Ageing
INTRODUCTION AND OVERVIEW
The global population trends of ageing reported by the US government show that the world population nearly quadrupled during the twentieth century, and is projected to grow by roughly 50% before stabilising during the late twenty-first century.1 This transition is expected to leave the population much larger and, on average, older than it was previously, with significant health and socioeconomic implications.
Before the demographic transition of populations began,2,3 fertility and mortality were both high throughout the world. Children often died in infancy, and people who reached adulthood tended to have many children and die relatively young. Famines and epidemics could rapidly and suddenly kill many people, causing large fluctuations in rates of mortality. As a consequence, most people were young, and very few lived to old age.2
The pattern of population mortality change has a number of recognised stages (listed below) and links with the epidemiological transition that began in Europe in the 1700s (Fig 58.1).3
At present, people born in one of the developed countries can expect to live well into their mid-seventies, or longer if current mortality rates prevail, and even longer if those rates continue to fall. Life expectancy at birth in these countries is therefore projected to rise continuously well into the future. This trend is exemplified in the growth of the world’s population (Fig 58.2).
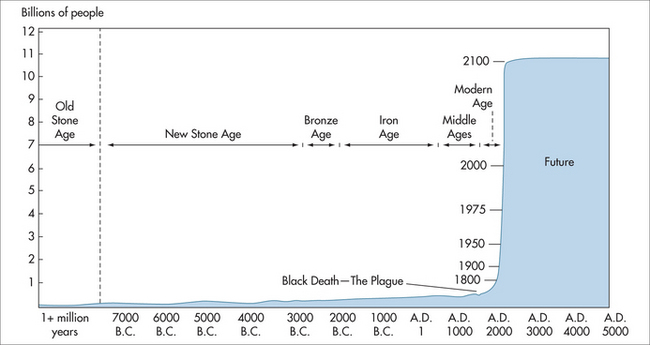
FIGURE 58.2 World population growth throughout history
(adapted from Population Reference Bureau5 and United Nations 19986)
Significant gains have occurred through reductions in death rates among the middle-aged and elderly, especially from artery disease (heart disease and stroke), over the past few decades. In Australia, males aged 30 in 2001 could expect to live to 78 years and females to 82.8 years.7 This is about 12 years longer than the respective life expectancies during 1901–1910. Males aged 65 years in 2001 could expect to live to 81.6 years and females to 85.2 years, about 6 years longer than for those in 1901–1910.1–3,7 Similar trends can be observed with the populations from other developed countries, such as the United States.1
Ninety per cent of all healthcare dollars are spent on extraordinary care in the last 2–3 years of life.2,3 The leading causes of death have undergone a profound shift, primarily due to improvements in sanitation and infection control since the turn of the twentieth century. The most common causes of death are now cardiovascular disease (heart disease and stroke) and cancer, and these diseases consume approximately 50% of the healthcare budget.7 In the United States, the reported leading causes of death include heart disease, malignant neoplasms, cerebrovascular diseases (stroke), chronic lower respiratory diseases, accidents (unintentional injuries), diabetes mellitus and Alzheimer’s disease.8 Nearly 80% of people aged 65 years or older in the United States have at least one chronic condition, such as heart disease, diabetes, arthritis or depression, and half have at least two chronic conditions.2,8 These trends are also applicable to other developed countries.1,9 In the United States, chronic diseases account for 16.2% of the nation’s gross domestic product, and this is among the highest of all industrialised countries.9
In order to make an impact on healthcare, there must be a focus on preventing degenerative diseases of ageing that are lifestyle-related.9 There needs to be an emphasis on preventing, delaying or reversing the diseases associated with ageing. In the past 10 years, fewer Australians have died from heart attacks, strokes and cancer. The life expectancy of Australians continues to increase; however, there are a number of areas where we can do better. For example, Australians are getting fatter and exercising less, which has serious implications for the health of our population and potentially could reduce life expectancy in the future.7 Recently, an international Chronic Disease Action Group was established that will encourage, support and monitor accomplishments on the implementation of evidence-based efforts to promote global, regional and national actions to prevent and control the development of chronic diseases.10
LIFE EXPECTANCY
Japan has the highest life expectancy of any nation. Approximately one-third of those aged over 110 years worldwide are living in the Okinawa region of Japan.11–13 It is clear that the Japanese rural lifestyle and diet are important in determining their longevity.
When the Japanese move to the United States, their life expectancy is reduced to that of the local population by the second generation. Japanese who migrate to the United States develop breast cancer at the same rate as locals after one generation, but with bowel cancer a similar rate occurs by the second generation.14
Considerable attention has also been focused on populations from three other geographical areas in the world: Abkhazia, in Georgia; Hunza, in Northern Pakistan; and Vilcabamba, in Ecuador. Life expectancy in these regions is considered to be much higher than average.13–19 In these areas, several observations have been made indicating that these people live in an area that is reasonably isolated and has little pollution. Some of the features of long-lived populations such as those documented from Okinawa are that families are closely knit, couples report having happier relationships, the elderly are respected within their communities, in general their diets would be considered healthy, and their level of physical activity is high. It is of great significance that not only do these groups live to an above-average life expectancy, but they are also healthy most of the time when they are elderly.15–17
PHYSIOLOGICAL CHANGES WITH AGEING
The ageing process is characterised by a number of factors that can reduce human mean life expectancy, and these changes are summarised in Table 58.1.
System | Major changes |
---|---|
Musculosketal/Body composition |
Level of evidence: I = strong, II = moderate.
MOLECULAR BIOLOGY OF LONGEVITY
CELL DIVISION, CHROMOSOMES AND TELOMERES
The ends of chromosomes are called telomeres (Fig 58.3). In most animal cells the enzyme responsible for replication of the ends of the chromosomes is telomeradse.20–22
If the telomeres of the chromosomal DNA are lost, cell cycle or cellular functions are lost, resulting in reduction of cell proliferation and re-differentiation. DNA nucleotides are added to the tip of the strand by the reverse transcriptase enzyme called telomerase. Telomerase hence adds DNA sequence repeats (i.e. TTAGGG in all vertebrates) to the 3′ end of DNA strands in the telomere regions. These regions are found at the ends of eukaryotic chromosomes, stabilising the structure and function of the entire chromosome. Normal human somatic cells undergo a finite number of cell divisions before they reach a non-dividing or senescence state. Each time a cell divides, the telomeres shorten due to the end of replication. As a consequence, this is reflected by the ever-shortening telomeres during organismal ageing, which is documented to occur in most animals.20–22 The absence of telomere shortening has led to the hypothesis of telomere length and cell longevity.24,25
It is possible to influence the length of telomeres of human cells in vitro by adding telomerase. This has subsequently been reported to decrease the rate of cellular ageing.25 Cancer cells do not seem to lose their telomerase activity and hence do not undergo cell death, indicating that cancer and longevity may have common properties.
A gene called longevity assurance or LAG-1 gene has been discovered in yeast cells.24 The LAG-1 gene can influence the number of yeast cell divisions and the cell’s longevity. The function of this gene is unknown, but it is believed to result in the synthesis of a protein found in the cell membrane. Attempts to clone a similar human gene are under way to ascertain whether it may influence longevity of the human cell.
In 1987, Denham Harman proposed that oxygen free radicals cause much of the damage associated with ageing.26 Oxygen free-radicals are molecules with an unpaired highly reactive electron produced normally as the body converts food and oxygen into energy. In an attempt to stabilise the free-radical, oxygen molecules take up an electron from another molecule, which then becomes unstable and starts a chain reaction. Some of these free-radicals are harmful to proteins, DNA, cell membranes and other cell structures such as mitochondria. In the normal cell most oxidative damage can be prevented by enzymes such as superoxide dismutase (SOD), catalase, glutathione, peroxidase and antioxidants such as vitamins C, E and beta-carotene, but the damage to the cells is felt to be cumulative. SOD levels and other antioxidants have been correlated with lifespan in at least 20 species.
MITOCHONDRIA AND FREE-RADICALS
That mitochondria have a pivotal role in the effective provision of energy to eukaryotic cells is an undisputed scientific fact. Cellular mechanisms regulating energy utilisation must function properly to sustain life. With increased ageing, there is a decrease in mitochondrial energy output.27 Hence, in aerobic animals, mitochondrial health for effective energy provision is central to life.
The free-radical theory of ageing, as formulated by Denham Harman,26 is supported by observations that the lifespan of most organisms is roughly proportional to their metabolic rate and thus due to the rate at which the organism generates mitochondria-derived reactive oxygen species (ROS). This view, however, may require modification within the confines of ageing. Cellular-generated ROS contributing to the overall production of ROS are apparently traced back to the mitochondria.27 ROS have been viewed as mostly deleterious to health and hence ageing. Reports that show that in a wide spectrum of animal species, dietary antioxidants or caloric restriction as well as chemical antioxidants or increased expression of antioxidant proteins can lower mitochondrial ROS production, which translates into an extension of the lifespan of these species, serve to support the free-radical theory of ageing.28–31 However, it is known that ROS are generated in multiple cellular compartments and by multiple enzyme systems within the cell and have cellular signalling functions that are critical for the normal physiological function of the cell.32–34
ROS produced by mitochondria have been demonstrated to have important and specific roles in cellular signalling.34 The notion that the mitochondria are the sole most abundant site of ROS formation is currently subject to much discussion and debate.33–38
The disruption of mitochondrial functions has been implicated in more than 40 known diseases, including atherosclerosis, ischaemic heart disease, cancer, diabetes and neurodegenerative diseases such as Alzheimer’s disease, Parkinson’s disease, Huntington’s disease and amyotrophic lateral sclerosis.39–41 Together these data indicate that mitochondrial health is an important factor for health and ageing. Current and future research would aim to further improve and preserve mitochondrial function. Although further research is warranted, recent reports show that supplementation with coenzyme Q10 shows promise in maintaining the health of mitochondria.42,43
ENDOCRINOLOGY OF AGEING
It is possible that the physical changes associated with ageing are physiological, but there is some evidence to suggest that a decline in hormonal activity plays an important role. As a result, studies have looked at the role of hormonal replacement strategies, so as to increase blood hormone levels. Unfortunately, the use of hormone replacement therapy (HRT) was not an overall success. There may be specific reasons for this failure, and hence more studies are necessary in order to establish whether hormone replacement can be both of benefit and safe.
PHYSICAL FRAILTY WITH AGEING
Physiological functions gradually decline with ageing. Such decline in functions includes diminished capacity for cellular protein synthesis, a decline in immune function, an increase in fat mass, a loss of muscle mass and strength and a decrease in bone density. People die from old age and it is mostly from cardiovascular disease, cancer or dementia. The characteristics of ageing include generalised weakness, impaired mobility and balance and poor endurance.44,45
Associated with physical frailty are falls (contributing to 40% of admissions to nursing homes), fractures that impair daily activities of everyday living and loss of independence.46
Experts on ageing define the characteristic aspect of ageing as a loss of muscle strength.33,37 A decline in muscle strength results from a sedentary lifestyle and decreased physical activity, as well as ageing of muscle fibres and their innervation, osteoarthritis and chronic debilitating diseases.47
A study utilising 100 frail nursing home residents with an average age of 87 years investigated supervised resistance exercise training, and found a doubling of muscle strength and a significant increase in their walking and climbing power. The investigation demonstrated that these muscle changes of ageing are not irreversible and that they can be reduced and possibly prevented. Prevention of frailty can be achieved by exercise, which is difficult to institute in an ageing population and, hence, there are very high numbers of dropouts from these programs.48 Muscle resistance exercises can also improve insulin sensitivity.49 A cultural change where exercise becomes a routine part of life may make a difference.
Changes in the endocrine system may be responsible for part of the ageing process that affects the body composition, including loss of muscle size and strength, loss of bone and increase in fat mass.50
PHYSICAL ACTIVITY AND AGEING
One of the common traits of the elderly is sarcopenia, which gives them the appearance of frailty due to loss of skeletal muscle mass. In a study with a representative North American population sample, it was reported that sarcopenia increased from 24% in those aged less than 70 years of age to over 50% in those aged over 80 years of age.51 The age-related sarcopenia that results in loss of skeletal muscle mass has been associated with a decrease in muscle fibre area, especially type II fibre.52 There are numerous consequences related to this reduction in muscle mass, including a decline in muscle strength and function, and impaired functional capacity. Sarcopenia also results in a reduction in the body’s major protein pool. Adequate dietary protein to replace obligatory nitrogen loss and to support protein turnover is essential for maintaining muscle mass, and therefore nutritional options are equally important in order to reduce and significantly slow the progression of loss of skeletal muscle mass. It is usually recommended that protein requirements in older people should be maintained above 1 g/kg/day. An inactive lifestyle may contribute to the loss of skeletal mass in elderly people. Physical activity can significantly assist in reversing this deficit and may improve the regeneration potential of muscle fibres.52
HORMONES AND AGEING
PANCREAS AND THYROID FUNCTION
The reduction of pancreas and thyroid function is clinically the most important change in endocrine activity with ageing. Approximately 40% of those aged 65–74 years and 50% of those aged over 80 years old have diabetes mellitus and nearly half are undiagnosed.53,54 Apart from insulin secretion by the beta cells, there is also insulin resistance related to stress, poor diet, lack of exercise, increased abdominal fat mass and decreased lean body mass.55–57
Age-related thyroid dysfunction is also common in the elderly.58,59 Lowered thyroxine (T4) and increased thyroid thyrotropin-stimulating hormone (TSH) occur in approximately 5–11% of elderly hospitalised men and women.60–62 Dysfunction is mainly the result of autoimmunity and is not a consequence of ageing.63–65 Ageing is accompanied by a decrease in TSH release and a decline in conversion of T4 to the more active triiodothyronine (T3).65 A study has found that T3 and T4 lead to improvements in cognition, depression, fatigue and general wellbeing.66
MENOPAUSE
The evidence that both the brain and the ovary are key pacemakers in menopause is compelling.67,68 Menopause does not result only from exhaustion of ovarian follicles.
Whereas menopause occurs quite abruptly, the changes in the hypothalamic–pituitary–gonadal axis in males are slower and more subtle (andropause, see below). There is a gradual decline of testosterone with ageing.69,70 This andropause is characterised by a decrease in testicular Leydig cell numbers and a decrease in gonadotropin secretion.69,70
In most women, the period of decline in oestrogens is accompanied by vasomotor reactions, depressed mood and changes in skin and body composition (increase in body fat and decrease in muscle mass). Menopause is associated with the increased incidence of cardiovascular disease, loss of bone mass and cognitive impairment.71,72 With increasing life expectancy, the time a woman spends after menopause is more than a third of her life.
A large prospective study on HRT, the Women’s Health Initiative, found that HRT helped in reducing hip fracture and bowel cancer but had negative effects in increasing heart deaths, thrombosis (embolic disease) and breast cancer. This study proved that the form of HRT being used was an overall failure.73 The results of this study were confirmed by the Million Women Study.74
The significant adverse effects that have been documented with the long-term use of HRT have elicited caution in relation to the long-term use of other forms of HRTs, such as natural oestrogens (e.g. oestradiol valerate extracted from soya beans), synthetic oestrogens (e.g. ethinyloestradiol), tibolone (a synthetic hormone that has some oestrogen-like and some progesterone-like activity), skin patches and oestrogen gels, oestrogen implants, vaginal oestrogen, phyto-oestrogens, progesterone treatments (e.g. tablets: dydrogesterone, levonorgestrel/norgestrel, medroxyprogesterone; patches) and testosterone replacement.72–74
ANDROPAUSE
There is a gradual decline in testosterone levels with ageing. Variability exists among the aged. Of those men aged over 65 years, two-thirds have testosterone levels below the normal values of men aged between 30 and 35 years.75 Impotence increases in men aged 60–70 years, to over 50%.76–78 There is an association between decline in free testosterone levels and increase in impotence.79 Overall, testosterone replacement therapy is not effective for the treatment of impotence in elderly males. Other factors such as artery disease, diabetes, excessive alcohol consumption, smoking and quality of the relationship seem to be more important.80,81
Although it has been shown that testosterone has anabolic effects,82–85 it is not clear whether the decline in muscle mass and muscle strength with ageing is related to the decrease in free testosterone levels. In mid-adult hypogonodal men who have muscle weakness, anaemia, decreased bone mass and mood disturbances, there is a rapid normalisation with testosterone replacement therapy.83,84 Pharmacological doses of testosterone therapy combined with exercise or weight training have been shown to increase muscle mass and strength in eugonadal adult men.84 An early study demonstrated that testosterone supplementation for 6 months in older men with a low normal testosterone concentration did not affect functional status or cognition but increased lean body mass and had mixed metabolic effects.85
There are limited long-term data on testosterone replacement therapy but in general there is a positive effect on muscle mass and strength as well as on cognition and sense of wellbeing.86,87 An adverse effect on lipid profiles has been reported with testosterone treatment.87 There are inadequate studies on testosterone replacement therapy in the elderly to ascertain who benefits and whether prostate disease and artery disease negate these benefits. Androgenic compounds with variable biological actions in different organs (antiandrogenic in the prostate) are currently being investigated.88 Moreover, a recent review concluded that on current evidence the studies in elderly men with lower than normal testosterone that have reported improvement in features of the metabolic syndrome, bone mineral density, mood and sexual functioning have provided no definitive proof. The beneficial effects of restoring testosterone levels to normal in elderly men, with regard to clinical parameters, requires further rigorous research.88
ADRENOPAUSE
With age there is a gradual decline in dehydroepiandrosterone (DHEA), resulting in adrenopause.89,90 Adrenal secretion of DHEA gradually decreases over time, whereas adrenocorticotropin (ACTH) secretion, which is physiologically linked to plasma cortisol levels, remains largely unchanged. The decrease in DHEA is due to a decrease in production from the adrenal cortex and is not due to a hypothalamic pacemaker.
Males over 90 years of age with the lowest DHEA levels have the lowest levels of activities of daily living.90 DHEA levels at age 30 are approximately five times higher than at age 85.90
In animal studies with very low levels of DHEA, its administration prevents obesity, diabetes mellitus, cancer and heart disease, while enhancing immune function.91–94 Supportive data in humans are few and highly controversial. Higher DHEA levels are associated with some reduced risk of cardiovascular deaths in males.91
DHEA has been shown to be beneficial in two randomised placebo-controlled studies.95,96 Twenty adults aged between 40 and 70 years were given 50 mg of DHEA daily over a 3-month period, with an increase in DHEA levels similar to those in young adults, resulting in increased androgen and insulin-like growth factor (IGF-1) concentrations. There was also a significant increase in physical and psychological wellbeing without an effect on libido in both sexes.96
Another study using 100 mg of DHEA for 6 months showed an increase in lean body mass in both sexes, but muscle strength was increased in men only.97
Recent short-term and long-term studies investigating the administration of an oral low dose (25 mg/day) of DHEA have demonstrated that DHEA increased serum DHEA, DHEAS, androstenedione and oestradiol levels of participants in the older group to the same level as that of younger participants.98 Further, it has been shown that DHEA was capable of modifying circulating levels of androgens and progestins in both early- and late-postmenopausal women by modulating the age-related changes in adrenal function.99
SOMATOPAUSE
The growth hormone (GH) / insulin-like growth factor I (IGF-1) is the third endocrine system that gradually declines with ageing.100,101 There is a progressive fall in circulating IGF-1 levels in both sexes with ageing.102 The triggering pacemaker appears to be localised in the hypothalamus and is linked with a decrease in GH.103 It is not known whether changes in gonadal function (menopause and andropause) are interrelated with adrenopause and somatopause, which occur in both sexes.
Changes such as muscle size and function, fat and bone mass, progression of atherosclerosis and changes in cognitive function have not been related to the changes in GH/IGF-1 endocrine activity. Some effects of normal ageing resemble features of isolated hormonal deficiency (i.e. hypogonadism and GH deficiency), which in mid-adult patients are reversed by replacement therapy with the appropriate hormone.104 Ageing is not simply the result of various hormone deficiencies.
The biological consequences of somatopause are poorly understood.105 A number of catabolic processes that are central in the biology of ageing can be reversed with GH administration. The IGF-1 levels in elderly individuals can be increased to those seen in the young after 3–6 months of GH administration.106 GH also significantly increased muscle mass, skin thickness and bone mineral, and reduced fat mass.107 Muscle strength and maximal oxygen consumption were not improved with GH therapy.108 GH therapy did not give any additional benefit to that of resistance exercise training in relation to muscle mass and muscle strength.109 An association between maximum aerobic capacity and circulating IGF-1 levels has been demonstrated.108,109
In summary, GH replacement therapy in GH-deficient adults has been shown to increase:
In addition, lipid profile was improved and fat mass decreased.106–109
If GH is given to those over 65 years old with hip fracture, there is an earlier return to independent living.110
Several other similar studies have investigated the role of GH in the treatment of acute catabolic states in the frail elderly. Hypothalamic peptide growth hormone releasing hormone (GHRH), when given subcutaneously over 2 weeks to healthy 70-year-old men, increased GH and IGF-1 levels to those of 35-year-olds.111 Long-term oral non-peptide analogues of GH-releasing peptides have even more powerful GH-releasing effects, restoring IGF-1 secretion in the elderly to those of young adults.112 Long-term administration in the elderly increased lean body mass but not muscle strength.113 These non-peptide analogues may be important in the prevention of frailty and reversing acute catabolism. Studies of the long-term use of these analogues are not available. There is concern about tumour growth, as IGF-1 receptors are present in most human solid cancers. The concerns related to GH have recently been reviewed.114
LIFESTYLE
Willet emphasised that genetic and environmental factors, including diet and lifestyle, both contribute to cardiovascular diseases, cancers and other major causes of mortality and that numerous lines of evidence indicate that environmental factors are the most important in disease prevention (Fig 58.4).115 Hence, environmental factors may certainly have the strongest influence on life expectancy and hence lifespan (Fig 58.2).115,116 It should be noted that life expectancy is a statistical projection of the length of time a human being is expected to live, based upon probabilities and assumptions of genetic predispositions, living conditions, medical discoveries and advances, natural disasters and other environmental factors, compared with lifespan defined as the characteristic observed age of death for its very oldest individuals.117 Intertwined with nutritional practices and lifestyle is life stressor modification.116
Stressors and negative stress-related reactions have been documented and recognised as having multiple ill-health-related sequelae, and exposure to chronic social stress has been associated with many systemic and mental disorders.118 These have significant deleterious outcomes that significantly reduce life expectancy.
Different research groups support the notion that health consequences are more likely to occur when unpredictable stressors of a social nature chronically induce physiological and behavioural adjustments that may create wear and tear on underlying physiological functions. When stressors challenge an organism’s integrity, a set of physiological reactions is elicited to counteract the possible threat and adjust the physiological setting of the organism to the new situation. This has become known as the stress response.119
Stressors and lifestyle choices can be a significant trigger for disease through immunomodulation of the immune system enhancing disease susceptibility, which can then affect life expectancy.119 Immune function modulation influenced and expressed by stressful life experiences has a significant correlate with Willett’s115 environmental trigger view of disease initiation.
Some recent research is now suggesting that psychological factors such as chronic stress and pessimism120 may have a direct effect on telomere length and telomerase activity, thus giving plausible mechanisms to explain the relationship between mental health and ageing. There is now also research to suggest that psychological strategies such as mindfulness techniques may help to slow the ageing process through their effects on stress, cognition and, consequently, telomerase activity.121
NUTRITION
Triggers for adverse and inappropriate nutritional practices in childhood have been shown to have psychosocial correlates in adult life, with an increase in risk for disease.122
Recently it was reported, and further confirmed, that a greater adherence to a traditional Mediterranean diet was associated with a significant reduction in total all-cause mortality.123 Meyer and colleagues have demonstrated that associating a CR diet with adherence to a Mediterranean-type diet that consisted of whole grains, beans, fish, fruit, olive oil and many different kinds of vegetables was beneficial for heart health.124 Hence, when optimal nutritional choices are coupled with CR, an increase in life expectancy is made possible. Such nutritional practices can serve to further extend the human lifespan.
CALORIC RESTRICTION
Optimising nutrition relates food intake to the benefits of prudent CR. That is, CR refers to a dietary regimen low in calories without under-nutrition that would lead to increased disease risk due to nutritional deficits. Following the pioneering work of McCay and colleagues over 70 years ago, CR was then first noted to significantly extend the lifespan of rodents.125 Since that time, the increase in longevity has been demonstrated to result from the limitation of total calories derived from carbohydrates, fats or proteins to 25–60% below that of control animals fed ad libitum.29,126,127 The extension in lifespan can approach 50% in rodents.125,128 Moreover, CR has been shown to extend the lifespan in a broad range of organisms including yeast, rotifers, spiders, worms, fish, mice and rats.126 Emerging data show that its effect may also apply to non-human primates.129 CR has also been reported to delay a wide spectrum of diseases in different experimental animals such as kidney disease,129 a variety of neoplasias,130–132 autoimmune disease133,134 and diabetes,135 and it reduces age-associated neuronal loss in most mouse models of neurodegenerative disorders such as Parkinson’s disease136 and Alzheimer’s disease.137,138 The CR regimen also prevents age-associated decline in psychomotor and spatial memory tasks139 and loss of dendritic spines necessary for learning,140 and improves the brain’s plasticity and ability to self-repair.137
Numerous biomarkers of CR have been identified in rodents, such as temperature, and DHEAS, insulin and glucose levels.141 Roth and colleagues have recently observed that body temperature and insulin and DHEAS levels were also altered in primates that had been subjected to CR, hence validating the usefulness of these biomarkers in longer-lived species.141 More importantly, they have also shown that these parameters were altered in longer-lived men. Together these findings support the role of these factors as biomarkers of longevity in humans.
Recently, a trial on the effect of a 6-month CR diet on metabolic biomarkers such as energy expenditure, and oxidative stress in humans was completed.142 The report showed that prolonged CR significantly reduced two biomarkers of longevity, namely, fasting insulin level and body temperature. This is the first human study to show that CR, in addition to significantly reducing well known biomarkers of ageing, also caused a metabolic adaptation in CR individuals and a reduction in DNA fragmentation that reflected less DNA damage.142
Plant factors such as resveratrol have been shown to increase longevity in several organisms by regulating genes.143 In mammals, there is growing evidence that resveratrol can prevent or delay the onset of cancer, heart disease, ischaemic and chemically induced injuries, diabetes, pathological inflammation and viral infection. These effects are observed despite extremely low bioavailability and rapid clearance of resveratrol from the circulation.143 Resveratrol has been suggested to be associated with the prevention of age-related diseases such as cancer because of its regulation of transcription factors that control tumour cell survival.144,145
NON-DRUG INTERVENTIONS IN CHRONIC DISEASES
The role of non-drug interventions in chronic diseases is summarised in Tables 58.2 and 58.3.
TABLE 58.2 Chronic diseases (by system) and non-drug interventions and supplements
System/disorder | Non-drug interventions and supplements* and strength of evidence |
---|---|
Musculoskeletal/Body composition | |
Level of evidence: I = strong, II = moderate, III = weak.
* Progression halted by lifestyle changes including diet, stress reduction, exercise, relaxation techniques and adequate sunlight exposure.
TABLE 58.3 Chronic diseases and non-drug interventions and supplements
Disorder | Non-drug interventions and supplements* and strength of evidence |
---|---|
Metabolic syndrome | |
Chronic inflammation: can involve most body systems | |
Cancer | |
Behavioural | |
Pain | Pain relief with acupunctureI, antiinflammatory herbs (see above) |
Urinary problems | Saw palmettoII, other herbs: stinging nettle (Urtica dioica)II, pygiumII |
Sleep apnoea |
Level of evidence: I = strong, II = moderate, III = weak.
* Progression halted by lifestyle changes including diet, stress reduction, exercise, relaxation techniques and adequate sunlight exposure.
HEALTHY AGEING
Throughout most of recorded human history, it has been recognised that poor socioeconomic and nutritional status are strongly associated with decreased life expectancy, a trend that is also very much evident today.146
The Alameda County Study highlights the importance of an overall plan for health maintenance and disease prevention that can lead to enhanced survival. The Human Population Laboratory, known as the Alameda County Study, quantified health risk practices and lifestyle issues such as exercise, diet, sleep, smoking and alcohol consumption, and defined their relationship to mortality.147 The first cohort participated in a health survey in 1965 and this has been followed with subsequent surveys to the present day. Health behaviours including seven health habits were considered and were reported to be strongly associated with most (current) or subsequent morbidity and mortality. This study emerged from efforts in the 1950s to measure health—physical, mental and social wellbeing—in accordance with the WHO definition of health, and asked questions about common health-related habits.
Lifestyle and future health issues were investigated and it was reported that there was a disparity of good health practices in 1965 between those with good health and those with poor health practices. Those with an intermediate level of health practices experienced about two-thirds the relative disability risk of those with poor health practices.148
Subsequent studies from the Alameda County Study showed that greater personal control over one’s biopsychosocial health after retirement was beneficial to health, providing a greater survival advantage.149,150
Restoration of telomerase activity, isolation and deciphering the activity of longevity genes, protection against environmentally induced free-radicals (e.g. excessive UV radiation) and supplementation with beneficial hormones, nutrients and herbs may increase longevity. We need to learn more about why people from certain countries have a greater life expectancy. Individuals who live to be 100 years of age or more also require further study to show how they may be different from others with an average life expectancy. Increasing knowledge should help us to postpone or prevent the advent of chronic and disabling diseases, as well as resetting the internal biological clocks that control longevity.
Duke University Center for the Study of Aging and Human Development. http://www.geri.duke.edu/resource/resource.html.
National Academy of an Aging Society. http://www.agingsociety.org/agingsociety/index.html.
National Institute on Aging (NIH). http://www.grc.nia.nih.gov/branches/blsa/blsanew.htm.
Resource Centre on Aging, University of California, Berkeley. http://socrates.berkeley.edu/~aging/Web.html.
Rosenthal Centre, Information Resources for Research on Aging. http://www.rosenthal.hs.columbia.edu/Aging_resources.html.
Sheffield Institute for Studies on Ageing. http://www.sheffield.ac.uk/sisa/.
1 Congress of the United States, Congressional Budget Office. Global population ageing in the 21st century and its economic implications. Online. Available: http://www.cbo.gov/ftpdocs/69xx/doc6952/12-12-Global.pdf, 2005.
2 United Nations. World population prospects; 2004 revision. Online. Available: http://www.un.org/esa/population/publications/WPP2004/2004Highlights_finalrevised.pdf.
3 Omran A. The epidemiologic transition: a theory of the epidemiology of population change. Milbank Quarterly. 1971;49:509-538.
4 Vielrose E. Elements of the natural movement of populations. Oxford: Pergamon Press, 1965.
5 Population Reference Bureau. 2009 World population data sheet. Online. Available: http://www.prb.org/pdf09/09wpds_eng.pdf.
6 United Nations, Population Division of the UN Secretariat. World population projections to 2100; 1998.
7 World Health Organization. Data on longevity in Australia. Online. Available: http://www.who.int/countries/aus/en/.
8 Kung HC, Hoyert DL, Xu JQ, et al. Deaths: final data for 2005. National Vital Statistics Reports 2008;56(10). Online. Available: http://www.cdc.gov/nchs/data/nvsr/nvsr56/nvsr56_10.pdf.
9 Centers for Medicare and Medicaid Services, Office of the Actuary, National Health Statistics Group, National Health Care Expenditures Data, January 2010. Online. Available: http://www.cms.hhs.gov/nationalhealthexpenddata/01_overview.asp?.
10 Beaglehole R, Ebrahim S, Reddy S, et al. Chronic Disease Action Group. Prevention of chronic diseases: a call to action. Lancet. 2008;370(9605):2152-2157.
11 Willcox DC, Willcox BJ, Sokolovsky J, et al. The cultural context of ‘successful ageing’ among older women weavers in a northern Okinawan village: the role of productive activity. J Cross Cult Gerontol. 2007;22(2):137-165.
12 Willcox DC, Willcox BJ, Todoriki H, et al. Caloric restriction and human longevity: what can we learn from the Okinawans? Biogerontology. 2006;7(3):173-177.
13 Willcox BJ, He Q, Chen R, et al. Midlife risk factors and healthy survival in men. JAMA. 2006;296(19):2343-2350.
14 Maskarinec G, Noh JJ. The effect of migration on cancer incidence among Japanese in Hawaii. Ethn Dis. 2004;14(3):431-439.
15 Willcox BJ, Willcox DC, Ferrucci L. Secrets of healthy aging and longevity from exceptional survivors around the globe: lessons from octogenarians to supercentenarians. J Gerontol A Biol Sci Med Sci. 2008;63(11):1181-1185.
16 Dodge HH, Kita Y, Takechi H, et al. Healthy cognitive aging and leisure activities among the oldest old in Japan: Takashima study. J Gerontol A Biol Sci Med Sci. 2008;63(11):1193-1200.
17 Mazess RB, Forman SH. Longevity and age exaggeration in Vilcabamba, Ecuador. J Gerontol. 1979;34(1):94-98.
18 Grigorov IG, Kozlovskaia SG, Semesko TM. Characteristics of nutrition in persons of older age groups in areas with different patterns of longevity. Vopr Pitan. 1991;5:24-32.
19 Vlahchev T, Zhivkov Z. Hunza—a healthy and a long living people. Asklepii. 2002;15:96-97.
20 Gilley D, Herbert BS, Huda N, et al. Factors impacting human telomere homeostasis and age-related disease. Mech Ageing Dev. 2008;129:27-34.
21 Hayflick L, Moorhead PS. The serial cultivation of human diploid cell strains. Exp Cell Res. 1961;25:585-621.
22 Nakamura TM, Cech TR. Reversing time: origin of telomeres. Cell. 1998;92:587-590.
23 Weinrich SL, Pruzan R, Ma L, et al. Reconstitution of human telomerase with the template RNA component hTR and the catalytic protein subunit hTR. Nat Genet. 1997;17(4):498-502.
24 Bodnar A, Ouellette M, Frolkis M, et al. Extension of life-span by introduction of telomerase into normal human cells. Science. 1998;279:349-352.
25 Wright WE, Shay JW. Telomere biology in aging and cancer. J Am Geriatr Soc. 2005;53:S292-S294.
26 Harman D. Ageing: a theory based on free radical and radiation chemistry. J Gerontol. 1956;11(3):298-300.
27 Lenaz G, Bovina C, D’Aurelio M, et al. Role of mitochondria in oxidative stress and ageing. Ann NY Acad Sci. 2002;959:199-213.
28 Orr WC, Sohal RS. Extension of life-span by overexpression of superoxide dismutase and catalase in Drosophila melanogaster. Science. 1994;263:1128-1130.
29 Sohal RS, Weindruch R. Oxidative stress, caloric restriction, and ageing. Science. 1996;273:59-63.
30 Parkes TL, Elia AJ, Dickinson D. Extension of Drosophila lifespan by overexpression of human SOD1 in motorneurons. Nat Genet. 1998;19:171-174.
31 Sun J, Tower J. FLP recombinase–mediated induction of Cu/Zn–superoxide dismutase transgene expression can extend the life span of adult Drosophila melanogaster flies. Mol Cell Biol. 1999;19:216-228.
32 Linnane AW, Kios M, Vitetta L. The essential roles of superoxide radical and nitric oxide formation for healthy ageing. Mitochondrion. 2007;7(1/2):1-5.
33 Linnane AW, Kios M, Vitetta L. Healthy ageing: regulation of the metabolome by cellular redox modulation and prooxidant signaling systems. The essential roles of superoxide anion and nitric oxide. Exp Gerontol. 2007;8(5):445-467.
34 Linnane AW, Kios M, Vitetta L. Coenzyme Q10—its role as a prooxidant in the formation of superoxide anion/hydrogen peroxide and the regulation of the metabolome. Mitochondrion. 2007;Suppl 1:S51-S61.
35 Rhee CG, Chang TS, Bae YS, et al. Cellular regulation by hydrogen peroxide. J Am Soc Nephrol. 2003;14(8 Suppl 3):S211-S215.
36 Balaban RS, Nemoto S, Finkel T. Mitochondria, oxidants, and ageing. Cell. 2005;120:483-495.
37 Linnane AW, Eastwood H. Cellular redox poise modulation: the role of coenzyme Q10, gene and metabolic regulation. Mitochondrion. 2004;4(5/6):779-789.
38 Moldovan L, Moldovan NI. Oxygen free radicals and redox biology of organelles. Histochem Cell Biol. 2004;122(4):395-412.
39 Schon EA. Mitochondrial genetics and disease. Trends Biochem Sci. 2000;25:555-560.
40 McKenzie M, Liolitsa D, Hanna MG. Mitochondrial disease: mutations and mechanisms. Neurochem Res. 2004;3:589-600.
41 Wallace DC. A mitochondrial paradigm of metabolic and degenerative diseases, ageing, and cancer: a dawn for evolutionary medicine. Ann Rev Genet. 2005;39:359-407.
42 Kagan T, Davis C, Lin L, et al. Coenzyme Q10 can in some circumstances block apoptosis, and this effect is mediated through mitochondria. Ann NY Acad Sci. 1999;887:31-47.
43 Crestanello JA, Doliba NM, et al. Effect of coenzyme Q10 supplementation on mitochondrial function after myocardial ischemia reperfusion. J Surg Res. 2002;102(2):221-228.
44 Buchner DM, Wagner EH. Preventing frail health. Clin Geriatr Med. 1992;8(1):1-17.
45 Cobon GS, Verrills N, Papakostopoulos P, et al. The proteomics of ageing. Biogerontology. 2002;3(1/2):133-136.
46 Tinetti ME, Speechley M, Ginter SF. Risk factors for falls among elderly persons living in the community. N Engl J Med. 1988;319(26):1701-1707.
47 Kallman DA, Plato CC, Tobin JD. The role of muscle loss in the aged-related decline of grip strength: cross-sectional and longitudinal perspectives. J Gerontol. 1990;45(3):M82-M88.
48 Rakowski W, Mor V. The association of physical activity with mortality among older adults in the Longitudinal Study of Ageing (1984–1988). J Gerontol. 1992;47(4):M122-M129.
49 Dela F, Kjaer M. Resistance training, insulin sensitivity and muscle function in the elderly. Essays Biochem. 2006;42:75-88.
50 Konerman SG. Endocrine aspects of ageing. New York: Elsevier, 1982.
51 Baumgartner RN, Koehler KM, Gallagher D, et al. Epidemiology of sarcopenia among the elderly in New Mexico. Am J Epidemiol. 1998;147(8):755-763.
52 Bonnefoy M, Constans T, Ferry M. Influence of nutrition and physical activity on muscle in the very elderly. Presse Med. 2000;29(39):2177-2182.
53 Pierson RNJr. Body composition in ageing: a biological perspective. Curr Opin Clin Nutr Metab Care. 2003;6(1):15-20.
54 Tonino RP, Minaker KL, Rowe JW. Effect of age on systemic delivery of oral glucose in men. Diabetes Care. 1989;12(6):394-398.
55 Harris MI. Epidemiology of diabetes mellitus among the elderly in the United States. Clin Geriatr Med. 1990;6(4):703-719.
56 Hales CN. Non-insulin-dependent diabetes mellitus. Br Med Bull. 1997;53(1):109-122.
57 Hu FB, Manson JE, Stampfer MJ, et al. Diet, lifestyle, and the risk of type 2 diabetes mellitus in women. N Engl J Med. 2001;345(11):790-797.
58 Chuo AM, Lim JK. Thyroid dysfunction in elderly patients. Ann Acad Med Singapore. 2003;32(1):96-100.
59 Mariotti S, Franceschi A, Cossarizza A, et al. The ageing thyroid. Endocr Rev. 1995;16(6):686-715.
60 Weissel M. Disturbances of thyroid function in the elderly. Wien Klin Wochenschr. 2006;118(1/2):16-20.
61 Livingston EH, Hershman JM, Sawin CT, et al. Prevalence of thyroid disease and abnormal thyroid tests in older hospitalized and ambulatory patients. J Am Geriatr Soc. 1987;35:109-114.
62 Vanderpump MPJ, Tunbridge WMG, French JM, et al. The incidence of thyroid disorders in the community: a twenty year follow-up of the Whickham survey. Clin Endocrinol. 1995;43:55-68.
63 Rose NR. Thymus function, ageing and autoimmunity. Immunol Lett. 1994;40(3):225-230.
64 Prelog M. Ageing of the immune system: a risk factor for autoimmunity? Autoimmun Rev. 2006;5(2):136-139.
65 Bunevicius R, Kazanavicius G, et al. Effects of thyroxine as compared with thyroxine plus triiodothyronine in patients with hypothyroidism. N Engl J Med. 1990;340:424-429.
66 Hall JE. Neuroendocrine changes with reproductive ageing in women. Semin Reprod Med. 2007;25(5):344-351.
67 Wise PM, Krajnak KM, Kashon ML. Menopause: the ageing of multiple pacemakers. Science. 1996;273(5271):67-70.
68 Ginsberg TB. Ageing and sexuality. Med Clin North Am. 2006;90(5):1025-1036.
69 Lambert SM, Masson P, Fisch H. The male biological clock. World J Urol. 2006;24(6):611-617.
70 Lobo RA. Postmenopausal hormones and coronary artery disease: potential benefits and risks. Climacteric. 2007;10(Suppl 2):21-26. Review.
71 Vitale C, Miceli M, Rosano GM. Gender-specific characteristics of atherosclerosis in menopausal women: risk factors, clinical course and strategies for prevention. Climacteric. 2007;Suppl 2:16-20.
72 Barrett-Connor E, Grady D, Stefanick ML. The rise and fall of menopausal hormone therapy. Ann Rev Pub Health. 2005;26:115-140.
73 Faber A, Bouvy ML, Loskamp L, et al. Dramatic change in prescribing of hormone replacement therapy in The Netherlands after publication of the Million Women Study: a follow-up study. Br J Clin Pharmacol. 2005;60(6):641-647.
74 Moskowitz D. A comprehensive review of the safety and efficacy of bioidentical hormones for the management of menopause and related health risks. Altern Med Rev. 2006;11(3):208-223.
75 Schatzl G, Madersbacher S, Temml C, et al. Serum androgen levels in men: impact of health status and age. Urology. 2003;61(3):629-633.
76 Anawalt BD, Merriam GR. Neuroendocrine ageing in men. Andropause and somatopause. Endocrinol Metab Clin North Am. 2001;30(3):647-669.
77 Harman SM, Tsitouras PD. Reproductive hormones in ageing men. II. Basal pituitary gonadotropins and gonadotropin responses to luteinizing hormone-releasing hormone. J Clin Endocrinol Metab. 1982;54(3):547-551.
78 Chahal HS, Drake WM. The endocrine system and ageing. J Pathol. 2007;211(2):173-180. Review.
79 Chew KK, Stuckey B, Bremner A, et al. Male erectile dysfunction: its prevalence in Western Australia and associated sociodemographic factors. J Sex Med. 2008;5(1):60-69.
80 Fink RI, Kolterman OG, Olefsky JM. The physiological significance of the glucose intolerance of ageing. J Gerontol. 1984;39(3):273-278.
81 Spark RF. Testosterone, diabetes mellitus, and the metabolic syndrome. Curr Urol Rep. 2007;8(6):467-471. Review.
82 Morley JE. Testosterone and behavior. Clin Geriatr Med. 2003;19(3):605-616. Review.
83 Alexander GM, Swerdloff RS, Wang C, et al. Androgen behavior correlations in hypogonadal and eugonadal men: cognitive abilities. Horm Behav. 1998;33:85-94.
84 Urban RJ, Bodenburg YH, Gilkison C, et al. Testosterone administration to elderly men increases skeletal muscle strength and protein synthesis. Am J Physiol. 1995;269:E820-E826.
85 Tenover JS. Androgen administration to ageing men. Endocrinol Metab Clin North Am. 1994;23:877-892.
86 Emmelot-Vonk MH, Verhaar HJ, Nakahi Pour HR, et al. Effect of testosterone supplementation on functional mobility, cognition, and other parameters in older men: a randomized controlled trial. JAMA. 2008;299(1):39-52.
87 Vigna GB, Bergami E. Testosterone replacement, cardiovascular system and risk factors in the ageing male. J Endocrinol Invest. 2005;28:69-74.
88 Gooren LJ. Androgens and male aging: current evidence of safety and efficacy. Asian J Androl. 2010;12(2):136-151.
89 Herbert J. The age of dehydroepiandrosterone. Lancet. 1995;345:1193-1194.
90 Ravaglia G, Forti P, Maioli F, et al. The relationship of dehydroepiandrosterone sulfate (DHEAS) to endocrine: metabolic parameters and functional status in the oldest-old. Results from an Italian study on healthy free-living over-ninety-year-olds. J Clin Endocrin Metab. 1996;81(3):1173-1178.
91 Barrett-Connor E, Goodman-Gruen D. The epidemiology of DHEAS and cardiovascular disease. Ann NY Acad Sci. 1995;774:259-270.
92 Wolf OT, Kirschbaum C. Actions of dehydroepiandrosterone and its sulfate in the central nervous system: effects on cognition and emotion in animals and humans. Brain Res Rev. 1999;30(3):264-288.
93 Brooke AM, Kalingag LA, Miraki-Moud F, et al. Dehydroepiandrosterone improves psychological well-being in male and female hypopituitary patients on maintenance growth hormone replacement. Clin Endocrinol Metab. 2006;91(10):3773-3779.
94 Watson RR, Huls A, Araghinikuam M, et al. Dehydroepiandrosterone and diseases of ageing. Drugs Ageing. 1996;9(4):274-291.
95 Yen SS, Morales AJ, Khorram O. Replacement of DHEA in ageing men and women. Potential remedial effects. Ann NY Acad Sci. 1995;774:128-142.
96 Morales AJ, Nolan JJ, Nelson JC, et al. Effects of replacement dose of dehydroepiandrosterone in men and women of advancing age. J Clin Endocrinol Metab. 1994;78(6):1360-1367.
97 Morales AJ, Haubrich RH, Hwang JY, et al. The effect of six months treatment with a 100 mg daily dose of dehydroepiandrosterone (DHEA) on circulating sex steroids, body composition and muscle strength in age-advanced men and women. Clin Endocrinol (Oxf). 1998;49(4):421-432.
98 Yamada Y, Sekihara H, Omura M, et al. Changes in serum sex hormone profiles after short-term low-dose administration of dehydroepiandrosterone (DHEA) to young and elderly persons. Endocr J. 2007;54(1):153-162.
99 Genazzani AR, Pluchino N, Begliuomini S, et al. Long-term low-dose oral administration of dehydroepiandrosterone modulates adrenal response to adrenocorticotropic hormone in early and late postmenopausal women. Gynaecol Endocrinol. 2006;22(11):627-635.
100 Clayton P, Gleeson H, Monson J, et al. Growth hormone replacement throughout life: insights into age-related responses to treatment. Growth Horm IGF Res. 2007;17(5):369-382.
101 Corpas E, Harman SM, Blackman MR. Human growth hormone and human ageing. Endocr Rev. 1993;14(1):20-39.
102 Sievers C, Schneider HJ, Stalla GK. Insulin-like growth factor-1 in plasma and brain: regulation in health and disease. Front Biosci. 2008;13:85-99.
103 Blackman MR. Pituitary hormones and ageing. Endocrinol Metab Clin North Am. 1987;16(4):981-994.
104 Merriam GR, Schwartz RS, Vitiello MV. Growth hormone-releasing hormone and growth hormone secretagogues in normal ageing. Endocrine. 2003;22(1):41-48.
105 Lamberts SW. The somatopause: to treat or not to treat? Horm Res. 2000;53(Suppl 3):42-43.
106 Rudman D, Feller AG, Nagraj HS, et al. Effects of human growth hormone in men over 60 years old. N Engl J Med. 1990;323(1):1-6.
107 Papadakis MA, Grady D, Black D, et al. Growth hormone replacement in healthy older men improves body composition but not functional ability. Ann Intern Med. 1996;124(8):708-716.
108 Taaffe DR, Pruitt L, Reim J, et al. Effect of recombinant human growth hormone on the muscle strength response to resistance exercise in elderly men. J Clin Endocrinol Metab. 1994;79(5):1361-1366.
109 Vittone J, Blackman MR, Busby-Whitehead J, et al. Effects of single nightly injections of growth hormone-releasing hormone (GHRH 1–29) in healthy elderly men. Metabolism. 1997;46(1):89-96.
110 Bach MA, Rockwood K, Zetterberg C, et al. The effects of MK-0677, an oral growth hormone secretagogue, in patients with hip fracture. J Am Geriatr Soc. 2004;52(4):516-523.
111 Poehlman ET, Copeland KC. Influence of physical activity on insulin-like growth factor in healthy younger and older men. J Clin Endocrin Metab. 1990;71(6):1468-1473.
112 Corpas E, Harman SM, Pineyro MA, et al. Growth hormone (GH)-releasing hormone (1–29) twice daily reverses the decreased GH and insulin-like growth factor-I levels in old men. J Clin Endocrin Metab. 1992;75(2):530-535.
113 Merriam GR, Buchner DM, Prinz PN, et al. Potential applications of GH secretagogs in the evaluation and treatment of the age-related decline in growth hormone secretion. Endocrine. 1997;7(1):49-52.
114 Perls TT, Reisman NR, Olshansky SJ. Provision or distribution of growth hormone for ‘antiageing’: clinical and legal issues. JAMA. 2005;294(16):2086-2090.
115 Willett W. Balancing lifestyle and genomics research for disease prevention. Science. 2002;296:695-698.
116 Vitetta L, Anton B, Cortizo F, et al. Mind–body medicine: stress and its impact on overall health and longevity. Ann NY Acad Sci. 2005;1057:492-505.
117 Vitetta L, Anton B. Lifestyle and nutrition, caloric restriction, mitochondrial health and hormones: scientific interventions for anti-aging. Clin Interv Aging. 2007;2(4):537-543.
118 Hassed C. New frontiers in medicine: the body as the shadow of the soul. Melbourne: Michelle Anderson Publishing, 2000.
119 Selye H. The stress of life. New York: McGraw-Hill, 1987.
120 Lin J, Dhabhar FS, Wolkowitz O, et al. Pessimism correlates with leukocyte telomere shortness and elevated interleukin-6 in post-menopausal women. Brain Behav Immun. 2009;23(4):446-449.
121 Epel E, Daubenmier J, Moskowitz JT, et al. Can mediation slow rate of cellular aging? Cognitive stress, mindfulness and telomeres. Ann NY Acad Sci. 2009;1172:34-53.
122 Jackson AA. Integrating the ideas of life course across cellular, individual, and population levels in cancer causation. J Nutr. 2005;135(Suppl 12):S2927-S2933.
123 Trichopoulou A, Costacou T, Bamia C, et al. Adherence to a Mediterranean diet and survival in a Greek population. N Engl J Med. 2003;348(26):2599-2608.
124 Meyer TE, Kovacs SJ, Ehsani AA, et al. Long-term caloric restriction ameliorates the decline in diastolic function in humans. J Am Coll Cardiol. 2006;47(2):398-402.
125 McCay CM, Cromwell MF, Maynard LA. The effect of retarded growth upon the length of life span and upon the ultimate body size. J Nutr. 1935;10:63-79.
126 Weindruch R, Keenan KP, Carney JM, et al. Caloric restriction mimetics: metabolic interventions. J Gerontol A Biol Sci Med Sci. 2001;56(Spec No 1):20-33.
127 Weindruch R, Sohal RS. Seminars in medicine of the Beth Israel Deaconess Medical Center. Caloric intake and ageing. N Engl J Med. 2001;337:986-994.
128 Holloszt JO. Mortality rate and longevity of food-restricted exercising male rats: a reevaluation. J Appl Physiol. 1997;82(2):399-403.
129 Lane MA, Black A, Handy A, et al. Caloric restriction in primates. Ann NY Acad Sci. 2001;928:287-295.
130 Fernandes G, Yunis EJ, Good RA. Suppression of adenocarcinoma by the immunological consequences of calorie restriction. Nature. 1976;263:504-507.
131 Fernandes G, Good RA. Inhibition by restricted calorie diet of lymphoproliferative disease and renal damage in MRL/lpr mice. Proc Natl Acad Sci. 1984;81:6144-6148.
132 Kubo C, Day NK, Good RA. Influence of early or late dietary restriction on life span and immunological parameters in MRL/Mp–lpr/lpr mice. Proc Natl Acad Sci USA. 1984;81:5831-5835.
133 Engelman RW, Day NK, Chen RF, et al. Calorie consumption level influences development of C3H/Ou breast adenocarcinoma with indifference to calorie source. Proc Soc Exp Biol Med. 1990;193:23-30.
134 Sarkar NH, Fernandes G, Telang NT, et al. Low-calorie diet prevents the development of mammary tumors in C3H mice and reduces circulating prolactin level, murine mammary tumor virus expression, and proliferation of mammary alveolar cells. Proc Natl Acad Sci USA. 1982;79:7758-7762.
135 Everitt AV, Le Couteur DG. Life extension by calorie restriction in humans. Ann NY Acad Sci. 2007;1114:428-433.
136 Duan W, Mattson MP. Dietary restriction and 2-deoxyglucose administration improve behavioral outcome and reduce degeneration of dopaminergic neurons in models of Parkinson’s disease. J Neurosci Res. 1999;57:195-206.
137 Zhu H, Guo Q, Mattson MP. Dietary restriction protects hippocampal neurons against the death-promoting action of presenilin-1 mutation. Brain Res. 1999;842:224-229.
138 Mattson MP. Neuroprotective signaling and the ageing brain: take away my food and let me run. Brain Res. 2000;886:47-53.
139 Ingram DK, Weindruch R, Spangler EL, et al. Dietary restriction benefits learning and motor performance of aged mice. J Gerontol. 1987;42:78-81.
140 Moroi-Fetters SE, Mervis RF, London ED, et al. Dietary restriction suppresses age-related changes in dendritic spines. Neurobiol Ageing. 1989;10:317-322.
141 Roth GS, Lane MA, Ingram DK, et al. Biomarkers of caloric restriction may predict longevity in humans. Science. 2002;297:811.
142 Heilbronn LK, de Jonge L, Frisard MI, et al. Effect of 6-month calorie restriction on biomarkers of longevity, metabolic adaptation, and oxidative stress in overweight individuals: a randomized controlled trial. JAMA. 2006;295(13):1539-1548.
143 Baur JA, Sinclair DA. Therapeutic potential of resveratrol: the in vivo evidence. Nat Rev Drug Discov. 2006;5(6):493-506.
144 Guarente L, Kenyon C. Genetic pathways that regulate ageing in model organisms. Nature. 2000;408:255-262.
145 Howitz KT, Bitterman KJ, Cohen HY, et al. Small molecule activators of sirtuins extend Saccharomyces cerevisiae lifespan. Nature. 2003;425:191-196.
146 Kirkland JL. The biology of senescence: potential for prevention of disease. Clin Geriatr Med. 2002;18(3):383-405.
147 Wiley J, Camacho T. Lifestyle and future health: evidence from the Alameda County Study. Prevent Med. 1980;9:1-21.
148 Seeman T, Kaplan G, Knudsen L, et al. Social network ties and mortality among the elderly in the Alameda County Study. Am J Epidemiol. 1987;126:714-723.
149 Breslow L, Breslow N. Health practices and disability: some evidence from Alameda County. Prevent Med. 1993;22:86-95.
150 Vaillant G, Mukamal K. Successful ageing. Am J Psych. 2001;158(6):839-847.