physical sensation of noxious stimuli and the entire conscious experience of such stimuli with attendant emotional and behavioral components.22 Perception is a unique process in each patient and is affected by age, developmental maturity, and underlying medical condition. Perception is also a highly dynamic process, varying among patients and in the same patient at different times.
Modulation refers to modification of pain by other nervous system input. Modulation can ameliorate or exacerbate pain and may explain some of the tremendous variability in subjective pain experience, especially in children. Modulation is a highly complex process and can take place at any point in transduction, transmission, and perception. A particularly important modulatory pathway is the descending pain system, with nerve axons projecting from the brainstem and other supraspinal centers to various laminae of the spinal cord. These descending fibers inhibit transmission of painful sensory stimuli, enhancing analgesia. Anxiety can also modulate pain, causing increased sensitivity to pain and resultant pain-related disability, particularly in chronic pain.40
Classification of Pain
Pain is often classified temporally as acute or chronic, anatomically as somatic or visceral, and pathophysiologically as nociceptive or neuropathic (Table 5-1). Such classifications are not mutually exclusive; clinical presentations may suggest considerable overlap, and clear distinctions are not always possible.
Temporal Classification* | |
Acute Pain | Chronic Pain |
Expected duration, often hours to days | Prolonged duration, often weeks to months |
Somatic or visceral | Somatic or visceral |
More commonly nociceptive | Nociceptive or neuropathic |
Anatomic Classification | |
Somatic Pain | Visceral Pain |
Originates in somatic innervation of periphery | Originates in autonomic innervation of viscera |
Acute or chronic | Acute or chronic |
Nociceptive or neuropathic | Nociceptive or neuropathic |
Pathophysiologic Classification | |
Nociceptive Pain | Neuropathic Pain |
Result of normal nervous system function | Associated with nervous system dysfunction |
Acute or chronic | More commonly chronic |
Somatic or visceral | More commonly somatic |
Typically responds to conventional analgesics | Responds poorly to conventional analgesics |
* Classifications are not mutually exclusive: clinical presentations frequently suggest considerable overlap, and clear distinctions are not always possible.
Temporal Classification of Pain
Pain can be classified temporally as acute or chronic. Acute pain persists for an expected duration, often hours to days. Many medical illnesses and surgical procedures are associated with acute pain of fairly predictable duration with subsequent resolution. Acute pain can be somatic or visceral (see section, Anatomic Classification of Pain). Acute pain in children is most commonly nociceptive, although acute neuropathic pain may be encountered.
Neuropathic Pain
Phantom or deafferentation pain is a variant of neuropathic pain associated with central nervous system dysfunction following disruption of peripheral sensory input. Phantom pain can occur long after denervation of affected areas and may persist chronically or even permanently. Phantom pain may be seen following traumatic or surgical amputation and has even been described after dental extraction.46 The pathophysiology of phantom pain is complex and incompletely understood.
Patient assessment
Recognition and treatment of pain and agitation can be particularly challenging in the absence of reliable objective quantitative assessments on which to base clinical decisions. Patient descriptions can guide analgesic and sedative interventions. However, even verbal children may find it difficult to express this information, particularly in the setting of critical illness. Children may be nonverbal or otherwise incommunicative secondary to age, developmental immaturity, medical illness, surgical procedures, or interventions such as intubation. Caregivers must recognize patient behaviors or behavioral patterns that provide clues to the presence, location, severity, and even cause of pain and agitation, and must monitor changes to guide therapy. No single objective assessment strategy will be sufficient or appropriate for every patient in every setting.31
Caregiver fears and beliefs may also hinder adequate analgesia and sedation. Caregivers may fear providing unnecessary analgesia or sedation, particularly controlled substances. They may misinterpret regulations and legal requirements associated with these medications, or may fear promoting patient addiction. In terminal or palliative care settings, caregivers may fear hastening or even causing death. These issues must be addressed within the healthcare team (see Chapter 3).
Assessment Tools
Subjective Patient Report
Assessment tools relying on a subjective patient report ask the patient to indicate status on a continuum. Pain scales commonly range from 0 (no pain) to 10 (maximum possible pain); sedation scales vary more widely. Verbal children who are able to count may simply be asked to indicate their pain score on a 0-10 scale. Interactive but nonverbal children, or children unable to count, may be asked to indicate their pain score on a continuum scale using colors, pictures of children, or drawings of faces that represent degrees of distress. Three such pain assessment tools commonly used in pediatric practice are the Oucher! (see Evolve Fig. 5-1 in the Chapter 5 Supplement on the Evolve Website), the McGrath Faces Pain Scale, and the Hicks Faces Pain Scale-Revised (Fig. 5-3). See Patient Assessment in the Chapter 5 Supplement on the Evolve Website.
Objective Caregiver Evaluation
Many assessment tools for objective caregiver evaluation of pain and agitation have been developed for and validated in the pediatric setting. Examples include the FLACC scale (characterizing the patient’s Face, Legs, Activity, Cry, Consolability using specific options) for assessment of pain in nonverbal children (Table 5-2), the COMFORT scale (assessing overall comfort by evaluating specific physical characteristics) for assessment of agitation in critically ill children, and the State Behavioral Scale for assessment of agitation in infants and children during mechanical ventilation.19 Examples of these tools may be found in Evolve Fig. 5-2 in the Chapter 5 Supplement on the Evolve Website. Additional tools have been reported for use in specific patient populations or particular clinical settings.50
Management Planning
With appropriate intervention, most critically ill children should experience little pain and minimal distress. Following comprehensive patient assessment, an appropriate management plan is developed to address patient pain and agitation.48 The goals of any management plan should be to maximize analgesia and sedation, minimize side effects and complications, and if possible aid in diagnosis and treatment of underlying critical illness. Ideally, all caregivers should be involved in development of this plan and should be aware of planned interventions. During and after interventions, caregivers must perform regular and periodic patient reassessment to evaluate patient response and guide further interventions. Management must be individualized for each patient, combining nonpharmacologic and pharmacologic interventions as appropriate.
Nonpharmacologic interventions
Cognitive and Behavioral Modalities
Music Therapy
Music therapy can provide both distraction and relaxation in children. It has been used effectively in the operating room, postanesthesia care unit, neonatal critical care unit,4,32 and oncology ward. Music choice should be based on patient age, culture, and preference, guided as necessary by parents, other family members, and friends. Listening to music through headphones offers the added benefit of masking chaotic auditory stimuli.
Systemic analgesics
Numerous systemic analgesics are available for use in children (Table 5-3). Systemic analgesics can have a wide range of physiologic effects, particularly in critically ill children. Knowledge of the agents being administered is essential for optimal efficacy and patient safety. Nurses must be aware of expected clinical effect, usual time of onset, likely duration, and potential side effects of each agent administered. Medications ordered on an as-needed basis are effective only when given appropriately with ongoing and recurring patient assessment. Frequent requirement for a drug ordered only on an as needed basis should prompt consideration of scheduled administration and additional interventions. Intramuscular injection is painful and absorption variable; it should be avoided except perhaps in the setting of difficult intravenous access. Although many systemic analgesics can produce sedation, they should not be used primarily for this purpose.
Drug | Dose | Comments |
Acetaminophen | ||
Acetaminophen and NSAIDs are generally synergistic and may be given together without need to alternate or stagger doses. | ||
Acetaminophen | Load: 20 mg/kg PO (maximum 1000 mg), then | Good antipyretic; hepatic toxicity with overdose |
Main: 15 mg/kg PO (maximum 1000 mg) q4-6 h | Loading dose especially useful for procedural or perioperative analgesia | |
Load: 40 mg/kg PR (maximum 1300 mg), then | FDA now advises maximum single adult dose of 650 mg for over-the-counter use | |
Main: 20 mg/kg PR (maximum 1300 mg) q4-6 h | ||
Maximum 4 g/24 h PO/PR | ||
Nonsteroidal Antiinflammatory Drugs (NSAIDs) | ||
Acetaminophen and NSAIDs are generally synergistic, and may be given together without the need to alternate or stagger doses. | ||
Choline magnesium trisalicylate | 10 mg/kg PO/PR (maximum 1500 mg) q6-8 h | Only NSAID without platelet dysfunction |
Maximum 4 g/24 h PO/PR | No association with Reye syndrome | |
Ibuprofen | 10 mg/kg PO/PR (maximum 800 mg) q6-8 h | Good antipyretic (IV dose: 5 mg/kg may be used for antipyretic) |
IV: 10 mg/kg (for pain) | ||
Maximum 3200 mg/24 h PO/PR | IV formula recently approved analgesic in adults | |
Ketorolac | 0.5 mg/kg IM/IV (maximum 30 mg) q6 h | Potentially significant platelet dysfunction |
Total therapy must be <5 days | ||
Lower Potency Oral Opioids | ||
Recommended doses are for initial administration in opioid-naïve patients: titration to clinical effect is required; recommended initial opioid doses should typically be reduced 35% to 50% in neonates and young infants. | ||
Codeine | 1 mg/kg PO q4 h (adult dose, 30-60 mg) | Tablet and liquid preparations typically in combination with acetaminophen |
High incidence of gastrointestinal side effects | ||
Hydrocodone | 0.2 mg/kg PO q4 h (adult dose, 10-15 mg) | Tablet and liquid preparations typically in combination with acetaminophen or NSAID |
Moderate incidence of gastrointestinal side effects | ||
Sustained-release product available as antitussive, under study as analgesic | ||
Oxycodone | 0.1 mg/kg PO q4 h (adult dose, 5-10 mg) | Tablet preparations with or without acetaminophen or NSAID |
Liquid preparations contain only oxycodone | ||
Low incidence of gastrointestinal side effects | ||
Sustained-release product available for chronic therapy | ||
Higher Potency Opioids | ||
Recommended doses are for initial administration in opioid-naive patients: titration to clinical effect is required; recommended initial opioid doses should typically be reduced 25% to 50% in neonates and young infants; PCA demand dose typically q 8-10 min for patient-controlled analgesia, q 15-60 min for nurse or parent-controlled analgesia. | ||
Fentanyl | 5-15 mcg/kg PO (adult dose 400 mcg) | Dosing interval for oral preparation not well defined |
0.5-2 mcg/kg IV (adult dose 100 mcg) q 1 h | ||
Infusion 0.5-2 mcg/kg per hour (adult dose 100 mcg/h) | Rapid IV infusion may cause chest wall rigidity in infants | |
Patch 25 mcg = 1 mg/h IV morphine | ||
PCA demand dose 0.5-1 mcg/kg IV (adult dose 50-100 mcg) | Tachyphylaxis common | |
PCA basal 0.5-1 mcg/kg per hour IV (adult dose 50-100 mcg/h) | Transdermal patch not for acute management | |
Hydromorphone | 20-40 mcg/kg PO (adult dose 2-4 mg) q 3 h | Sustained-release oral product under study as analgesic |
10-20 mcg/kg IM/IV/SC (adult dose 1-2 mg) q 3 h | ||
Infusion 4 mcg/kg per h (adult dose 0.2-0.3 mg/h) | Less histamine release than morphine | |
PCA demand dose 4 mcg/kg IV (adult dose 0.2-0.3 mg) | ||
PCA basal 4 mcg/kg per hour IV (adult dose 0.2-0.3 mg/h) | ||
Meperidine | 0.25-0.5 mg/kg IM/IV/SC (adult dose 12.5-25 mg) | Doses for treatment of shivering |
Infusion/PCA not recommended | Neurotoxic metabolite may induce seizures | |
No hepatobiliary advantage over any other opioid | ||
No longer recommended as primary analgesic | ||
Methadone | 0.1 mg/kg PO (adult dose 5-10 mg) q6-12 h | Useful for chronic therapy Treatment of addiction must be in federally licensed facility |
0.05 mg/kg IV (adult dose 2.5-5 mg) q6-12 h | ||
Infusion/PCA not generally used | ||
Morphine | 0.3 mg/kg PO (adult dose 15-30 mg) q3 h | Sustained-release oral product available for chronic therapy |
0.05-0.1 mg/kg IM/IV/SC (adult dose: 5-10 mg) q3 h | ||
Infusion 0.02 mg/kg per h (adult dose 1-1.5 mg/h) | Potentially significant histamine release | |
PCA demand dose, 0.02 mg/kg IV (adult dose 1-1.5 mg) | ||
PCA basal 0.02 mg/kg per hour IV (adult dose 1-1.5 mg/h) |
FDA, U.S. Food and Drug Administration; h, hour; IM, intramuscular; IV, intravenous; Load, loading dose; Main, maintenance dose; mcg, microgram; PCA, patient-controlled analgesia; PO, by mouth; PR, by rectum; q, every; SC, subcutaneous.
Nonopioid Analgesics
Acetaminophen
Acetaminophen is widely used as an analgesic and an antipyretic. It can provide complete analgesia for mild to moderate pain and may reduce opioid requirement in moderate to severe pain, particularly when given on a scheduled basis. Acetaminophen is not an NSAID. Although it is a cyclooxygenase inhibitor, it has virtually no antiinflammatory activity, and therefore has few gastrointestinal, renal, or hematologic side effects. The primary toxicity of acetaminophen is hepatic, seen with both acute and chronic overdose, although renal toxicity has been described.42
Acetaminophen is at least as effective an analgesic as codeine,12 and it is synergistic with NSAIDs.52 Acetaminophen and NSAIDs can be given simultaneously without need to alternate or stagger doses. As with most nonopioid analgesics, acetaminophen demonstrates a ceiling effect: exceeding recommended doses does not significantly improve analgesia and increases risk of side effects and toxicity. Recently, an FDA advisory committee recommended the maximum single adult dose for over-the-counter products be reduced to 650 mg because of risk of hepatic injury.24
Nonsteroidal Antiinflammatory Drugs
The most widely used oral NSAID in children in the United States is ibuprofen, available in a variety of liquid, tablet, and capsule preparations. Intravenous ibuprofen, like intravenous indomethacin, is approved only for medical closure of patent ductus arteriosus in infants. Ibuprofen is a moderate potency analgesic and excellent antipyretic with an impressive pediatric safety record; it is underused for procedural and perioperative pain management in children. Ibuprofen is at least as effective an analgesic as acetaminophen, and it is superior to codeine.12 Ibuprofen and acetaminophen are synergistic.52 The liquid preparation can be given rectally at similar doses to patients unwilling or unable to tolerate oral doses.
Significant platelet dysfunction can develop after a single dose of ketorolac, and its use in patients with risk for bleeding is controversial. Although initial experience suggested greater blood loss during tonsillectomy in children receiving perioperative ketorolac, prospective randomized trials showed only nonsignificant trends toward increased bleeding without clinical complications.51a It may be prudent to avoid ketorolac in patients at risk for bleeding until more definitive information is available.
Opioid Analgesics
Opioids remain the mainstay of pharmacologic analgesia in patients of all ages. Acting on opioid receptors in the central nervous system and elsewhere, opioids cause dose-dependent analgesia and respiratory depression. Other side effects include somnolence, pupil constriction, decreased gastrointestinal motility, nausea, and urinary retention. Many opioids induce histamine release, causing urticaria, pruritus, nausea, bronchospasm, and occasionally hypotension. Pruritus is more common and typically more intense with neuraxial administration, likely caused by central nervous system opioid effect rather than histamine release. Opioid side effects can be managed with a variety of agents (Table 5-4).
The opioid antagonist naloxone rapidly reverses opioid effects. Naloxone may precipitate withdrawal in opioid-dependent patients, and pulmonary edema has been reported with higher doses. Low-dose naloxone infusion has been described for prevention and treatment of side effects secondary to ongoing opioid administration.41 For treatment of opioid overdose, support of effective ventilation before naloxone administration may prevent excessive adrenergic response.
Oral Opioids
Methadone has prolonged onset and duration of action, making it poorly suited for management of acute or dynamic pain, but well suited for chronic therapy in patients with stable analgesic requirements. Use of methadone as an analgesic, or for weaning in the setting of tolerance or dependence, is appropriate and legal. Use of methadone for treatment of psychopathologic addiction is restricted to federally licensed facilities. Although methadone may cause Q-T prolongation and ventricular arrhythmias, particularly at higher doses, recent suggestions to consider screening electrocardiogram before and at intervals during methadone therapy35,36 remain controversial.27
Fentanyl is available for oral administration as a lozenge attached to a stick, the so-called fentanyl lollipop. Oral fentanyl has been used for pre-anesthetic sedation in children, although it is no longer marketed for this indication. Oral fentanyl has more recently been used to provide analgesia and sedation for painful procedures, and for patients with breakthrough acute and chronic pain.5 Nausea and emesis are fairly common. Intranasal fentanyl is increasingly used for management of acute pain in the emergency department9 and for treatment of breakthrough pain in opioid-dependent oncology patients.37 Onset of action is rapid with either route: analgesia and respiratory depression may develop quickly.
Patient-Controlled Analgesia (PCA)
If an opioid is administered only after a patient reports pain, this can create a vicious cycle of suffering, a delay in drug administration, and resultant persistent pain or excessive sedation. Optimal opioid administration provides pain relief, but avoids respiratory depression or excessive sedation, preserving a balance between desired efficacy and undesired side effects (Fig. 5-4). Intermittent dosing is time-consuming for providers and frequently upsets this analgesic balance. Continuous infusion eventually establishes a drug level steady state, but is independent of patient request. A safe, effective, and readily titratable modality for ongoing opioid administration in children is PCA. PCA modalities maximize analgesia, minimize side effects, and reduce overall opioid consumption in patients of all ages.
Drug delivery in PCA is controlled by a microprocessor providing dose administration in response to patient or proxy request, usually with the press of a button. Appropriate lockout intervals and maximum doses are programmed to prevent overdose. Analgesia is generally excellent. With adequate instruction, most developmentally appropriate school-aged children can safely and effectively manage their own PCA. Nurse- or parent-controlled PCA, so-called PCA by proxy, can be used for children who are unable or unwilling to control their own pump, although the risk of respiratory depression increases if dosing intervals are not adjusted, particularly in combination with basal infusions. Prevalence of complications during PCA by proxy is similar to that during conventional PCA, although serious complications may be more common.60
Local anesthetics
Local anesthetics impair nerve conduction through the blockade of nerve cell sodium channels. Local anesthetics are potentially neurotoxic, altering level of consciousness or inducing seizures if therapeutic levels are exceeded. Local anesthetics are also potentially cardiotoxic and may induce arrhythmias including ventricular tachycardia, ventricular fibrillation, and cardiac arrest. Prolonged resuscitation after local anesthetic overdose may be required, particularly with long-acting agents. Recent reports suggest that administration of intravenous lipid emulsion increases the likelihood of successful resuscitation after local anesthetic toxicity, perhaps by serving as a binding reservoir.25
Topical Application
Many local anesthetics are formulated to provide cutaneous analgesia without the need for potentially painful injections (Table 5-5). Such preparations enhance usefulness of topical anesthetics for minor procedures, and in many instances they reduce or eliminate the need for supplementary systemic analgesia and sedation. The use of topical formulas is limited primarily by cost and time of onset. Specific agents are discussed in Local Anesthetics, Topical Application in the Chapter 5 Supplement on the Evolve Website.
Product/Compound | Ingredients | Comments |
Lidocaine cream or gel | Liposomal lidocaine 3%, 4% or 5% | Apply for 30 min |
No dressing required | ||
Nonprescription | ||
EMLA (generic): Eutectic mixture of local anesthetics | Lidocaine 2.5% + prilocaine 2.5% | Apply for 1-4 h |
Requires occlusive dressing | ||
Prilocaine may cause methemoglobinemia | ||
TAC: tetracaine, adrenaline, cocaine | Tetracaine 0.5-1% + adrenaline (epinephrine 1:2000-4000) + Cocaine 4-11.8% | Apply for 15-20 min |
Avoid mucous membranes | ||
Avoid terminally perfused areas | ||
Potential cocaine toxicity | ||
LET: lidocaine, epinephrine, tetracaine | Lidocaine 4% + epinephrine 1:2000 + tetracaine 0.5% | Contains lidocaine rather than cocaine |
Apply for 15-20 min | ||
Avoid mucous membranes | ||
Avoid terminally perfused areas | ||
Synera | 70 mg lidocaine/70 mg tetracaine in a topical patch | FDA-approved for children 3 years of age and older |
Apply for 20-30 min | ||
Erythema common; monitor for allergic reaction |
Regional Anesthetic Techniques
Peripheral Nerve Blocks
Virtually any peripheral nerve can be blocked with appropriate equipment and sufficient provider interest. Typically, a small volume of local anesthetic is injected adjacent to the appropriate nerve or nerves to provide anesthesia in the desired distribution. For additional information about these blocks, please see Regional Anesthetic Techniques in the Chapter 5 Supplement on the Evolve Website.
Plexus Blocks
Pediatric providers are gaining experience with plexus blocks, including brachial plexus,21 lumbosacral, and paravertebral blocks. Intravenous regional anesthesia of the extremities, or Bier block, has been described in children, but widespread use has been limited by the theoretical risk of local anesthetic toxicity. Although rare in clinical practice, systemic local anesthetic toxicity may warrant observation in the critical care setting given risk for neurologic and cardiac compromise. Plexus blocks are discussed in greater detail in Regional Anesthetic Techniques in the Chapter 5 Supplement on the Evolve Website.
Neuraxial Blocks
Excellent analgesia may be provided by repeated injection or continuous infusion of anesthetic through indwelling epidural catheters. Epidural catheters can be inserted caudally and threaded to the desired vertebral level, particularly in infants and young children. Epidural catheters can also be placed directly at the desired vertebral level. As with other invasive access, skin preparation with chlorhexidine rather than iodine confers a lower risk of subsequent epidural catheter colonization. Many combinations of local anesthetic, opioid, and adjuvant agents are commonly used for epidural administration in the United States (Table 5-6). Placement of epidural catheters and selection of agents for epidural injection or infusion are discussed in greater detail in the Chapter 5 Supplement on the Evolve Website.
Local Anesthetic* | Opioid/Adjuvant | Infusion Rates |
Bupivacaine 0.0625-0.1% (maximum dose 0.4 mg/kg per h) | Fentanyl 0.5-1 mcg/kg per h (adult dose 50-100 mcg/h) | Thoracic typically 0.2-0.3 mL/kg per h (maximum 5-10 mL/h) |
-OR- | -OR- | |
Levobupivacaine 0.0625-0.1% (maximum dose 0.4 mg/kg per h) | Hydromorphone 2-4 mcg/kg per h (adult dose 150-300 mcg/h) | Lumbar typically 0.3-0.4 mL/kg per h (maximum 10-15 mL/h) |
-OR- | -OR- | |
Lidocaine 0.1-0.5% (maximum dose 3 mg/kg per h) | Morphine 3-6 mcg/kg per h (adult dose 250-500 mcg/h) | Caudal typically 0.4-0.5 mL/kg per h (maximum 15-20 mL/h) |
-OR- | -AND/OR- | |
Ropivacaine 0.1-0.2% (maximum dose 0.5 mg/kg per h) | Clonidine 0.1-0.5 mcg/kg per h (adult dose 25-50 mcg/h) |
* Recommended local anesthetic and initial opioid doses should typically be reduced 25-50% in neonates and young infants.
Systemic sedatives
Because of the variability of individual responses, particularly in children, administration of systemic sedatives with intent to provide light or moderate sedation can rapidly and unexpectedly induce deep sedation or general anesthesia. All sedation depresses level of consciousness and increases risk of airway obstruction, respiratory depression, aspiration, and cardiovascular depression; any of these complications can produce significant morbidity and potential mortality. Because children are particularly prone to such complications, nurses caring for children receiving systemic sedatives must be extremely vigilant.1–3,16,17
Sedative-Hypnotic Agents
Systemic sedatives produce a wide range of physiologic effects. Knowledge of the agents being administered is essential for optimal efficacy and greatest patient safety. Nurses must be aware of expected clinical effect, usual time of onset, likely duration, and potential side effects of each agent. Medications ordered on an “as needed” basis are effective only when given appropriately in response to ongoing and recurring patient assessment. If a drug ordered on an “as needed” (PRN) basis is required frequently, the healthcare team should consider a change to scheduled administration and other interventions to improve patient comfort. Intramuscular injection is painful and should be avoided if possible, except perhaps in the setting of difficult intravenous access. Most systemic sedatives lack analgesic efficacy and should not be used primarily for analgesia. Many sedative-hypnotic agents are used in children; selection is determined by anticipated depth and duration of sedation desired, risk of potential side effects, and available routes of administration (Table 5-7).
Drug | Indication | Dose |
Chloral hydrate | Procedural sedation | 25-100 mg/kg PO/PR (maximum 2 g) |
Pentobarbital | Procedural sedation | 1-2 mg/kg IV (adult dose 50-100 mg) initial dose; |
0.25-0.5 mg/kg IV (adult dose 25-50 mg) q5-10 min | ||
Ongoing sedation | 1-2 mg/kg IV (adult dose 50-100 mg) q1-2 h | |
Infusion | 1-2 mg/kg per h IV (adult dose 50-100 mg/h) | |
Midazolam | Premedication | 0.25-0.5 mg/kg PO/PR (maximum 20 mg) |
0.2-0.4 mg/kg SL/nasally (maximum 10 mg) | ||
Procedural sedation | 0.05-0.1 mg/kg IV (adult dose 5 mg) initial dose; | |
0.025-0.05 mg/kg IV (adult dose 2 mg) q5-10 min | ||
Ongoing sedation | 0.05-0.1 mg/kg IV (adult dose 2-5 mg) q1-2 h | |
Infusion | 0.05-0.1 mg/kg per h IV (adult dose 2-5 mg/h) | |
Ketamine | Procedural sedation | 4-10 mg/kg PO (adult dose 300-500 mg) |
3-4 mg/kg IM (150-300 mg) | ||
0.5-1 mg/kg IV (adult dose 50-100 mg) | ||
Ongoing sedation | 0.5-1 mg/kg IV (adult dose 50-100 mg) q1-2 h | |
Propofol | Procedural sedation | 0.5-2 mg/kg IV (adult dose 50-100 mg) initial dose; |
0.5 mg/kg IV (adult dose 25-50 mg) q5-10 min | ||
Infusion | 50-150 mcg/kg per min IV | |
Dexmedetomidine | Premedication | 1-2 mcg/kg nasally |
Procedural sedation | 1-2 mcg/kg IV over 10 min | |
Infusion | 0.2-1 mcg/kg per h IV |
Recommended doses are for initial administration: titration to clinical effect is required. Appropriate analgesia should be provided in settings entailing significant pain.
IM, Intramuscular; IV, intravenous; PO, by mouth; PR, by rectum; q, every; SL, sublingual.
Benzodiazepines
Many other benzodiazepines are available, although relatively few are commonly used in children. Lorazepam has a longer time of onset than midazolam, and it is less likely to induce acute respiratory depression. Lorazepam also has a longer duration of action than midazolam and may be useful when longer-term sedation is desired. Lorazepam is also widely used as an acute anticonvulsant. Diazepam has even slower time of onset and longer duration of action, and it can be useful for maintenance therapy in patients with significant benzodiazepine tolerance or when ongoing prolonged sedation is desired.34 Commercial preparations of lorazepam and diazepam contain propylene glycol as a solvent and benzyl alcohol as a preservative. Higher or repetitive doses or continuous infusion should be administered with caution to neonates and other patients susceptible to benzyl alcohol toxicity. Although significant propylene glycol accumulation has been documented in critically ill children receiving lorazepam infusion, other laboratory abnormalities were not noted. Peripheral intravenous administration of diazepam can cause significant discomfort and chemical phlebitis; intramuscular administration is not recommended.
Ketamine
Ketamine is most commonly administered intravenously to induce general anesthesia in medically fragile infants and children, and intramuscularly in patients who are unable to cooperate or who have no reliable intravenous access. Ketamine is used as an analgesic for children with burns44 or receiving palliative care13 and when other analgesics prove inadequate. PCA with ketamine has been described.62 Ketamine is widely used for procedural sedation in children and has become particularly popular in pediatric emergency departments given its favorable safety profile. Continuous infusion may be useful if repeated dosing becomes necessary or if a need for ongoing sedation is anticipated. Emergence may be prolonged after oral administration, particularly with a higher dose, and emergence delirium is common. Although an anticholinergic agent such as atropine or glycopyrrolate can be given to prevent excessive salivation, the effectiveness of this practice has been questioned, as has concomitant administration of benzodiazepines to decrease the likelihood of hallucinations and delirium.28
Propofol
Propofol is a sedative-hypnotic agent without specific analgesic effect; it is commonly used for induction and maintenance of sedation and anesthesia. Chemically unrelated to other systemic sedatives, its mechanisms of action are unknown, although propofol likely has significant GABA agonism. Propofol is available only for intravenous administration. It is supplied in a lipid emulsion with an alkaline pH and may cause significant pain on injection. Propofol has considerable antiemetic efficacy,23 and like ketamine it promotes bronchodilation. Propofol has an extremely rapid onset of action, and with relatively prompt hepatic conjugation and renal excretion it allows for rapid recovery after intermittent dosing or brief infusion. Propofol is also highly lipophilic, leading to significant tissue accumulation and potentially prolonged emergence after repeated administration or prolonged infusion. Because propofol lacks analgesic efficacy, appropriate analgesia should be provided for significant pain.
Prolonged propofol infusion is associated with a rare but often fatal syndrome of metabolic acidosis and cardiovascular failure. Current recommendations suggest propofol infusion in children not exceed 48 hours, although death from apparent propofol infusion syndrome has been reported with shorter duration or with reexposure to the drug. The etiology of this life-threatening reaction to propofol infusion in some patients is unknown, although impairment of mitochondrial oxidative phosphorylation and altered lipid metabolism have been suggested.26
Propofol induces dose-dependent sedation, loss of airway reflexes, hypoventilation, apnea, and cardiovascular depression. Higher doses of propofol induce general anesthesia. Although there has been considerable concern over the safety of propofol administration by nonanesthesiologists,39 current evidence suggests that such use is safe under controlled circumstances.18 Propofol has been safely and successfully used for pediatric procedural sedation in numerous settings, including the pediatric critical care unit, burn unit, radiology suite, emergency department, ambulatory procedure center, and dental clinic. Although such success is impressive, patient safety was protected by the restriction of propofol use to appropriately trained personnel working in the context of a dedicated sedation team, following carefully designed protocols and adhering to appropriate standards for patient monitoring and management. Propofol infusion may be used in the critical care setting during weaning from mechanical ventilation or for rescue sedation when other agents prove inadequate.59
Dexmedetomidine
Dexmedetomidine is a newer sedative-hypnotic agent that is becoming popular in pediatric practice,18 although the drug is formally approved only for use in adults. As an alpha (α)-2 adrenergic agonist similar to clonidine, dexmedetomidine induces potentially profound sedation with little associated respiratory depression. Dexmedetomidine can cause significant bradycardia and hypotension, particularly at high dose or with rapid administration. Heart rate and blood pressure during anesthesia with dexmedetomidine infusion tend to be lower and recovery more prolonged than during anesthesia with propofol infusion.30
Dexmedetomidine is marketed for intravenous use, although the commercially available form can be given nasally, and subcutaneous administration in children has been described.57 Nasal dexmedetomidine lacks unpleasant taste or odor, it provides preoperative sedation comparable to56 or greater than64 oral midazolam, and it may enhance postoperative analgesia.53
Dexmedetomidine is often used for pediatric procedural and perioperative sedation45 and for weaning mechanical ventilation.11,20 Dexmedetomidine is also used to treat postoperative shivering in children8 and to facilitate weaning from opioids58 and benzodiazepines. Although officially approved for use up to 24 hours, prolonged dexmedetomidine infusion for days or even weeks for sedation of critically ill infants and children,51 including pediatric cardiothoracic surgical patients,7 has been described. Dexmedetomidine provides little direct analgesia and is useful as a sole agent only when significant analgesia is not required.6 Appropriate analgesia should be provided when significant pain is present.45
Procedural Sedation
Diagnostic and therapeutic procedures requiring sedation in children have increased dramatically in recent years. Although anesthesiologists provide care for many such interventions, procedural sedation for children is also administered by other healthcare providers, including those in the pediatric critical care setting.18,38
The American Academy of Pediatrics has established guidelines for the monitoring and management of children undergoing procedural sedation,1–3 and different guidelines have been published by other organizations.16 This has created considerable debate, occasional confusion, and some inconsistency, but all guidelines share the common goal of fostering safe and efficient practice.
To minimize the risk of aspiration of gastric contents, oral intake should be suspended before any elective procedure for which sedation is planned, as for any elective procedure entailing anesthesia (Table 5-8). If sedation must be provided despite recent oral intake, urgency of the intervention should be documented and systemic sedatives administered cautiously to minimize depression of airway reflexes and risk of aspiration. Agents such as metoclopramide, ranitidine, and sodium bicitrate may be considered to augment gastric motility, decrease gastric volume, and increase gastric pH. When appropriate fasting intervals cannot be observed and deep sedation is anticipated, airway protection with induction of anesthesia and rapid sequence intubation may be advisable.
Table 5-8 Recommended Fasting Intervals Before Elective Sedation or Anesthesia
Type of Intake* | Recommended Fasting Interval (h) |
Clear liquids, including water, juice without pulp, carbonated beverages, clear tea, black coffee | 2 |
Human breast milk | 4 |
Nonhuman milk, infant formula, light meal without significant protein or fat content | 6 |
Heavy meal with significant protein or fat content, including particularly fried foods or meat | 8 |
* These recommendations apply regardless of patient age.
Adapted from American Society of Anesthesiologists Task Force on Preoperative Fasting: Practice guidelines for preoperative fasting and the use of pharmacologic agents to reduce the risk of pulmonary aspiration: application to healthy patients undergoing elective procedures: a report by the American Society of Anesthesiologists Task Force on Preoperative Fasting. Anesthesiology 90:896–905, 1999.
Neuromuscular blockade
Neuromuscular blockade, or muscle relaxation, entails pharmacologic inhibition of voluntary muscle movement. Description as paralysis should be avoided because of negative connotations for patients and families. Neuromuscular blockade has no effect on level of consciousness and provides neither analgesia nor sedation. Neuromuscular blockade should be used only when clinically essential,14,29 and never in isolation. Neuromuscular blockade should never be used simply to keep patients from moving. Appropriate airway and ventilatory support are mandatory. Neuromuscular blockade may be used to facilitate endotracheal intubation, provide surgical relaxation, and allow mechanical ventilation. Prolonged neuromuscular blockade may be necessary in some settings, as after surgical airway reconstruction.29
Normal neuromuscular function relies on the release of acetylcholine from motor nerve terminals at the neuromuscular junction (Fig. 5-5). Acetylcholine crosses the synaptic cleft between motor nerve and skeletal muscle fiber, transiently binding to and briefly opening nicotinic acetylcholine receptors on the myocyte motor end plate. The resultant transmembrane flow of ions, primarily sodium, potassium, and calcium depolarizes the surrounding muscle cell membrane, eventually causing myocyte contraction if an appropriate action potential is achieved. Acetylcholine is subsequently metabolized by acetylcholinesterase within the junctional folds.
Neuromuscular Blocking Agents
Neuromuscular blockade is achieved through blockade of nicotinic acetylcholine receptors at the neuromuscular junction. Neuromuscular blocking agents are classified according to their mechanism of action as depolarizing or nondepolarizing agents (Table 5-9). Depolarizing agents activate nicotinic acetylcholine receptors, inducing myocyte depolarization. As long as they persist at the neuromuscular junction, they prevent any further myocyte depolarization, thereby maintaining muscle relaxation. Nondepolarizing agents compete directly with acetylcholine for binding sites on nicotinic acetylcholine receptors, preventing binding of acetylcholine without activating the receptor. Neuromuscular blockade persists as long as sufficient nondepolarizing agent remains to prevent acetylcholine receptor activation. Nondepolarizing agents are eliminated by a wide range of mechanisms. Younger patients, particularly infants, are generally relatively resistant to depolarizing agents but relatively sensitive to nondepolarizing agents.
Nondepolarizing Agents
Neuromuscular blockade can be maintained with repeated dosing or continuous infusion of any agent.10 Repeated dosing typically requires 25% to 50% of the usual initial dose, whereas continuous infusion typically requires 25% to 50% of the usual initial dose per hour, depending on drug pharmacology and underlying patient disease. Whenever neuromuscular blockade is maintained through repeated dosing or continuous infusion, nurses should monitor the degree of neuromuscular blockade (see section, Neuromuscular Monitoring) to prevent unnecessary and excessive dosing.15,49 Neuromuscular monitoring is discussed in greater detail in the Chapter 5 Supplement on the Evolve Website.
Reversal of Neuromuscular Blockade
Sugammadex is a recently developed gamma (γ)-cyclodextrin capable of binding an aminosteroid nondepolarizing agent. The sugammadex molecule encapsulates or traps the aminosteroid compound within a central pore, and the resultant complex is renally excreted.55 Initial experience with sugammadex has been encouraging,43 although pediatric experience is limited47 and the drug is not yet approved for use in the United States. Preliminary data suggest that this agent may increase the safety of longer acting, nondepolarizing neuromuscular blockers.
Critical Illness Polyneuropathy and Myopathy
Prolonged muscle weakness after pharmacologic neuromuscular blockade in critically ill patients is common, affecting many adults requiring mechanical ventilation.63 Although a similar syndrome of prolonged muscle weakness following critical illness in children is increasingly recognized, particularly after pharmacologic neuromuscular blockade,33 the condition is thought to be much less common in the pediatric setting, reportedly affecting approximately 1% of critically ill children. The condition has recently been termed critical illness polyneuropathy and myopathy, and it is increasingly recognized in pediatric patients.63
Critical illness, particularly sepsis, is associated with prolonged subsequent muscle weakness, as well as with immobility and muscle atrophy. Many drugs, particularly aminoglycoside antibiotics and high-dose steroids, are known to cause a predisposition to prolonged muscle weakness. Many other agents, including diuretics such as furosemide and sedatives such as benzodiazepines, may also contribute. Pharmacologic neuromuscular blockade is highly associated with subsequent prolonged muscle weakness, particularly after prolonged blockade, and the risk appears greater with aminosteroid compounds.10 Concomitant administration of multiple agents predisposing to prolonged muscle weakness likely heightens risk. The etiology of critical illness polyneuropathy and myopathy is likely multifactorial.
Critical illness polyneuropathy and myopathy can vary in clinical presentation from mild diffuse muscle weakness to profound flaccid quadriplegia with respiratory failure. The condition appears to be associated with neuromuscular pathology, including muscle atrophy with denervation and axonal neuropathy, even in the absence of significant inflammation. Clinical weakness may persist for months or years, with neurodiagnostic abnormalities lasting even longer.61 Pediatric patients generally seem to have a somewhat more favorable prognosis than do adults, although recovery is less likely in very young patients, particularly neonates and premature infants in whom neuromuscular development is still incomplete.
Neuromuscular Monitoring
During repeated dosing or continuous infusion of any neuromuscular blocking agent, providers should consider neuromuscular monitoring to prevent unnecessary and excessive dosing.49 Neuromuscular monitoring can be challenging in many patients,15 particularly small infants, and findings can be influenced by factors such as peripheral edema, level of hydration, electrolyte and acid-base balance, and hemodynamic status. Ideally, providers should evaluate response to “train-of-four” stimulation (i.e., response to provision of 4 pulses in sequence from a nerve stimulator)49 at least once every 24 hours. If monitoring proves unreliable and patient safety permits, providers may consider daily discontinuation of neuromuscular blockade to assess for respiratory effort and spontaneous movement. Neuromuscular monitoring is reviewed in more detail in the Chapter 5 Supplement on the Evolve Website. Ongoing physical rehabilitation services should be provided to all patients with ongoing critical illness, particularly those receiving pharmacologic neuromuscular blockade.
Summary
Neuromuscular blockade, although providing neither analgesia nor sedation, is at times necessary in critical care. Neuromuscular blockade should never be administered without first ensuring adequate analgesia and sedation, and then only when necessary. Care should be taken to minimize risk of subsequent prolonged neuromuscular weakness. For additional information about pharmacokinetics and pharmacodynamics in pediatric critical care, see Chapter 4.
1 American Academy of Pediatrics, American Academy on Pediatric Dentistry. Guideline for monitoring and management of pediatric patients during and after sedation for diagnostic and therapeutic procedures. Pediatr Dent. 2008–2009;30(7 Suppl.):143-159.
2 American Academy of Pediatrics, American Academy of Pediatric Dentistry, Coté C.J., Wilson S., Work Group on Sedation. Guidelines for monitoring and management of pediatric patients during and after sedation for diagnostic and therapeutic procedures: an update. Pediatrics. 2006;118(6):2587-2602.
3 American Academy of Pediatrics, American Academy of Pediatric Dentistry, Coté C.J., Wilson S., Work Group on Sedation. Guidelines for monitoring and management of pediatric patients during and after sedation for diagnostic and therapeutic procedures: an update. Paediatr Anaesth. 2008;18(1):9-10.
4 Arnon S., et al. Live music is beneficial to preterm infants in the neonatal intensive care unit environment. Birth. 2006;33(2):131-136.
5 Aronoff G.M., et al. Evidence-based oral transmucosal fentanyl citrate (OTFC) dosing guidelines. Pain Med. 2005;6(4):305-314.
6 Barton K.P., et al. Dexmedetomidine as the primary sedative during invasive procedures in infants and toddlers with congenital heart disease. Pediatr Crit Care Med. 2008;9(6):612-615.
7 Bejian S., et al. Prolonged use of dexmedetomidine in the paediatric cardiothoracic intensive care unit. Cardiol Young. 2009;19(1):98-104.
8 Blaine Easley R., Brady K.M., Tobias J.D. Dexmedetomidine for the treatment of postanesthesia shivering in children. Paediatr Anaesth. 2007;17(4):341-346.
9 Borland M.L., Clark L.J., Esson A. Comparative review of the clinical use of intranasal fentanyl versus morphine in a paediatric emergency department. Emerg Med Australas. 2008;20(6):515-520.
10 Burmester M., Mok Q. Randomised controlled trial comparing cisatracurium and vecuronium infusions in a paediatric intensive care unit. Intensive Care Med. 2005;31(5):686-692.
11 Carroll C.L., et al. Use of dexmedetomidine for sedation of children hospitalized in the intensive care unit. J Hosp Med. 2008;3(2):142-147.
12 Clark E., et al. A randomized, controlled trial of acetaminophen, ibuprofen, and codeine for acute pain relief in children with musculoskeletal trauma. Pediatrics. 2007;119(3):460-467.
13 Conway M., et al. Use of continuous intravenous ketamine for end-stage cancer pain in children. J Pediatr Oncol Nurs. 2009;26(2):100-106.
14 Cools F., Offringa M. Neuromuscular paralysis for newborn infants receiving mechanical ventilation. Cochrane Database Syst Rev. 2005;18(2):CD002773.
15 Corso L. Train-of-four results and observed muscle movement in children during continuous neuromuscular blockade. Crit Care Nurse. 2008;28(3):30-38.
16 Coté C.J. Round and round we go: sedation—what is it, who does it, and have we made things safer for children? Paediatr Anaesth. 2008;18(1):3-8.
17 Coté C.J. Strategies for preventing sedation accidents. Pediatr Ann. 2005;34(8):625-633.
18 Cravero J.P., et alPediatric Sedation Research Consortium. The incidence and nature of adverse events during pediatric sedation/anesthesia with propofol for procedures outside the operating room: a report from the Pediatric Sedation Research Consortium. Anesth Analg. 2009;108(3):795-804.
19 Curley M.A., et al. State Behavioral Scale: a sedation assessment instrument for infants and young children supported on mechanical ventilation. Pediatr Crit Care Med. 2006;7(2):107-114.
20 Czaja A.S., Zimmerman J.J. The use of dexmedetomidine in critically ill children. Pediatr Crit Care Med. 2009;10(3):381-386.
21 De José María B., et al. Ultrasound-guided supraclavicular vs infraclavicular brachial plexus blocks in children. Paediatr Anaesth. 2008;18(9):838-844.
22 Derbyshire S.W., Osborn J. Modeling pain circuits: how imaging may modify perception. Neuroimaging Clin N Am. 2007;17(4):485-493.
23 Erdem A.F., et al. Subhypnotic propofol infusion plus dexamethasone is more effective than dexamethasone alone for the prevention of vomiting in children after tonsillectomy. Paediatr Anaesth. 2008;18(9):878-883.
24 FDA Joint Advisory Committee Meeting; Drug Safety and Risk Management Committee, Anesthetic and Life Support Drugs Advisory Committee and Nonprescription Drugs Advisory Committee: Acetaminophen overdose and liver injury—background and options for reducing injury. 2009 June 29-30
25 Felice K., Schumann H. Intravenous lipid emulsion for local anesthetic toxicity: a review of the literature. J Med Toxicol. 2008;4(3):184-191.
26 Fudickar A., Bein B. Propofol infusion syndrome: update of clinical manifestation and pathophysiology. Minerva Anestesiol. 2009;75(5):339-344.
27 Gourevitch M.N. First do no harm… Reduction? Ann Intern Med. 2009;150(6):417-418. 17
28 Green S.M., et alEmergency Department Ketamine Meta-Analysis Study Group. Predictors of emesis and recovery agitation with emergency department ketamine sedation: an individual-patient data meta-analysis of 8,282 children. Ann Emerg Med. 2009;54(2):171-180. e1-4. Epub 2009 Jun 6
29 Hammer G.B. Sedation and analgesia in the pediatric Intensive Care Unit following laryngotracheal reconstruction. Otolaryngol Clin North Am. 2008;41(5):1023-1044. x-xi
30 Heard C., et al. A comparison of dexmedetomidine-midazolam with propofol for maintenance of anesthesia in children undergoing magnetic resonance imaging. Anesth Analg. 2008;107(6):1832-1839.
31 Herr K., et alAmerican Society for Pain Management Nursing. Pain assessment in the nonverbal patient: position statement with clinical practice recommendations. Pain Manag Nurs. 2006;7(2):44-52.
32 Hunter B.C., Sahler O.J. Music for very young ears. Birth. 2006;33(2):137-138.
33 Iodice F., et al. Acute quadriplegic myopathy in a 16-month-old child. Paediatr Anaesth. 2005;15(7):611-615.
34 Kost-Byerly S., et al. Perioperative anesthetic and analgesic management of newborn bladder exstrophy repair. J Pediatr Urol. 2008;4(4):280-285.
35 Krantz M.J., et al. QTc interval screening in methadone treatment: the CSAT consensus guideline. Ann Intern Med. 2008. Dec 1 [Epub ahead of print]
36 Krantz M.J., et al. QTc interval screening in methadone treatment. Ann Intern Med. 2009;150(6):387-395.
37 Kress H.G., et al. Efficacy and tolerability of intranasal fentanyl spray 50 to 200 microg for breakthrough pain in patients with cancer: a phase III, multinational, randomized, double-blind, placebo-controlled, crossover trial with a 10-month, open-label extension treatment period. Clin Ther. 2009;31(6):1177-1191.
38 Leroy P.L., Gorzeman M.P., Sury M.R. Procedural sedation and analgesia in children by non-anesthesiologists in an Emergency Department. Minerva Pediatr. 2009;61(2):193-215.
39 Litman R.S. Sedation by non-anesthesiologists. Curr Opin Anaesthesiol. 2005;18(3):263-264.
40 Martin A.L., et al. Anxiety sensitivity, fear of pain and pain-related disability in children and adolescents with chronic pain. Pain Res Manag. 2007;12(4):267-272.
41 Maxwell L.G., et al. The effects of a small-dose naloxone infusion on opioid-induced side effects and analgesia in children and adolescents treated with intravenous patient-controlled analgesia: a double-blind, prospective, randomized, controlled study. Anesth Analg. 2005;100(4):953-958.
42 Mazer M., Perrone J. Acetaminophen-induced nephrotoxicity: pathophysiology, clinical manifestations, and management. J Med Toxicol. 2008;4(1):2-6.
43 Mirakhur R.K. Sugammadex in clinical practice. Anaesthesia. 2009;64(Suppl. 1):45-54.
44 Owens V.F., et al. Ketamine: a safe and effective agent for painful procedures in the pediatric burn patient. J Burn Care Res. 2006;27(2):211-216.
45 Phan H., Nahata M.C. Clinical uses of dexmedetomidine in pediatric patients. Paediatr Drugs. 2008;10(1):49-69.
46 Pinto A., Balasubramaniam R., Arava-Parastatidis M. Neuropathic orofacial pain in children and adolescents. Pediatr Dent. 2008;30(6):510-515.
47 Plaud B., et al. Reversal of rocuronium-induced neuromuscular blockade with sugammadex in pediatric and adult surgical patients. Anesthesiology. 2009;110(2):284-294.
48 Playfor S., et al. Consensus guidelines on sedation and analgesia in critically ill children. Intensive Care Med. 2006;32(8):1125-1136.
49 Playfor S., et al. Consensus guidelines for sustained neuromuscular blockade in critically ill children. Paediatr Anaesth. 2007;17(9):881-887.
50 Razmus I., Wilson D. Current trends in the development of sedation/analgesia scales for the pediatric critical care patient. Pediatr Nurs. 2006;32(5):435-441.
51 Reiter P.D., Pietras M., Dobyns E.L. Prolonged dexmedetomidine infusions in critically ill infants and children. Indian Pediatr. 2009. Apr 1 [Epub ahead of print]
51a Romsing J., Ostergaard D., Walther-Larsen S., Valentin N. Analgesic efficacy and safety of preoperative versus postoperative ketorolac in paediatric tonsillectomy. Acta Anaesthesiol Scand. 1998;42:770-775.
52 Sarrell E.M., Wielunsky E., Cohen H.A. Antipyretic treatment in young children with fever: acetaminophen, ibuprofen, or both alternating in a randomized, double-blind study. Arch Pediatr Adolesc Med. 2006;160(2):197-202.
53 Schmidt A.P., et al. Effects of preanesthetic administration of midazolam, clonidine, or dexmedetomidine on postoperative pain and anxiety in children. Paediatr Anaesth. 2007;17(7):667-674.
54 Sorce L.R. Adverse responses: sedation, analgesia and neuromuscular blocking agents in critically ill children. Crit Care Nurs Clin North Am. 2005;17(4):441-450.
55 Sparr H.J., Booij L.H., Fuchs-Buder T. Sugammadex. New pharmacological concept for antagonizing rocuronium and vecuronium. Anaesthesist. 2009;58(1):66-80.
56 Talon M.D., et al. Intranasal dexmedetomidine premedication is comparable with midazolam in burn children undergoing reconstructive surgery. J Burn Care Res. 2009. Jun 5 [Epub ahead of print]
57 Tobias J.D. Subcutaneous dexmedetomidine infusions to treat or prevent drug withdrawal in infants and children. J Opioid Manag. 2008;4(4):187-191.
58 Tobias J.D. Dexmedetomidine to treat opioid withdrawal in infants following prolonged sedation in the pediatric ICU. J Opioid Manag. 2006;2(4):201-205.
59 Tobias J. Sedation and analgesia in the pediatric intensive care unit. Pediatr Ann. 2005;34(8):636-645.
60 Voepel-Lewis T., et al. The prevalence of and risk factors for adverse events in children receiving patient-controlled analgesia by proxy or patient-controlled analgesia after surgery. Anesth Analg. 2008;107(1):70-75.
61 Vondracek P., Bednarik J. Clinical and electrophysiological findings and long-term outcomes in paediatric patients with critical illness polyneuromyopathy. Eur J Paediatr Neurol. 2006;10(4):176-181.
62 White M., et al. Pain management in fulminating ulcerative colitis. Paediatr Anaesth. 2006;16(11):1148-1152.
63 Williams S., et al. Critical illness polyneuropathy and myopathy in pediatric intensive care: a review. Pediatr Crit Care Med. 2007;8(1):18-22.
64 Yuen V.M., et al. A comparison of intranasal dexmedetomidine and oral midazolam for premedication in pediatric anesthesia: a double-blinded randomized controlled trial. Anesth Analg. 2008;106(6):1715-1721.