Chapter 95 Adjuncts to hepatic resection
Ultrasound and emerging guidance systems
Overview
Hepatic surgery performed without a parenchyma-sparing policy carries relevant risks for patient survival because of postoperative liver failure; for this reason, the application of hepatic surgery is limited (Vauthey et al, 2004). In particular, the coexistence of liver cirrhosis in most patients with hepatocellular carcinoma (HCC) has a considerable adverse effect on the surgical results (see Chapter 80), and recent series are still associated with mortality rates higher than 5% (Liu et al, 2004) and with resectability rates of 30% to 35%, which is relatively low (Sotiropoulos et al, 2006). For this reason, and for the broadening of ultrasound (US)-guided percutaneous therapies (N’Kontchou et al, 2009), the surgical treatment of HCC has surrendered its role as first choice treatment for all patients and is now reserved only for those patients with a normal bilirubin level, no signs of portal hypertension, and a single, small HCC (Bruix & Llovet, 2009).
Conversely, for colorectal cancer liver metastases (CLM), surgical treatment remains the gold standard (see Chapter 81A). The definition of resectability has shifted from a focus on tumor characteristics, such as tumor number and size, to determination of whether both intrahepatic and extrahepatic disease can be completely resected, and whether such an approach is appropriate from an oncologic standpoint. Therefore limiting surgery in the case of CLM has increasingly focused on the technical feasibility, although not entirely (Minagawa et al, 2000; Torzilli et al, 2009b). In this sense, progress with chemotherapeutic regimens has improved the resectability rate (Wicherts et al, 2008), although it remains generally around 15% to 25% of patients (Garcea et al, 2008). A major limit of resectability is the need for major hepatectomies, which still represents a significant factor impacting the risk of perioperative mortality and morbidity (Schroeder et al, 2006; Cucchetti et al, 2006). Portal vein embolization (PVE) was devised with the double aim of increasing the safety of major liver resection and allowing more patients to benefit from the surgical treatment (Jaeck et al, 2004; see Chapter 93A, Chapter 93B ). However, liver failure occurs in 33% of patients who did not undergo PVE, and it still happens in 10% of those who did (Hemming et al, 2003). As with HCC, alternative therapies have been devised for treating CLM, such as percutaneous interstitial treatment (Siperstein et al, 2007). Although these new therapies initially enabled patients otherwise excluded from the surgical program to be treated, the apparently good results obtained have generated confusion in the scientific community as to which treatment modalities should be chosen to manage these patients.
Imaging techniques have been introduced as aids for surgeons in performing liver resection. In fact, since the early 1980s, intraoperative ultrasonography (IOUS) has been used in hepatic surgery, initially in patients with liver cirrhosis (Makuuchi et al, 1980; see Chapter 21). Now liver resections can be carried out with very low mortality, even if cirrhosis is present (Imamura et al, 2003; Torzilli et al, 1999a). There are many reasons for this improvement, but a large part can be attributed to better operative technique, including IOUS. Indeed, US guidance allowing the so-called radical but conservative policy (Torzilli et al, 2005a, 2006b) makes feasible an alternative to major hepatectomies, having the aim of reducing the need for major parenchymal removal while keeping an acceptable oncologic radicality. This policy has allowed resectability rates from outpatient evaluation to hepatectomy of 68% and 69% for HCC and CLM, respectively (Torzilli et al, 2008b, 2009b). Recently, the demonstration of feasibility and efficacy of contrast-enhanced US performed intraoperatively (CE-IOUS) has furthermore stressed the relevance of IOUS guidance during liver surgery (Torzilli et al, 2007b, 2008a).
Technical Aspects
Probe Selection
High-frequency (7.5 to 10 MHz) echoprobes are commonly recommended to perform IOUS, because they allow a higher spatial resolution than those that operate at lower frequencies (3.5 to 5 MHz); however, the latter are also useful, at least along the initial exploration, providing a better panoramicity that helps compensate for the lower spatial resolution (Fig. 95.1). On the other hand, it should be pointed out that often higher spatial resolution at the superficial portion of the liver is less important than the overall visibility of the deeper structures, because the most superficial portions are also those appreciable with palpation. In the latter condition, once a nodule is slightly visible at IOUS but is not palpable, especially in a cirrhotic liver, a surgical glove filled with deaerated sterile water can be positioned between the probe and the liver surface for making the lesion more visible (Fig. 95.2). Lower frequency echoprobes are also useful for allowing CE-IOUS; therefore, having both low- and high-frequency probes would be the optimal solution, and new probes with wider ranges of frequencies (3.5 to 10 MHz) are expected.
The most frequently used probes are the T-shaped (Fig. 95.3), interdigital, and microconvex probes (Fig. 95.4). The microconvex probe represents the best compromise among all the aforementioned requirements. Indeed, the T-shaped probe, although it remains more stable and is associated with higher image resolution, has a lower ratio between lateral length and US scanning window than the microconvex probe. Linear transducers with enlarged scanning windows are under development that combine the stability of the linear probes and their higher image resolution with larger scanning windows and a limited volume (Fig. 95.5). When evaluating probes, another aspect that should be considered is the possibility of using them for surgical maneuvers, as described below; newer probes are being designed from this perspective (see Fig. 95.5).
Color Doppler imaging, and particularly new, more sensitive color-flow modes, are progressively assuming greater relevance in hepatic surgery. For example, e-flow intraoperative US (EF-IOUS) is useful for studying the inflow and outflow of the liver intraoperatively (Fig. 95.6), and it shows the flow modifications during surgical maneuvers; this represents crucial data for allowing surgical strategies that would not be feasible otherwise. These promising new technologies will be discussed in this chapter.
CE-IOUS is one of the latest advancements in intraoperative application of US, but dedicated probes built to carry out this diagnostic modality in direct contact with the targeted organ are few; furthermore, CE-IOUS requires high-quality digital US machines. We use the Alpha 10 (Aloka, Tokyo; Fig. 95.7), which is equipped with a convex probe working at 3- to 6-MHz frequency and at 1.88- to 3.76-MHz harmonic frequency (see Fig. 95.1). The contrast agent used, SonoVue (Bracco Imaging, Milan, Italy), consists of sulfur hexafluoride microbubbles stabilized by a phospholipid shell; the anesthesiologist injects 4.8 mL of SonoVue intravenously for exploration through a peripheral vein. The purpose of the contrast enhancement is to evaluate the lesion’s vascularity, aid in the characterization of those eventually found at IOUS, and to detect new ones, taking advantage of the enhanced brightness of the liver parenchyma, especially along the delayed phases (Fig. 95.8). New liver-specific contrast agents, such as those containing perfluorobutane (Sonazoid, Daiichi Sankyo, Tokyo), will soon become commercially available for clinical use; however, initially, these are being used clinically and only in Japan. This contrast agent, which has properties similar to liver-specific contrast medium used in magnetic resonance imaging (MRI), could improve nodule differentiation (Kudo, 2007) and detection (Nakano et al, 2008).
Preparation for Liver Exploration
The US machine should be positioned opposite the first operator, who should be able to simultaneously view both the screen and the operative field (Fig. 95.9). The screen must be large enough to allow optimal visibility at that distance, and lights should be positioned with caution so as not to interfere with the visibility of the US screen. An assistant familiar with the US keyboard should stay beside it, or a transparent and sterile covering pad should be available that allows the lead member of the surgical team to handle the keyboard directly (see Figs. 95.7 and 95.9).
After entering the abdominal cavity, liver mobilization, division of the round and falciform ligaments, and division of adhesions to free the anterosuperior and inferior surfaces of the liver are the steps that should precede liver exploration with IOUS (Fig. 95.10). Of course, adhesions from the tumor to other organs or structures should not be approached, as they may represent areas of tumor infiltration; in this situation, IOUS can help exclude or confirm tumor invasion, which could change the surgical strategy.
Ultrasound Liver Anatomy
Solid knowledge of the liver anatomy is required to perform IOUS properly (see Chapter 1B). For surgical anatomy, the Brisbane terminology is considered here (Pang, 2002). The three main hepatic veins are readily identified at their junction with the inferior vena cava (IVC), positioning the probe at this level and tilting it upward once the confluence of the hepatic veins into the IVC is recognized. Then, gently withdrawing the probe, the hepatic vein paths can be traced into the liver. Hepatic veins appear as echo-free zones in the liver parenchyma, and the vessel wall is invisible or appears as a thin, hyperechogenic line (Fig. 95.11A); the walls of hepatic veins can be thicker in the cirrhotic liver, and their lumens thinner, as a result of the stiffness of the diseased liver (Fig. 95.11B).
The portal vein branches can be followed by initially positioning the probe horizontally above the inferior portion of segment IV (see Fig. 95.10) to visualize the first-order bifurcation (Fig. 95.12), then the first-, second-, and third-order portal branches can be followed. Because of the existence of the Glisson capsule, the portal pedicles—which include portal vein branches, arteries, and bile ducts—have thicker vessel walls compared with the hepatic veins, and for this reason, they appear at IOUS as echo-free zones surrounded by a thicker hyperechogenic layer (see Fig. 95.11); furthermore, other parallel, thinner vascular structure are visible, which are the arteries (Fig. 95.13), and the bile ducts of the glissonian triad are also visible (Fig. 95.14). The newer, more sensitive color-flow modalities, such as EF-IOUS, help in this differentiation by allowing the recognition of arterial and portal elements that also lie within thin glissonian sheaths (Fig. 95.15); however, in principle, distinction between hepatic veins and portal branches should be based not only on their appearance but mainly on their anatomy. Indeed, in the cirrhotic liver, as already mentioned, the vessel wall of the hepatic vein could be thicker and not easily differentiated from a peripheral portal branch. Following the portal pedicles at the sectional, segmental, and subsegmental level, and positioning them in relation to the hepatic veins, it is possible to precisely define the location of the IOUS target in terms of sections and segments (Fig. 95.16).
The appearance of the bile ducts on IOUS is worth mentioning because of their peculiarity. Whereas normally they result as thin echo-free zones in the glissonian triad (see Fig. 95.14), once dilated, they appear as more evident echo-free zones with a serpiginous path (Fig. 95.17). The element that is difficult to recognize in the IOUS study of the bile ducts is their segmental anatomy; bifurcation of sectional and segmental ducts is closer to the hilum, compared with the portal branches (Fig. 95.18), and because of that, it is possible to visualize more than one segmental bile duct with one scan; however, with enough US background, IOUS can allow exact definition of the bile duct anatomy, not only in pathologic conditions but also in the normal state. This possibility also allows assessment of variations in the normal anatomy, such as confluence of the right posterior sectional bile duct into the left hepatic duct (Fig. 95.19), which is clearly critical information, particularly if a left hepatectomy is planned.
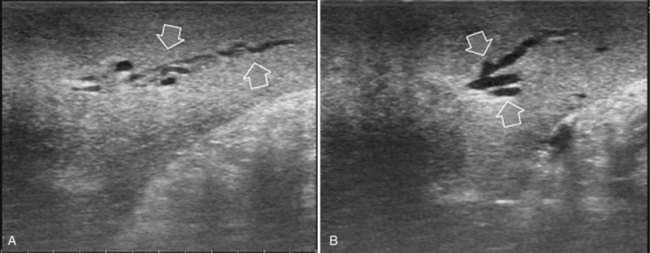
FIGURE 95.17 A and B show the dilation of segmental bile ducts, which assume a serpiginous path (arrows).
Indications
Liver Exploration
Detection and differentiation are the two main targets of IOUS exploration of the liver. The hard and irregular surface of cirrhotic livers makes difficult the detection of small nodules by palpation; conversely, IOUS allows the detection of new lesions in around 30% of cases (Kokudo et al, 1996), although most of the nodules detected by IOUS in the cirrhotic liver are not tumors but more often are regenerative nodules. In this way, IOUS introduces the risk of overestimating tumor stage (Fig. 95.20). Indeed, except for those nodules with a mosaic pattern evident on US (Fig. 95.21A), which are malignant in 84% of cases, only 24% to 30% of hypoechogenic (dark) nodules (Fig. 95.21B) and 0% to 18% of those hyperechogenic (bright) nodules (Fig. 95.21C) are neoplasms (Kokudo et al, 1996; Takigawa et al, 2001).
To overcome this problem, even biopsy seems to be inadequate. The only nodule that can be easily differentiated intraoperatively from HCC or liver metastases is the small hemangioma, often discovered primarily at IOUS (see Chapter 79A, Chapter 79B ); it displays a typical ultrasonographic pattern, and when compressed, it changes in size and appearance (Fig. 95.22; see Chapter 13). The problem of differentiating benign from malignant lesions on IOUS exploration becomes crucial. Some attempts are now ongoing using elastography, which should allow lesion differentiation based on tissue stiffness expressed on the IOUS screen and indicated by different colors (Fig. 95.23); however, to date, limited data are available to describe its use in this application. In the next section, it will be shown how CE-IOUS will play an increasingly important role in this context and will be even more relevant in the near future.
Rather than differentiation, in the case of CLM, their clue is their accurate detection, particularly in the event of multinodular hepatic involvement. Indeed, in patients with CLM, the detection of any tiny nodule undiscovered preoperatively becomes crucial for attempting a reduction of the still high postoperative early recurrence rate (Yoshidome et al, 2008). From 10% to 40% of patients with colon cancer have nonpalpable CLM (Agrawal et al, 2006; Machi et al, 1991); as a consequence, IOUS exploration of the liver remains crucial, and CE-IOUS seems able to enhance its role in this context.
Contrast-Enhanced Intraoperative Ultrasonography
Hepatocellular Carcinoma
CE-IOUS is now used for characterizing the new lesions of HCC eventually detected at IOUS (Torzilli et al, 2007b). The rationale is to check the vascular pattern during contrast enhancement of each new lesion. Because it is very important to identify the arterial vascularization in HCC, which can take 20 to 30 seconds, each nodule must be carefully evaluated, and this necessity demands multiple injections in the presence of multiple nodules. This requirement may be obviated by the new liver-specific contrast agents. Using these, the contrast enhancement remains visible from several minutes to even hours after injection, and CE-IOUS should become easier to use and will likely be more effective for detection of HCC.
Tumor vascularity as a criterion for differentiating regenerative or dysplastic nodules from HCC correlates with the histologic evidence of a progressive increase in unpaired arteries from dysplastic to neoplastic nodules in a cirrhotic liver (Roncalli et al, 1999). Certainly, the pattern of vascular enhancement by itself is not sufficient for differentiating malignant from nonmalignant nodules with 100% specificity. Indeed, although percutaneous contrast-enhanced US (CE-US) provides differential diagnosis of hepatic nodules based on their vascular pattern with 95% specificity (Quaia et al, 2004), intraoperatively there is the need to differentiate lesions smaller than 1 cm. For these nodules, the vascularity as a criterion for differential diagnosis is less specific, although some improvements compared with conventional IOUS could be expected. Therefore, in the early 1990s, attempts were made to apply CE-IOUS using carbon dioxide as a contrast material, but the need for arterial catheterization made this technique too invasive (Takada et al, 1990). In my preliminary experience, CE-IOUS has provided remarkable findings, either by adding information on nodular vascularity in patients with HCC, or by detecting nodules that were not visible at IOUS, in patients with CLM (Torzilli et al, 2004).
For patients undergoing operation for HCC, my colleagues and I have introduced a classification of the enhancement pattern of lesions seen at CE-IOUS, upon which the surgical decision making is established (Fig. 95.24; Torzilli et al, 2007b). In brief, any pathologic lesion should appear as hypoechogenic at the late phases, with or without inner vessels and with or without an arterial phase, in which it is enhanced prior to the remnant liver parenchyma. Lesions with this type of appearance should be removed (Fig. 95.25). Those lesions that disappear once the contrast enhances the liver are not considered neoplastic and are not removed (Fig. 95.26). With these criteria, we obtained with CE-IOUS a specificity of 69% (Torzilli et al, 2007b). This value is probably not that high, especially when compared with that reported for CE-US (Quaia et al, 2004); however, as mentioned before, the small size of the lesions targeted for CE-IOUS study could explain this discrepancy. For these tiny nodules, the neovascularity as a criterion for differentiation between malignant and benign lesions has limits that are independent from the method we use; therefore CE-IOUS can be helpful in a certain percentage of nodules but not in all, although the rate of 69% specificity is encouraging, as it means that we can provide proper information with this new technique in 7 of 10 lesions we detect at laparotomy. For those lesions remaining, even histology may be lacking, as we know no common agreement exists among Western and Eastern pathologists on the definition of early HCC and dysplastic lesions (International Consensus Group for Hepatocellular Carcinoma, 2009). New perspective may be provided by a more extensive use of the new contrast agent, for now clinically available only in Japan (Kudo, 2007). This agent (Sonozoid) has a Kupffer phase, and it adds further criteria for differentiating those nodules detected at IOUS.
Colorectal Liver Metastases
Improving detection is the main target of CE-IOUS in the case of CLM. In the 1990s, half of the patients who underwent surgery for CLM had a surgical strategy modified by IOUS findings (Kane et al, 1994). More recently, progress in preoperative imaging has reduced this rate; in fact, some authors have reported that just 4% of the operative decision making is modified by IOUS (Sahani et al, 2004). Conversely, adding CE-IOUS to IOUS exploration modified operative decision making in 38% of patients with CLM (Torzilli et al, 2008a). If this discrepancy in the rates of modified surgical strategy among series is partially the result of different surgical approaches, particularly more parenchyma-sparing procedures, then probably CE-IOUS is playing a role, too. Indeed, with CE-US, the CLM shows the so-called “black hole” effect (Fig. 95.27). The metastastic nodule in the late phase, which lasts from 2 to 5 minutes after injection, remains unenhanced and black in comparison with the surrounding enhanced liver parenchyma (Fig. 95.28). This is particularly useful in those patients who undergo surgery after chemotherapy, in whom some nodules may not be well visualized by IOUS because of the histologic rearrangements induced by the chemotherapeutic agents. CE-IOUS allows better nodule visibility and has allowed my colleagues and me to detect 9% of adjunctive nodules (Torzilli et al, 2008a); therefore CE-IOUS application resulted in an increased sensitivity, and in patients with CLM, it appears to have an important role. The only condition under which it may not help is in the event of a bright liver at IOUS (Chen et al, 2008), which correlates with the degree of fatty change and accounts for 10% of our patients (Torzilli et al, 2008a). Indeed, in these circumstances, the visibility of CLM, generally hypoechogenic, is enhanced by the brightness of the surrounding liver parenchyma, mimicking the effect of the contrast enhancement (Fig. 95.29). As a confirmation of this, we never found new sites of disease at CE-IOUS in those patients having a bright liver at IOUS.
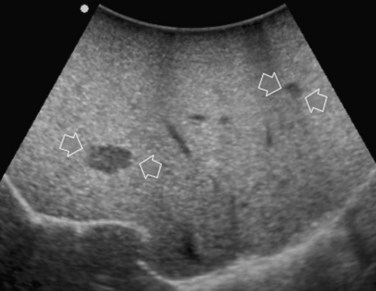
FIGURE 95.29 Clear visibility of two hypoechoic masses (arrows) in a so-called bright (fatty) liver.
For CLM, new perspectives could be obtained by using liver-specific contrast agents, which allow prolonged exploration. A preliminary experience of eight patients showed new lesions in two detected only at CE-IOUS (Nakano et al, 2008).
Care should be used in CLM patients who also have coexisting liver cysts, which may appear similar to the CLM along the delayed phases of contrast enhancement; however, the cysts should have been already mapped based on the preoperative imaging and should have been identified at exploration with conventional IOUS. Therefore, any new “black hole” detected in the liver in locations other than where cysts were eventually detected should be considered suspicious for malignancy. Liver cyst features at unenhanced IOUS have an anechoic content and a posterior enhanced echo (Fig. 95.30; see Chapter 13), and it must be taken into account that the anechoic pattern could be mimicked by tiny CLM (Fig. 95.31).
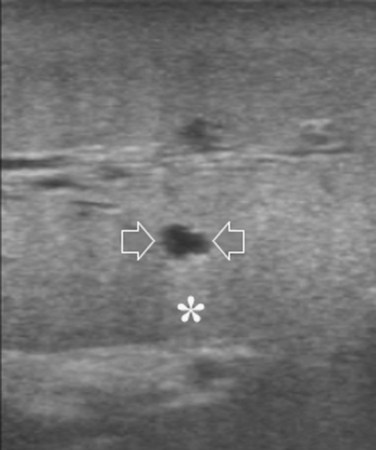
FIGURE 95.30 A small, simple hepatic cyst (arrows) with its typical posterior echo (asterisk) and the echo-free (black) content.
Other Intraoperative Techniques
Recently, imaging using indocyanine green (ICG) as a fluorescent source has been proposed (Ishizawa et al, 2009; Gotoh et al, 2009). Used in many centers to assess liver function, ICG is injected intravenously approximately 3 days before surgery and during laparotomy, and the liver is explored to detect any sign of fluorescence on its surface by means of a fluorescent imaging system that comprises a control unit and a camera. Once removed, the specimen is explored to detect undisclosed fluorescent areas. With this device, Ishizawa and colleagues (2009) disclosed 13 of 91 lesions with only an ICG fluorescent-imaging technique, obtaining a sensitivity of 100% for HCC and 93% for CLM. Although in a smaller series that included only patients with HCC, Gotoh and colleagues (2009) similarly showed that all the new lesions detected in the examined patients were visible only because of the ICG fluorescent-imaging technique.
Planning of the Surgical Strategy
IOUS exploration of the liver could have a significant impact on the surgical strategy. More recently, impact of IOUS on the operative decision making, when compared with that of preoperative imaging techniques, is reported to be around 4% to 7% (Cerwenka et al, 2003; Jarnagin et al, 2001). The problem of the impact of IOUS on the operative decision making depends on two main factors: the surgical policy of each specific team and the type of tumor. Indeed, the relatively low rates reported (Cerwenka et al, 2003; Jarnagin et al, 2001) are also influenced by the surgeon’s resection policy; when a considerable number of patients undergo major hepatectomies, new nodules detected by IOUS in the same hemiliver would still be resected, and the surgical strategy would not be modified.
Recently, it was shown that major hepatectomies are carried out in a minority of patients, even in extremely complex presentations (Torzilli et al, 2009b), simply because of the extensive use of IOUS guidance for achieving parenchyma-sparing resections; from this perspective, detection of new nodules is more likely to change the surgical strategy.
IOUS allows an accurate three-dimensional reconstruction of the relationship between the tumor, the portal branches, and the hepatic veins, which is a fundamental step in defining the proper surgical strategy. Definition of tumor-vessel relationships is relevant for planning the type of resection (Torzilli et al, 2008c, 2010a). IOUS allows the surgeon to easily recognize whether an HCC is separated by some normal parenchyma from the vessel, or if it is in contact with the vessel without invading its wall, or conversely whether the HCC is invading the vessel wall or is determining the proximal bile duct dilation, or if it is associated with a tumor thrombus. Similarly, definition of the relationship between a CLM and intrahepatic vascular structures is precisely defined by IOUS; once the vessel wall is found to be intact, the circumferential extent of the contact is relevant for planning a resection that spares the vessel in contact with the CLM (Torzilli et al, 2009b). Based on these features, the decision whether the vessel should be resected is considered, and a precise surgical strategy can be pursued.
Tumor in Contact with a Glissonian Pedicle
The glissonian pedicle may be spared when in contact with an encapsulated HCC with integrity of the vessel wall appreciable at IOUS without any sign of bile duct dilation (Fig. 95.32). Similarly, the pedicle can be spared when it is in contact with a CLM, the integrity of the vessel wall is confirmed, bile duct dilation is ruled out, and the contact extent is less than one third of the pedicle circumference (Fig. 95.33). In the presence of bile duct dilation, tumor thrombus, invasion of the vessel wall, and for CLM, contact wider than one third of pedicle circumference, the pedicle must be divided (Fig. 95.34). In these conditions, extension of the hepatectomy is required for complete tumor clearance.
Tumor in Contact with a Hepatic Vein
The hepatic vein may be spared when in contact with an encapsulated HCC with integrity of the vessel wall appreciable at IOUS (Fig. 95.35). Initially for the CLM, its contact was considered an indication for hepatic vein resection; however, the vein may be spared when it is in contact with a CLM, but at IOUS, the integrity of the vessel wall is confirmed, and the contact extension is less than two thirds of the vein circumference (Figs. 95.36 and 95.37). In the presence of tumor thrombus, invasion of the vessel wall, and contact wider than two thirds of the vein circumference in CLM, the hepatic vein must be divided (Fig. 95.38). In this condition, extension of the hepatectomy is not compulsorily considered, even if the hepatic vein is invaded at its caval confluence (the last 4 cm). Indeed, in this condition, an extension of the resection to the liver parenchyma theoretically drained by the hepatic vein to be resected is considered only if one of the following US signs is missing:
• Presence of accessory hepatic veins at IOUS as an inferior right hepatic vein in the presence of an invasion at the caval confluence of the right hepatic vein (Fig. 95.39)
• EF-IOUS showing hepatopetal blood flow in the feeding portal branch (Fig. 95.40), once the hepatic vein to be resected is clamped (Torzilli et al, 2006b) by means of encirclement, or more simply by vein compression at its extrahepatic route using a fingertip (Torzilli et al, 2007a)
• Communicating veins connecting adjacent hepatic veins (Fig. 95.41), these being more easily detectable using EF-IOUS to disclose their presence (Torzilli et al, 2010c).
Systematic Extended Right Posterior Sectionectomy
Systematic extended right posterior sectionectomy (SERPS) is a surgical technique that allows the systematic sparing of part of the right anterior section (see Fig. 95.38, C and D) in the presence of tumors with the presentation shown in Figure 95.42 (Torzilli et al, 2008c).
Eligibility Criteria
Patients suitable for SERPS are those with tumors showing one of three conditions:
1 Invasion of the right hepatic vein (RHV) is evident within 4 cm of the hepatocaval confluence, with other lesions involving segment VI and eventually segment VII (Fig. 95.42A).
2 Invasion of the RHV within 4 cm of the hepatocaval confluence is evident, without other lesions involving segment VI, without an inferior RHV (IRHV), and with hepatofugal portal blood flow at color-flow IOUS in the portal branch to segment VI (P6) when the RHV is clamped if not already occluded (Fig. 95.42B). In the event an IRHV is present, or, if not, when the flow direction in P6 remains hepatopetal, resection of segments VII and VIII together with the RHV is carried out (Makuuchi et al, 1987) rather than SERPS; therefore SERPS is applied as an alternative to resection of segments VII and VIII in those patients who do not have proper outflow for segment VI once the RHV is divided.
3 Contact with the right anterior glissonian sheath and a relationship with the right posterior section is evident, with at least one of the following features: contact with the right posterior section determining proximal bile duct dilation, vessel wall invasion, or, for CLM, contact wider than one third of the pedicle circumference (Fig. 95.42C).
Procedure
In the first two conditions, extension to the right anterior section is tailored to guarantee the complete removal of the tumor, and a dissection line is drawn on the left side of the RHV, which is also resected (see Fig. 95.42, A and B); flow direction in the right anterior portal branch at EF-IOUS is estimated as previously described, once the RHV is clamped, if it is not occluded (Fig. 95.43). The right anterior pedicle is not necessarily exposed on the liver cut surface. In the third condition, the extension of the resection into the right anterior section is tailored to preserve most of the parenchyma of segment VIII, the tract of the RHV at the hepatocaval confluence, and the left portion of segment V without division of the right anterior pedicle, which is exposed on the cut surface (see Fig. 95.42C).
Minimesohepatectomy
This procedure represents an alternative to the conventional mesohepatectomy in patients with tumors invading the middle hepatic vein (MHV) at its caval confluence; it consists of a limited resection, including the tract of the invaded vein without its reconstruction, sparing part of segment IV, and/or of the right anterior section as shown in Figure 95.44 and as described in the next section (Torzilli et al, 2010a).
Procedure
1 Reversal flow on EF-IOUS in the peripheral portion of the MHV, which suggests drainage through collateral circulation in the RHV/left hepatic vein (LHV), depending on the side of the MHV branch with reversal flow (Fig. 95.45)
2 Detectable communicating veins on EF-IOUS between the MHV and RHV and/or LHV and/or IVC (Fig. 95.46; as mentioned, this last feature could be also visible without compressing the vein)
3 Hepatopetal flow in segments V through VIII and/or the segment IV inferior portal branches (Fig. 95.47A)
If none of these findings is confirmed, and especially if hepatofugal flow direction in portal branches to segments V and/or VII and/or IV inferior is detected (Fig. 95.47B), hepatectomy should be extended to the area fed by those portal branches.
The posterior wall of the MHV, or of the tumor involving the paracaval portion, is used as a deep landmark for delimiting the resection area (Fig. 95.48). A crucial point for proper performance of the MMH in the presence of a communicating vein is to delineate a dissection plane that does not interrupt the communicating vein (see Fig. 95.48I).
Resection Guidance
Mobilization of the Liver (See Chapter 90A, Chapter 90B, Chapter 90C, Chapter 90D, Chapter 90E, Chapter 90F )
For right-sided segmentectomies, subsegmentectomies, or sectionectomies, the bare area is dissected, and the right hemiliver is mobilized, until the surgeon’s nondominant hand is positioned behind the hemiliver to sustain it and is passing over the resection area established by means of IOUS (Fig. 95.49). A slight mobilization of the right hemiliver, just dividing the triangular ligament and partially or completely dividing the bare area, will be accomplished for lesions located in segments V, VI, and the inferior portion of section VII (Fig. 95.50). Conversely, the right side of the retrohepatic IVC is reached for lesions located in the dorsal portions of segments VII and VIII (Fig. 95.51). If the lesion is located in the superior portion of segment VII or the dorsal portion of section VIII—that is, close to the hepatocaval confluence, in the last 4 cm—but it is not in contact with the hepatic veins and an anatomic segmental approach is not required, the hepatocaval ligament is not divided, and only the space between the RHV and the MHV is dissected to allow fingertip insertion. The caval confluence of the RHV is recognized, following the trajectory of the right inferior phrenic vein, which flows near the RHV at this level and which is a constant landmark (Fig. 95.52; Torzilli et al, 2006a).
If the lesion is still right-sided but makes contact with an HV at its caval confluence, or if it involves the paracaval portion of segment I or an anatomic segmental approach with hepatic vein exposure is not required, liver mobilization includes division of the retrohepatic caval ligament and exposure of the retrohepatic IVC; this proceeds until the area to be resected is under control of the surgeon’s nondominant hand, with the surgeon’s fingertip reaching over the most distal portion of the planned dissection plane, including the complete detachment (Figs. 95.52 and 95.53).
Anatomic Resections (See Chapter 90A, Chapter 90B, Chapter 90C, Chapter 90D, Chapter 90E, Chapter 90F, Chapter 92 )
Selection of an anatomic resection for CLM may not be relevant for long-term prognosis (Sarpel et al, 2009), and its use for HCC is still controversial (Cho et al, 2007; Eguchi et al, 2008; Kaibori et al, 2006; Regimbeau et al, 2002; Tanaka et al, 2008; Wakai et al, 2007); indeed, for the latter, no studies have been done that compare anatomic and nonanatomic resections in a truly randomized fashion; however, many reports show the long-term benefits of the anatomic approach (Cho et al, 2007; Eguchi et al, 2008; Kaibori et al, 2006; Regimbeau et al, 2002; Ueno et al, 2008; Wakai et al, 2007), and significant selection bias likely influences the reliability of the results (Hasegawa et al, 2005). The rationale for anatomic resection for HCC is based on its pattern of intrahepatic dissemination from the main lesion through the portal branches. As a consequence, the resected specimen should include at least the portal area perfusing the area of the liver that includes the lesion. Indeed, the superiority of the anatomic resection seems more evident in those subgroups with a single HCC larger than 2 cm in diameter (Eguchi et al, 2008) or with extranodular growth (Ueno et al, 2008), which should be those with higher risk of satellites and microvascular invasion.
Whether technical requirements for accomplishing truly anatomic sectionectomies and formal major hepatectomies are more uniformly accepted, the agreement on the requirements for defining a subsegmental resection as a genuine anatomic one is still unclear. Indeed, the recognition and demarcation of the segmental area to be removed in a fully anatomic fashion is still subject to debate. The only certainty is that the portal area feeding the tumor cannot be recognized visually on the liver surface if it is not associated with an interventional procedure for its precise disclosure. Almost all the proposed techniques require US guidance, especially in a cirrhotic liver, where there are generally wide variations and abnormalities in the extension of the segmental areas. The first procedure described in this was the systematic segmentectomy devised in early 1980s (Makuuchi et al, 1980), which consists of puncture of the portal branch feeding the tumor and subsequent injection of dye (Fig. 95.54); more recently, alternatives to this approach have been devised. In the following paragraphs, details concerning US-guided techniques and others for segmental and sectional resection will be provided.
Segmentectomies (See Chapter 92)
Puncture of the Portal Branch
The portal branch feeding the tumor to be resected is punctured under IOUS guidance, using a freehand technique or with a proper device, and 3 to 5 mL of indigo-carmine dye is injected into the vessel (see Fig. 95.54A). The stained area becomes evident on the liver surface, it is marked with electrocautery (see Fig. 95.54B), and the resection can be accomplished fully anatomically (see Fig. 95.54C). If the nodule is located between two adjacent segments, the two portal branches afferent to the area must be punctured and injected. In this case, the deepest and most dorsal vessel must be punctured first to prevent the air bubbles contained in the dye from disturbing the US detection and puncture of the other branch. The portal branch is punctured 1 to 2 cm distally from its origin to avoid dye reflux; the direction and velocity of the infusion are controlled by IOUS. To prolong the staining, the hepatic artery at the hilum is clamped before portal branch puncture. When there are numerous and thin vessels that should be punctured, or in the case of tumor thrombus in the segmental portal branch of the segment that should be removed, the dye is injected in the portal branches afferent to the adjacent segment; this is the so-called counterstaining technique (Takayama et al, 1991).
Compression of the Portal Branch
Initially used for tumors located in the left hemiliver (Torzilli & Makuuchi, 2004), more recently it has been successfully extended in its application to any segmental location (Torzilli et al, 2010b, 2011a) and even to a sectional portion of the liver (Torzilli et al, 2009a). Conceptually, the procedure could be summarized as shown in Figure 95.55. In practice, once the feeding portal branch is identified at IOUS (Figs. 95.56A and 95.57A), it is compressed using the IOUS probe on one side of the liver and the finger on the opposite side (Figs. 95.56B and 95.57B), confirming the proper compression by IOUS real-time control (Figs. 95.56C and 95.57C); in this way, it is possible to induce a transient ischemia of the portion of the liver distally to the compression site. This portion can be marked with electrocautery, the compression is released (Figs. 95.56D and 95.57D), and the resection is carried out (Figs. 95.56E and 95.57E). This technique is simple, fast, noninvasive, and reversible, with the possibility of modifying the site of compression if necessary.
Furthermore, the compression can be used in a countercompression perspective, borrowing from the technique proposed by Takayama and colleagues (1991) of defining the adjacent segmental margins. Indeed for segments such as segment I and the superior portion of segment IV, for which direct compression of the feeding portal branch is difficult, compressing the adjacent segmental branch allows the definition of their segmental margins (Torzilli et al, 2010b; Fig. 95.58). Similarly, as is described later, this technique can be applied to disclose the margin of the right anterior section of the liver by compressing the glissonian pedicles to the right posterior section and to the left hemiliver, respectively (Torzilli et al, 2009a).
More recently, for segment VIII as a whole—and selectively for its subsegmental dorsal and ventral branches—contrary to what had appeared at the first experiences with right-sided compression of the portal branches (Torzilli et al, 2010b), direct compression of their respective feeding portal branches has been demonstrated as feasible (Torzilli et al, 2011a).
Hooking of the Portal Branch
The segmental portal branches to segment IV are generally divided into two groups: those for the superior and those for the inferior portion, but the commonest branching pattern can be recognized in only half of the patients (Onishi et al, 2000). Instead of puncture under IOUS guidance, these branches can be approached by dissecting the umbilical portion. Once exposed the vessel can be encircled with a suture and pulled, under IOUS control, to verify that it is the branch to the inferior portion of segment IV. The proper portal branch can then be ligated and divided, and the discolored area that appears on the liver surface should correspond to the inferior portion of segment IV, which can be marked with electrocautery; this is a peculiar application of the so-called hooking technique (Torzilli & Makuuchi, 2001). Furthermore, the superior portion of subsegment IV could be resected by clamping the portal branch to the inferior portion, as it is identified with the hooking technique; the discolored inferior subsegment IV caudally, the plane that includes the MHV laterally, as seen on IOUS, and that marked by the falciform ligament medially delimit the area to be resected.
Other Techniques
Other methods have been proposed, such as a balloon catheter inserted transhepatically to occlude the feeding portal branch (Shimamura et al, 1986), or, more recently, the portal branch is accessed through the mesenteric vein (Ou et al, 2007). For segment VIII resection, Mazziotti and colleagues (2000) proposed the division of the liver along the main portal fissure and a subsequent intraparenchymal approach to the segment VIII glissonian pedicle. Other authors have recently suggested ablation of the feeding portal and arterial branches (Curro et al, 2009; Lupo et al, 2003; Santambrogio et al, 2008). All these alternative techniques are made less attractive by their invasive nature, and in some circumstances (Curro et al, 2009; Lupo et al, 2003; Santambrogio et al, 2008), for their irreversible damage to the target, which could imply compulsory extension of the resection area, should the first targeted vessel be incorrectly identified. This extension of the resection area, although accidental, is exactly what anatomic segmental resection seeks to avoid.
Sectionectomies (See Chapter 92)
Right Posterior Sectionectomy
As for segmentectomies, the demarcation of the sectional area to be removed is advocated. Among methods proposed for obtaining this demarcation, extrahepatic isolation of the right-sided sectional pedicles consists of careful and meticulous skeletonization of each sectional arterial and portal branch (Makuuchi et al, 1993). Alternatively, the three glissonian pedicles in their surrounding fibrous sheath could be encircled as a whole with or without the use of a hepatotomy incision (Takasaki et al, 1990). As an alternative to these established techniques, the compression technique could be applied (Torzilli et al, 2011b). The hepatic pedicle is encircled with a tourniquet but not dissected.
At IOUS, the portal pedicle for the right posterior section (segments VI and VII) is identified as well as the branches for segments VI and VII (Fig. 95.59A); the level targeted for compression is then identified (Fig. 95.59B). The surgeon’s nondominant hand is put behind the right hemiliver, and the probe is positioned with the dominant hand to show the sectional portal branch at the level of interest, which corresponds to the most distal portion of the vessel in relation to its origin but proximal to the tumor to be removed.
The surgeon next uses the fingertips of the nondominant hand and the IOUS probe as instruments to compress the liver bilaterally at the targeted position, resulting in compression of the sectional portal branch in the previously identified tract. When there is not a common sectional pedicle to segments VI and VII, compression is applied to the respective segmental portal pedicle as previously described. This maneuver is constantly monitored in real time by IOUS by means of the probe used for compression, and compression is maintained until the surface of the right posterior section lateral to the compression site starts to discolor (Fig. 95.59C); at this time the assistant marks the discolored area with the electrocautery device, and the compression is released (Fig. 95.59D). In this way, a three-dimensional plane has been drawn on the liver surface that passes through portal branch at the level of compression; liver resection is now carried out following that plane (Fig. 95.59E).
Right Anterior Sectionectomy
Similarly, as for the right posterior section, the hilar dissection and the encirclement of the sectional glissonian pedicles are the most commonly adopted techniques for defining the resection area in a fully anatomic manner. More recently, the countercompression technique has been applied for this purpose (Torzilli et al, 2009a). The portal pedicle feeding the right posterior section (segments VI and VII) is identified at IOUS, and the level targeted for compression is then detected just after its origin from the right portal branch (Fig. 95.60A). Demarcation of the right posterior section is carried out as described in the previous paragraph (Fig. 95.60, B and C). Similarly, the left portal vein (LPV) is identified at IOUS, and the level targeted for compression is then identified just past its origin from the main portal vein (Fig. 95.61A); the LPV compression is carried out and is released once the Cantlie line becomes evident by left hemiliver discoloration (Fig. 95.61, B and C), and it is demarcated with the electrocautery. Once the right anterior section is defined, resection can be carried out.
Limited Resections
Selection of an anatomic or nonanatomic resection for HCC, although a controversial issue (Cho et al, 2007; Eguchi et al, 2008; Kaibori et al, 2006; Regimbeau et al, 2002; Tanaka et al, 2008; Wakai et al, 2007), is leaning in favor of the anatomic approach (Eguchi et al, 2008; Hasegawa et al, 2005; Ueno et al, 2008). Conversely, limited resection is commonly accepted as an oncologically proper approach for CLM (Sarpel et al, 2009). For limited resections, IOUS guidance plays a fundamental role; indeed, although there is no need for identifying the area of the liver fed by the portal branch to be ligated, once the tumor is identified, the surgeon can mark the border of the lesion and that of the area to be removed on the surface of the liver with the electrocautery under IOUS control. To carry out this maneuver, the flat and thin tip of the electrocautery device is positioned between the probe and the liver surface. This results in a shadow on the IOUS image that runs deeply just below the electrocautery (Fig. 95.62, A to D). In this way, it is possible to define the position of the electrocautery device with the tumor edge and consequently to mark the nodule profile on the liver surface with electrocautery and to select the safer edge for the incision. The adequacy of the marked edge can be further checked with IOUS, as the air trapped between the probe and the irregular surface of the demarcation line drawn with the electrocautery on the liver surface can be visualized at IOUS, having a similar aspect compared with the images that result with the electrocautery (Fig. 95.63).
Additionally, with the probe positioned on the liver surface at the site of the resection margin previously drawn, the surgeon uses a fingertip to push at the opposite edge of the resection area (see Fig. 95.62A), and the profile of the resection area is visualized at IOUS. As a consequence, structures between the fingertip and the tumor edge can be precisely estimated, the resection area can be marked on the liver surface, and the dissection plane is visualized (see Fig. 95.62B). The main target to be obtained once the resection area is drawn on the liver surface is that of achieving at the end of the dissection the flattest and most regular cut surface (see Fig. 95.62E).
Liver Parenchyma Dissection
The main advantage provided by the resection guidance accomplished with the aid of IOUS is the modification of the traditional way to dissect the liver tissue, which was done on vertical planes to avoid the tumor exposure on the cut surface. IOUS allows the surgeon to follow the dissection plane in real time, to see it constantly in relation to the tumor edge, and then to modify its direction when needed: this is because it is possible to visualize the dissection plane on the IOUS image, which appears as an echogenic line as a result of the entrapment of air bubbles and clots between facing cut surfaces (Fig. 95.64). If the dissection plane is not clearly visible, it can be better visualized by inserting plain gauze (Fig. 95.65) or a specifically devised silicone gauze between facing surfaces. These techniques allow the surgeon to keep the proper dissection plane, and early recognition of an improper dissection plane permits its modification and avoids a possible tumor seeding. In this way, it is possible to carry out a rounded trajectory of the dissection plane around the tumor, avoiding its seeding and allowing the surgeon to spare important vascular structures; this results in more conservative but radical treatments and in a lower rate of major hepatectomies.
The artifacts that may show up on IOUS sometimes mask structures critical to the dissection plan, such as portal branches, which should be ligated or conversely respected. For this reason, to better visualize the targeted point where the portal branch should be divided, the so-called hooking technique has been devised (Torzilli et al, 1999b). When the glissonian sheath is exposed and skeletonized, it is encircled with a stitch. Under US control, the stitch hooking the exposed vessel is then gently pulled up, which stretches the portal branch slightly; this traction point is demonstrated clearly by IOUS (Fig. 95.66, A and B). If the exposed portal branch is not clearly visible because it has collapsed, the portal triad is unclamped. If the target site is correct, the portal branch is ligated and divided, and resection is completed under IOUS guidance; conversely, if the exposed vessel was not the targeted one, it is spared, and useless sacrifice of further liver parenchyma is avoided.
This technique is also useful in case of tumor thrombus in major portal branches (Torzilli et al, 2005b). In this situation, once the portal branch is skeletonized, it is encircled with a stitch; then the stitch is gently pulled up under IOUS control, and this traction stretches the portal branch slightly, and the traction point is demonstrated clearly by IOUS (Fig. 95.67). If the traction point is not at the level of the tumor thrombus, it is possible to ligate the portal branch and proceed with the liver resection, being sure that thrombus will not migrate because of surgical manipulation.
During liver dissection, the backflow bleeding from the hepatic veins is an important source of blood loss, and it is one of the most important factors in determining the short- and long-term outcome; therefore limiting the backflow bleeding from the hepatic veins is a priority in liver resections. A US-guided technique has been recently described for backflow bleeding control from the RHV during right-sided liver resection (Torzilli et al, 2007a). The technique is very simple and is now applied to every hepatic vein. Once the hepatocaval confluence is exposed anteriorly (Fig. 95.68, A to C), dissection proceeds until the surgeon’s fingertip is able to compress the targeted hepatic vein at its caval confluence; the effectiveness of this maneuver is checked by EF-IOUS (Fig. 95.68D to E).
Alternative Methods for Liver Dissection Guidance
In recent years, tracking systems that combine the position of the dissecting instruments and the three-dimensional (3D) liver reconstruction, based on software elaboration of the preoperative CT or MRI or the 3D US images themselves, have been proposed for dissection guidance (Chopra et al, 2008). This approach is mainly used to offer real-time and continuous guidance of the liver dissection, rather than the one warranted by IOUS guidance, which can be done repeatedly but is not providing information anyway, if dissection is not interrupted for the US check; however, up to now, data provided by a few groups are not consistent enough to disclose a real advantage of an approach that on the other hand seems rather complex. A free line between the tracking system and the trackers allocated on the US probe and the dissecting instrument used must be maintained; otherwise, data registration for navigation is interrupted. In this context, tracking systems may be optical, for which a free line of sight must be maintained between the instruments, or they may be electromagnetic, which makes them sensitive to disturbances originated by the surgical instruments.
In one of the few experiences reported, the lack of communication between elements connected via an infrared-based optical line was responsible for the failure of the navigation in 4% of those treated (Beller et al, 2007). Another problem concerning the use of these navigation systems is in the reduction of precision, once the dissection gets deeper into the liver, as a result of organ shifting and deformation that make data less reliable. This represents the major limitation of this approach, because it seems to fail to provide information exactly when more data may be needed. Indeed, with neurologic or head and neck surgery, for example, the targets are fixed, and results seem more consistent with the objectives (Hamada et al, 2005; Hohlweg-Majert et al, 2005); whereas in abdominal surgery, the mobility of the viscera do not facilitate the fixing of targets. More recently, a further modification has been introduced in the original setting in which an electromagnetic sensor is inserted into the liver under US guidance, close to the tumor to be removed (Beller et al, 2009), with the purpose of overcoming the problem of decreased navigating precision as the instrument gets deeper into the liver; however, some concerns may be raised by the further complexity of the method proposed.
Some authors are trying to overcome the problem of organ shifting and deformation during surgical maneuvers with a framework that makes feasible the updating of the 3D preoperative models with the new intraoperative data (Dagon et al, 2008). This allows us to foresee that, in the future, improvements in technology may be able to overcome the difficulties brought about by organ deformation and shifting; however, the need for adjunctive instruments in the surgical theatre—and the use and related costs of 3D US, which is somewhat more complex to interpret than two-dimensional US, even if the technical drawbacks are resolved—make unclear the advantages of the implementation of these technologies compared with the use of US guidance as it has been described here. Indeed, in expert hands, US guidance provides suitable results in terms of enhanced conservation of parenchyma in the various hepatectomies, while at the same time maintaining and somewhat improving the oncologic radicality; therefore US guidance should be considered the gold standard in terms of “navigated” liver surgery.
Postresectional Control
After nodule removal, two possibilities for specimen handling are offered by IOUS: one is the “water bath” technique, which consists of real-time control of the proper resection of the targeted nodule, verifying its complete inclusion in the specimen removed from the liver (Makuuchi, 1987; Fig. 95.69); the second is done checking the cut surface, which is refilled with saline to avoid the artifacts generated by the residual air bubbles and clots (Fig. 95.70).
In those patients in whom major resections are needed, color Doppler IOUS allows the proper positioning of the remnant liver, until the inflow and outflow are proper in terms of velocity and waveform on color Doppler US (Ogata et al, 2005; Fig. 95.71).
Conclusions
The aforementioned methods for performing an IOUS-guided resection guarantee, whenever possible, both anatomic and limited resection with a radical intent; this has consequences for both the effectiveness of the surgical treatment and for its safety. Procedures that are not guided by IOUS lead to dangerous and somewhat useless major resection or, inversely, to incomplete operations. IOUS tumor vessel classification and the related surgical policy has proven that in selected patients, it is possible to get close to the tumor burden without increasing the risk of incomplete removal and, as a consequence, of local recurrence or tumor seeding (Torzilli et al, 2005a); therefore new oncologic concepts are introduced.
For HCC, tumor exposure of the cut surface is not in contradiction with a fully anatomic resection, if it respects the oncologic requirements for this kind of tumor (see Figs. 95.32 and 95.35), and descriptions of several experiences are confirming this (Torzilli et al, 2008b; Matsui et al, 2007; Ochiai et al, 1999). Similarly, in the case of CLM, getting closer to the tumor burden under IOUS guidance, even exposing the tumor, is not only not in contradiction to the concept of radical surgery, at least in conditions otherwise not suitable to any other approach, but also does not increase the risk of local recurrence and allows survivals similar to those obtained by more conventional procedures (de Haas et al, 2008; Kokudo et al, 2002; Minagawa et al, 2000; Pawlik et al, 2005; Torzilli et al, 2009b).
In practice, this evidence suggests that with IOUS guidance, it is possible to perform conservative but radical hepatectomies, even in complex presentations, and then to enlarge the surgical indications. With this approach, the rate of major hepatectomies has been limited to about 8% in patients with tumors involving one or more hepatic veins close to their caval confluence, without performing any vascular reconstruction (Torzilli et al, 2006b); it has been limited to 4% in patients with multiple bilobar CLM, with removal of up to 49 lesions at once (Torzilli et al, 2009b).
Limitation of major hepatectomy allows resection of advanced HCC, with a less than 1% mortality rate and improved long-term outcome (Torzilli et al, 2008b). New US-guided operations have contributed to achieving these results, thus allowing technical solutions otherwise not feasible (Torzilli et al, 2008c, 2010a). Just because this approach reduces the rate of major hepatectomies, the real need could be debated for interventions such as preoperative PVE, which are adopted to prevent liver failure after removal of significant amounts of liver parenchyma (Torzilli et al, 2009c); however, this approach to liver resection, which allows the combination of widened indications and a high standard of safety, demands large incisions with extensive mobilization and complex dissection planes. These requirements run counter to the trend to use smaller incisions, such as with the anterior approach, with or without the hanging maneuver (Liu et al, 2006; Ogata et al, 2007), and in laparoscopic hepatic surgery, in which the complex IOUS-guided maneuvers described herein, and the required complex dissection planes, would be difficult to realize, at least with the same level of safety.
In spite of this, IOUS-guided liver surgery should be considered a minimally invasive procedure whose main target is the sparing of liver parenchyma, and its absolute safety is paradigmatic of what has been just affirmed. Mostly because of that, surgery can still be considered the treatment of choice for most liver tumors, in spite of the development and progresses in other local treatments, such as ablation therapies and intravascular procedures. Furthermore, this use of US intraoperatively for expanding the indications for tumor removal is paradoxically in opposition to the trend of introducing US for guiding intraoperative tumor ablation. As a matter of fact, stressing the use of IOUS for guidance, it is possible to expand indications for HCC and CLM without risking the recurrence rates observed after ablation, even when performed intraoperatively (Abdalla et al, 2004; N’Kontchou et al, 2009; Torzilli et al, 2008b, 2009b).
Abdalla EK, et al. Recurrence and outcomes following hepatic resection, radiofrequency ablation, and combined resection/ablation for colorectal liver metastases. Ann Surg. 2004;239:818-825.
Agrawal N, Fowler AL, Thomas MG. The routine use of intra-operative ultrasound in patients with colorectal cancer improves the detection of hepatic metastases. Colorectal Dis. 2006;8:192-194.
Beller S, et al. Feasibility of navigated resection of liver tumors using multiplanar visualization of intraoperative 3-dimensional ultrasound data. Ann Surg. 2007;246:288-294.
Beller S, et al. Upgrade of an optical navigation system with a permanent electromagnetic position control: a first step towards “navigated control” for liver surgery. J Hepatobiliary Pancreat Surg. 2009;16:165-170.
Bruix J, Llovet JM. Major achievements in hepatocellular carcinoma. Lancet. 2009;373:614-616.
Cerwenka H, et al. Is intraoperative ultrasonography during partial hepatectomy still necessary in the age of magnetic resonance imaging? Hepatogastroenterology. 2003;50:1539-1541.
Chen CH, et al. The accuracy of sonography in predicting steatosis and fibrosis in chronic hepatitis C. Dig Dis Sci. 2008;53:1699-1706.
Cho YB, et al. Anatomic versus non-anatomic resection for small single hepatocellular carcinomas. Hepatogastroenterology. 2007;54:1766-1769.
Chopra SS, et al. Development and validation of a three dimensional ultrasound based navigation system for tumor resection. Eur J Surg Oncol. 2008;34:456-461.
Cucchetti A, et al. Recovery from liver failure after hepatectomy for hepatocellular carcinoma in cirrhosis: meaning for the Model of End-stage Liver Disease. J Am Coll Surg. 2006;203:670-676.
Curro G, et al. Ultrasound-guided radiofrequency-assisted segmental liver resection: a new technique. Ann Surg. 2009;250:229-233.
Dagon B, Baur C, Bettschart V, 2008: A framework for intraoperative update of 3D deformable models in liver surgery: proceedings of the 30th Annual International IEEE EMBS Conference, Vancouver, British Columbia, Canada, August 20-24.
de Haas RJ, et al. R1 resection by necessity for colorectal liver metastases: is it still a contraindication to surgery? Ann Surg. 2008;248:626-637.
Eguchi S, et al. Comparison of the outcomes between an anatomical subsegmentectomy and a non-anatomical minor hepatectomy for single hepatocellular carcinomas based on a Japanese nationwide survey. Surgery. 2008;143:469-475.
Garcea G, Ong SL, Maddern GJ. Inoperable colorectal liver metastases: a declining entity? Eur J Cancer. 2008;44:2555-2572.
Gotoh N, et al. A novel image-guided surgery of hepatocellular carcinoma by indocyanine green fluorescence imaging navigation. J Surg Oncol. 2009;100:75-79.
Hamada H, et al. Efficacy of a navigation system in neuro-endoscopic surgery. Minim Invasive Neurosurg. 2005;48:197-201.
Hasegawa K, et al. Prognostic impact of anatomic resection for hepatocellular carcinoma. Ann Surg. 2005;242:252-259.
Hemming AW, et al. Preoperative portal vein embolization for extended hepatectomy. Ann Surg. 2003;237:686-693.
Hohlweg-Majert B, et al. Navigational maxillofacial surgery using virtual models. World J Surg. 2005;29:1530-1538.
Imamura H, et al. One thousand fifty-six consecutive hepatectomies without mortality in 8 years. Arch Surg. 2003;138:198-206.
International Consensus Group for Hepatocellular Carcinoma. Pathologic diagnosis of early hepatocellular carcinoma: a report of the International Consensus Group for Hepatocellular Neoplasia. Hepatology. 2009;49:658-664.
Ishizawa T, et al. Real-time identification of liver cancers by using indocyanine green fluorescent imaging. Cancer. 2009;115:2491-2504.
Jaeck D, et al. A two-stage hepatectomy procedure combined with portal vein embolization to achieve curative resection for initially unresectable multiple and bilobar colorectal liver metastases. Ann Surg. 2004;240:1037-1049.
Jarnagin WR, et al. What is the yield of intraoperative ultrasonography during partial hepatectomy for malignant disease? J Am Coll Surg. 2001;192:577-583.
Kaibori M, et al. Comparison of limited and anatomic hepatic resection for hepatocellular carcinoma with hepatitis C. Surgery. 2006;139:385-394.
Kane RA, et al. The impact of intraoperative ultrasonography on surgery for liver neoplasms. J Ultrasound Med. 1994;13:1-6.
Kokudo N, et al. Management of new hepatic nodules detected by intraoperative ultrasonography during hepatic resection for hepatocellular carcinoma. Surgery. 1996;119:634-640.
Kokudo N, et al. Genetic and histological assessment of surgical margins in resected liver metastases from colorectal carcinoma: minimum surgical margins for successful resection. Arch Surg. 2002;137:833-840.
Kudo M. New sonographic techniques for the diagnosis and treatment of hepatocellular carcinoma. Hepatol Res. 2007;37(Suppl 2):S193-S199.
Liu CL, et al. Abdominal drainage after hepatic resection is contraindicated in patients with chronic liver diseases. Ann Surg. 2004;239:194-201.
Liu CL, et al. Anterior approach versus conventional approach right hepatic resection for large hepatocellular carcinoma: a prospective randomized controlled study. Ann Surg. 2006;244:194-203.
Lupo L, et al. Anatomical hepatic resection using radiofrequency thermoablation in the treatment of primary or secondary liver tumors. Tumori. 2003;89(4 Suppl):105-106.
Machi J, et al. Accuracy of intraoperative ultrasonography in diagnosing liver metastasis from colorectal cancer: evaluation with postoperative follow-up results. World J Surg. 1991;15:551-556.
Makuuchi M. Abdominal Intraoperative Utrasonography. Tokyo: lgaku-Shoin; 1987.
Makuuchi M, et al. Ultrasonically guided liver surgery. Jpn J Ultrasonics Med. 1980;7:45-49.
Makuuchi M, et al. Four new hepatectomy procedures for resection of the right hepatic vein and preservation of the inferior right hepatic vein. Surg Gynecol Obstet. 1987;164:68-72.
Makuuchi M, et al. Personal experience of right anterior segmentectomy (segments V and VIII) for hepatic malignancies. Surgery. 1993;114:52-58.
Matsui Y, et al. Postoperative outcomes in patients with hepatocellular carcinomas resected with exposure of the tumor surface: clinical role of the no-margin resection. Arch Surg. 2007;142:596-602.
Mazziotti A, et al. Isolated resection of segment 8 for liver tumors: a new approach for anatomical segmentectomy. Arch Surg. 2000;135:1224-1249.
Minagawa M, et al. Extension of the frontiers of surgical indications in the treatment of liver metastases from colorectal cancer: long-term results of our experience. Ann Surg. 2000;231:487-499.
Nakano H, et al. Contrast-enhanced intraoperative ultrasonography equipped with late Kupffer-phase image obtained by Sonazoid in patients with colorectal liver metastases. World J Gastroenterol. 2008;14:3207-3211.
N’Kontchou G, et al. Radiofrequency ablation of hepatocellular carcinoma: long-term results and prognostic factors in 235 Western patients with cirrhosis. Hepatology. 2009;50:1475-1483.
Ochiai T, et al. Hepatic resection with and without surgical margins for hepatocellular carcinoma in patients with impaired liver function. Hepatogastroenterology. 1999;46:1885-1889.
Ogata S, Kianmanesh R, Belghiti J. Doppler assessment after right hepatectomy confirms the need to fix the remnant left liver in the anatomical position. Br J Surg. 2005;92:592-595.
Ogata S, et al. Two hundred liver hanging maneuvers for major hepatectomy: a single-center experience. Ann Surg. 2007;245:31-35.
Onishi H, et al. Surgical anatomy of the medial segment (S4) of the liver with special reference to bile ducts and vessels. Hepatogastroenterology. 2000;47:143-150.
Ou JR, Chen W, Lau WY. A new technique of hepatic segmentectomy by selective portal venous occlusion using a balloon catheter through a branch of the superior mesenteric vein. World J Surg. 2007;31:1240-1242.
Pang YY. The Brisbane 2000 terminology of liver anatomy and resections. HPB. 2002;2:333-339. HPB (Oxford) 4:99
Pawlik TM, et al. Effect of surgical margin status on survival and site of recurrence after hepatic resection for colorectal metastases. Ann Surg. 2005;241:715-722.
Quaia E, et al. Characterization of focal liver lesions with contrast-specific US modes and a sulfur hexafluoride-filled microbubble contrast agent: diagnostic performance and confidence. Radiology. 2004;232:420-430.
Regimbeau JM, et al. Extent of liver resection influences the outcome in patients with cirrhosis and small hepatocellular carcinoma. Surgery. 2002;131:311-317.
Roncalli M, et al. The vascular profile of regenerative and dysplastic nodules of the cirrhotic liver: implications for diagnosis and classification. Hepatology. 1999;30:1174-1178.
Sahani DV, et al. Intraoperative US in patients undergoing surgery for liver neoplasms: comparison with MR imaging. Radiology. 2004;232:810-814.
Santambrogio R, et al. Laparoscopic radiofrequency of hepatocellular carcinoma using ultrasound-guided selective intrahepatic vascular occlusion. Surg Endosc. 2008;22:2051-2055.
Sarpel U, et al. Does anatomic versus nonanatomic resection affect recurrence and survival in patients undergoing surgery for colorectal liver metastasis? Ann Surg Oncol. 2009;16:379-384.
Schroeder RA, et al. Predictive indices of morbidity and mortality after liver resection. Ann Surg. 2006;243:373-379.
Shimamura Y, et al. Selective portal branch occlusion by balloon catheter during liver resection. Surgery. 1986;100:938-941.
Siperstein AE, et al. Survival after radiofrequency ablation of colorectal liver metastases: 10-years experience. Ann Surg. 2007;246:559-565.
Sotiropoulos GC, et al. Resectability of hepatocellular carcinoma: evaluation of 333 consecutive cases at a single hepatobiliary specialty center and systematic review of the literature. Hepatogastroenterology. 2006;53:322-329.
Takada T, et al. Contrast-enhanced intraoperative ultrasonography of small hepatocellular carcinoma. Surgery. 1990;107:528-532.
Takasaki K, et al. Highly anatomically systematized hepatic resection with Glissonean sheath code transection at the hepatic hilus. Int Surg. 1990;75:73-77.
Takayama T, et al. A new method for mapping hepatic subsegment: counterstaining identification technique. Surgery. 1991;109:226-229.
Takigawa Y, et al. New lesions detected by intraoperative ultrasound during liver resection for hepatocellular carcinoma. Ultrasound Med Biol. 2001;27:151-156.
Tanaka K, et al. Anatomic versus limited nonanatomic resection for solitary hepatocellular carcinoma. Surgery. 2008;143:607-615.
Torzilli G, Makuuchi M. Ultrasound-guided liver subsegmentectomy: the peculiarity of segment 4. J Am Coll Surg. 2001;193:706-708.
Torzilli G, Makuuchi M. Ultrasound-guided finger compression in liver subsegmentectomy for hepatocellular carcinoma. Surg Endosc. 2004;18:136-139.
Torzilli G, et al. No-mortality liver resection for hepatocellular carcinoma in cirrhotic and non-cirrhotic patients: is there a way? A prospective analysis of our approach. Arch Surg. 1999;134:984-992.
Torzilli G, et al. A new technical aspect of ultrasound-guided liver surgery. Am J Surg. 1999;178:341-343.
Torzilli G, et al. Contrast-enhanced ultrasonography during liver surgery. Br J Surg. 2004;91:1165-1167.
Torzilli G, et al. “Radical but conservative” is the main goal for ultrasonography-guided liver resection: prospective validation of this approach. J Am Coll Surg. 2005;201:517-528.
Torzilli G, et al. Utility of the hooking technique for cases of major hepatectomy. Surg Endosc. 2005;19:1156-1157.
Torzilli G, et al. Right inferior phrenic vein indicating the right hepatic vein confluence into the inferior vena cava. Am J Surg. 2006;192:690-694.
Torzilli G, et al. Ultrasonographically guided surgical approach to liver tumors involving the hepatic veins close to the caval confluence. Br J Surg. 2006;93:1238-1246.
Torzilli G, et al. Back-flow bleeding control during resection of right-sided liver tumors by means of ultrasound-guided finger compression of the right hepatic vein at its caval confluence. Hepatogastroenterol. 2007;54:1364-1367.
Torzilli G, et al. Contrast-enhanced intraoperative ultrasonography during surgery for hepatocellular carcinoma in liver cirrhosis: is it useful or useless? A prospective cohort study of our experience. Ann Surg Oncol. 2007;14:1347-1355.
Torzilli G, et al. Does contrast-enhanced intraoperative ultrasonography impact radicality of hepatectomies for colorectal cancer liver metastases in spite of modern preoperative imaging? Analysis on a prospective cohort. Eur J Cancer. 2008;6:16-23.
Torzilli G, et al. Hepatectomy for hepatocellular carcinoma in stage B and C of Barcelona Clinic Liver Cancer Classification: results of a prospective analysis. Arch Surg. 2008;143:1082-1090.
Torzilli G, et al. Systematic extended right posterior sectionectomy: a safe and effective alternative to right hepatectomy. Ann Surg. 2008;247:603-611.
Torzilli G, et al. New technique for defining the right anterior section intraoperatively using ultrasound-guided finger counter-compression. J Am Coll Surg. 2009;209:e8-e11.
Torzilli G, et al. One-stage ultrasonographically guided hepatectomy for multiple bilobar colorectal metastases: a feasible and effective alternative to the 2-stage approach. Surgery. 2009;146:60-71.
Torzilli G, et al. Ultrasound guided liver resection: does this approach limit the need for portal vein embolization? Hepatogastroenterology. 2009;56:1483-1490.
Torzilli G, et al. A new systematic small for size resection for liver tumors invading the middle hepatic vein at its caval confluence: the mini-mesohepatectomy. Ann Surg. 2010;251:33-39.
Torzilli G, et al. Anatomical segmental and subsegmental resection of the liver for hepatocellular carcinoma: a new approach by means of ultrasound-guided vessel compression. Ann Surg. 2010;251:229-235.
Torzilli G, et al. Intraoperative ultrasonographic detection of communicating veins between adjacent hepatic veins during hepatectomy for tumours at the hepatocaval confluence. Br J Surg. 2010;97:1867-1873.
Torzilli G, et al. Total or partial anatomical resection of segment 8 using the ultrasound-guided finger compression technique. HPB (Oxford). 2011;13(8):586-591.
Torzilli G, et al. Anatomical right posterior sectionectomy: a further expansion of the ultrasound-guided compression technique. Updates Surg. 2011;63(2):91-95.
Ueno S, et al. Efficacy of anatomic resection vs non-anatomic resection for small nodular hepatocellular carcinoma based on gross classification. J Hepatobiliary Pancreat Surg. 2008;15:493-500.
Vauthey JN, et al. Is extended hepatectomy for hepatobiliary malignancy justified? Ann Surg. 2004;239:722-739.
Wakai T, et al. Anatomic resection independently improves long-term survival in patients with T1-T2 hepatocellular carcinoma. Ann Surg Oncol. 2007;14:1356-1365.
Wicherts DA, et al. Long-term results of two-stage hepatectomy for irresectable colorectal cancer liver metastases. Ann Surg. 2008;248:994-1005.
Yoshidome H, et al. Interval period tumor progression: does delayed hepatectomy detect occult metastases in synchronous colorectal metastases? J Gastrointest Surg. 2008;12:1391-1398.