CHAPTER 65 ALZHEIMER’S DISEASE
DEFINITION
Two excellent sets of clinical criteria with good clinicopathological correlation are given in Tables 65-1 and 65-2.
Memory impairment: impaired ability to learn new information or to recall previously learned information
|
DSM-IV, Diagnostic and Statistical Manual of Mental Disorders, Fourth Edition (American Psychiatric Association, 1994); HIV, human immunodeficiency virus.
TABLE 65-2 NINCDS-ARDA Criteria for Diagnosis of Probable Alzheimer’s Disease
CT, computed tomographic; EEG, electroencephalographic; NINCDS-ADRDA, National Institute of Neurological Disorders and Stroke–Alzheimer’s Disease Related Disorders Association.
Adapted from McKhann, G, Drachman D, Folstein M, et al: Clinical diagnosis of Alzheimer’s disease: report of the NINCDS/ADRDA Work Group under the auspices of Department of Health Services Task Force on Alzheimer’s Disease. Neurology 1984; 34:939-944.
EPIDEMIOLOGY
Alzheimer’s disease causes about 70% of cases of dementia.1 In the Framingham study, Bachman and colleagues reported that the incidence of Alzheimer’s disease increases from 3.5 per 1000 per year between the ages of 65 and 69 to 72.8 per 1000 per year at ages 85 to 89 years.2 Bachman and colleagues found that the incidence of Alzheimer’s disease doubled with every 5 years of age. Alzheimer’s disease prevalence is low among persons younger than 65 years, but it increases to 10% to 30% among persons older than 85.3
In 1907, when Alois Alzheimer described the disease named for him, mean life expectancy was 42 years; since then, in developed countries, life expectancy has greatly increased.4 For example, in the United States, 50% of persons are expected to live past 75 and 25% past 85. With age being such an important risk factor and so many people living into the high-risk ages, the prevalence of Alzheimer’s disease has greatly increased; it is 4.5 million in the United States, according to the 2000 census.5 Because of the staggering cost of caring for patients with Alzheimer’s disease (estimated at $100 billion annually in the United States6) and because “baby boomers” are reaching the high-risk period, it can be argued strongly that the world should invest heavily in finding ways to prevent this disease. In fact, Brookmeyer and associates7 predicted that if the onset of the disease is delayed by an average of 5 years, the present-day U.S. prevalence of Alzheimer’s disease would remain constant (at 4.5 million) instead of increasing to an anticipated 13 million in 2047.
RISK AND PUTATIVE PROTECTIVE FACTORS FOR ALZHEIMER’S DISEASE
Table 65-3 lists risk factors and Table 65-4 the putative protective factors for Alzheimer’s disease.
TABLE 65-4 Putative Protective Factors for Alzheimer’s Disease
Genetics
Researchers have discovered three genes that, if they have undergone mutation, cause early-onset Alzheimer’s disease. Although they account for fewer than 1% of cases of Alzheimer’s disease, the knowledge of how these genes probably cause Alzheimer’s disease has been helpful for understanding the pathogenesis of this disease. Table 65-5 lists the genes from the Alzheimer Disease and Frontotemporal Dementia Mutation Database and tabulates for each gene the number of mutations and families reported. By January 2005, investigators had reported a total 404 families with 189 different mutations in these three genes.
Gene | Number of Mutations | Total Number of Families Published |
---|---|---|
APP | 18 | 48 |
PSEN1 | 141 | 280 |
PSEN2 | 10 | 16 |
Based on Alzheimer Disease and Frontotemporal Dementia Mutation Database, maintained by Marc Cruts and Roos Rademakers (www.molgen.ua.ac.be/ADMutations/, accessed June 8, 2006).
APP, amyloid precursor protein; PSEN, presenilin.
Many studies (summarized in a meta-analysis by Farrer and colleagues8) have established apolipoprotein E4 (ApoE4) as an important risk factor for Alzheimer’s disease. ApoE is a lipoprotein that carries cholesterol and has three forms: ApoE2, ApoE3, and ApoE4. The allelic prevalence varies in different ethnic groups. Table 65-6 reveals that persons with Alzheimer’s disease have a higher prevalence of ApoE4. Although community-based studies do not show ApoE4 as a risk factor for Alzheimer’s disease in African American patients, a large clinically based series reported that it is.9 One of Graff-Radford and colleagues’ findings in that report was that ApoE4 is associated with early-onset risk. In the community-based studies, there were very few early-onset cases.
TABLE 65-6 Apolipoprotein (Apo) Genotypes Related to Alzheimer’s Disease in Different Population Groups, Divided into Cases and Controls
There is strong evidence that there are undiscovered genetic factors related to late-onset Alzheimer’s disease.10 Although many candidate genes have been proposed, associations have often not been reproduced by different investigators. Therefore, researchers are working on refining their techniques and working out the pitfalls in case-control methods. Difficulties in the studies so far include heterogeneity of populations, the possible complexity of the factors causing the disease, the fact that many of the controls are probably susceptible themselves to the disease (as they age), and the fact that the effect size is too small to detect with the size of the samples used.
Amyloid Hypothesis
When Alzheimer described the disease histologically, he noted the deposition of a substance in the form of plaques that was later identified as the β amyloid protein (Aβ) by Glenner and Wong.11 Subsequently, many lines of evidence have led to the hypothesis that brain deposition of this protein causes Alzheimer’s disease.
Aβ is produced by the sequential proteolytic cleavage of the amyloid precursor protein (APP) (coded for on chromosome 21) by enzymes known as secretases (Fig. 65-1). There are two pathways, one of which leads to the production of the Aβ peptides and the other does not. In the amyloidogenic pathway, the first step involves cleavage of APP at the amino terminus by an aspartyl protease (β-secretase), resulting in the formation of soluble APPβ and a membrane-bound APP carboxy terminal fragment (CTF-β). Cleavage of this CTF-β by γ-secretase results in the formation of Aβ peptides of variable length, including a 40–amino acid Aβ peptide (Aβ40) and a 42–amino acid peptide (Aβ42). The γ-secretase cleavage requires the presence of two membrane proteases, presenilin-1 and presenilin-2; it is thought that these presenilins may, in fact, be part of the γ-secretase complex. Other parts of this complex include nicastrin, a type I membrane protein that was originally purified, and two other membrane proteins, APH-1 and PEN-2. Together with presenilin, these three components appear to constitute a minimal functional γ-secretase complex. It is also possible that these “accessory” proteins are involved in substrate presentation. The non-amyloidogenic pathway is carried out by α-secretase, which cleaves the APP protein in the middle into soluble APPα and CTF-β, which, in turn, is cleaved by γ-secretase into P3 and CTF-γ (see Fig. 65-1).
Aβ42 constitutes less than 10% of the total secreted amyloid protein and is more likely to form fibrils than is Aβ40.12 Aβ42 is found in the brains of all patients with Alzheimer’s disease, and Aβ40 is found in two thirds.13 Both accumulate as extracellular plaques consisting of β-pleated sheets, but before plaque formation, a combination of fewer Aβ molecules form oligomers, which may mediate the neurotoxic effects seen in Alzheimer’s disease. Understanding of the generation of Aβ has proceeded at a faster rate than understanding of the pathways that lead to neuronal dysfunction and death. Aβ may be neurotoxic directly, may initiate inflammation, may cause oxidative stress, or may affect calcium homeostasis, or it may lead to a combination of all of these. Table 65-7 lists some of these factors supporting the amyloid hypothesis.
TABLE 65-7 Factors Supporting the Amyloid Hypothesis as a Cause of Alzheimer’s Disease
Aβ, β amyloid protein; CSF, cerebrospinal fluid.
Arguments against the Amyloid Hypothesis
The anatomical distribution of the tangle (tau) pathology is correlated better with the clinical picture than is distribution of the Aβ plaques.14 Because of this, some authors have proposed that tau, rather than Aβ, is the cause of the disease. Furthermore, there are many patients with extensive Aβ plaques who are not demented. Their condition is referred to as pathological aging in the literature.15 Because tau pathology is accelerated in the double-transgenic mouse16 by Aβ deposition, it is possible that Aβ deposition may be partially responsible for the tau pathology. Also, in the cases of early-onset Alzheimer’s disease, such as those caused by the APP mutations, there is typical tau pathology. Thus, attributing the disease exclusively to either the Aβ or tau pathology is incorrect; rather, it is much more likely that both pathological processes are involved. In further studies, investigators should work out how they interact, rather than ruling out one. Other important factors found in brains of patients with Alzheimer’s disease, including oxidative stress, inflammation, cell loss, apoptosis, and synapse loss, are probably crucial in the pathogenesis. Many researchers are studying the relationship of these factors to the amyloid and tau pathology.
PATHOLOGY
A definite diagnosis of Alzheimer’s dementia requires histopathological confirmation.
On gross examination (Fig. 65-2), the brain weight varies between 900 and 1200 g. The loss of brain weight is more marked in the early-onset form of Alzheimer’s disease, whereas in the late-onset form, there is substantial overlap with findings in age-matched controls. The temporal and parietal lobes are more affected than the frontal lobes. The occipital lobe may be involved but is relatively spared in the majority of cases. On examination, the diseased brain may appear normal in the early stages, but closer inspection usually reveals temporal lobe atrophy of its medial aspect with thinning of the cortical mantle. Other features include enlargement of the third and lateral ventricles, normal pigmentation of the substantia nigra, and pallor of the locus ceruleus in advanced cases. Alteration of pigmentation in the brainstem raises the possibility of other neurodegenerative disease (for example, Lewy body disease). In Alzheimer’s disease, the olfactory bulb is consistently smaller than expected. On occasion, atrophy may be asymmetrical or focal, and cases of Alzheimer’s disease with focal atrophy may be associated with focal cognitive deficits such as progressive aphasia, Balint’s syndrome, or frontal dementia.
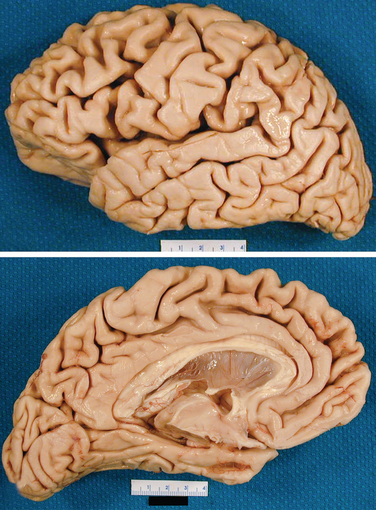
Figure 65-2 Gross appearance of the brain of a patient with Alzheimer’s disease.
(Courtesy of Dennis Dickson, MD.)
The cardinal histopathological lesions of Alzheimer’s disease are senile plaques composed of extracellular amyloid deposits (Fig. 65-3) and neurofibrillary tangles composed of intraneuronal tau protein aggregates (Fig. 65-4). The plaques are detected by silver staining (Bielschowsky stain) or by specialized amyloid stains (thioflavin S, Congo red). The β-pleated sheet structure gives rise to the staining characteristics: that is, a birefringent apple-green appearance under polarized light. Some plaques are “diffuse,” and these may be present in nondemented individuals. These plaques do not have dystrophic neurites (nerve endings containing tau protein), unlike the “cored” or neuritic plaques, which have deposition of extracellular amyloid surrounded by tau-containing neurites. Neurofibrillary tangles are filamentous inclusions that are intraneuronal and are composed of hyperphosphorylated aggregates of the microtubule-associated protein tau. Electron microscopy reveals that tau consists of a paired helical filament structure. Initially, tau collects in the nucleus and becomes aggregated into paired helical filaments, after which it may be attached to the protein ubiquitin. These tau tangles affect the microtubules, which, in turn, interfere with neuronal and dendrite/axonal transport. The neurons die and are surrounded by phagocytes removing the debris. Eventually, the tangles become extracellular structures.