CHAPTER 81 Acute Treatment of Patients with Spinal Cord Injury
Since the implementation of emergency medical systems and model spinal cord injury care systems in the early 1970s, the mortality rate from spinal cord injury during the initial period of hospitalization has decreased fivefold.1 This reduction primarily reflects improvements in the initial management of these injuries, which still account for about 10,000 to 12,000 new cases each year.2–4 The improved care at time of injury and thereafter has increased life expectancies. Consequently, the current prevalence of about 250,000 people with spinal cord injury in the United States will continue to grow.5,6 The cost to society, in both health care expenses and living expenses, is almost $8 billion per year. Lifetime expenses per patient range from $435,000 to more than $2.6 million.6,7 That the average age of spinal cord–injured patients is about 30 years further increases lifetime expenses.3,8
Since 2005 the most frequent cause of spinal cord injury in the United States has been motor vehicle crashes (42%), followed by falls (27.1%). Recently, injuries from falls have increased the most. The proportion of injuries related to violence (15.3%) has decreased from its peak in 1999 (24.8%), and the proportion of sports-related injuries has also diminished (7.4%).4 About 50% of victims have a complete neurologic deficit below the level of injury and require significant assistance with activities of daily living.8 Prevention is the single best remedy for spinal cord injuries. Nonetheless, advances in the acute management of these injuries have had the greatest effect on improving long-term outcomes.1
Prehospital Management
Cervical Spine Immobilization
Evidence from the past 30 years has shown that patients should be placed in rigid external cervical spine immobilization when extricated at the scene.1,9,10 The rationale for immobilization is predicated on the theory that neurologic function can be compromised by pathologic motion of injured vertebrae. Although logical, no class I evidence supports this supposition, and controversy surrounds the effectiveness of prophylactic spinal immobilization.11,12
Several estimates indicate that 3% to 26% of spinal cord injuries occur after the initial injury, presumably caused by abnormal movement of the spinal column and subsequent damage to neural structures.9,13–15 If so, a large number of cervical spinal cord injuries might be prevented by earlier immobilization of the cervical spine. Supporting this assertion is the dramatic decrease in the percentage of complete injuries (as opposed to incomplete) in the past 30 years.16,17 Prehospital immobilization has not been the only major change during this period, but it is thought to have been a major contributor to this decrease.
The large number of traumatically injured patients in the United States each year raises the issue of which patients need spinal immobilization. The routine use of immobilization to prevent unnecessary injuries makes sense. However, only a small percentage of immobilized patients actually have a significant spinal injury. The decision to immobilize a patient is usually based on the mechanism of injury and the patient’s clinical status. The following clinical criteria for a potential spinal injury are most predictive of the need for immobilization18: an altered mental status; focal neurologic deficits; evidence of intoxication, spinal pain, or tenderness; and suspected extremity fracture. Even when these criteria are strictly applied, up to 5% of spinal injuries may still be missed.19
Although techniques of spinal immobilization may vary, guidelines from the American College of Surgeons recommend that a hard backboard, rigid cervical collar, and lateral support device be used, with tape or straps to secure the patient, collar, and lateral support devices to the backboard.20 The general goal for adequate immobilization is the “neutral position,” which is the normal anatomic position of the head and torso when standing and looking forward.21 Although these recommendations are straightforward, there is some controversy about the appropriate position to attain optimal spinal stability. The neutral position is usually attained by providing about 12 degrees of cervical spine extension on a lateral radiograph. However, this position may not offer the best alignment. Elevating the occiput 2 cm to create a slight flexion provides more room for the spinal cord at C5-6, which is the spinal level most commonly injured.22 However, the large variability in body habitus precludes rigid guidelines for the use of occipital padding.
Spinal immobilization is not a completely benign process, and its limitations must be recognized. A large portion of patients experience moderate to occasionally severe pain after relatively short periods (30 minutes) of immobilization on a rigid backboard.23 Relieving this discomfort with a small amount of padding has no effect on the level of immobilization and may minimize patient movements related to pain.24 Another concern with immobilization is the increase in intracranial pressure (ICP) related to the use of rigid cervical collars.25,26 Although such increases are relatively small (4.5 mm Hg and 24.7 cm H2O), they may be clinically significant in patients with severe head injuries whose ICP is already elevated. Furthermore, immobilization on a rigid, nonpadded backboard has been associated with the development of pressure sores. Not surprisingly, this relationship is related to the length of time spent on the backboard: the presence of ulcers increases after 2 or more hours on the board.27
Transportation of Spinal Cord–Injured Patients
Many advances in the transportation of spinal cord–injured patients reflect protocols implemented after the Vietnam War. The most significant advance was rapid transportation to a specialized center for definitive care. In the United States and Canada this system has grown into the trauma level system at a regional basis. Several studies have shown significant benefits associated with both rapid transport times to definitive medical centers and improved neurologic function at admission.28–30 Although patients fare better when transported quickly to definitive centers, unstable patients may still be better served by transport to a regional center until stabilized and then later transported to the appropriate tertiary center.20 Because there are no prospective, randomized trials (and unlikely to be any) on this issue, the treatment of each case must be individualized.
The type of transportation available usually depends on the region, but most areas in the United States are served by both air and ground transportation. In rural areas, air transportation providing rapid arrival at tertiary care centers can dramatically decrease mortality rates in trauma patients.31 In a retrospective analysis at a single institution, there was no difference in the neurologic outcomes of patients transported by ground or air.32 Thus both air and ground transportation appear to be equally safe, and the decision to use one or the other depends on availability and speed.
Various transfer techniques from the scene of the accident or between vehicles and different support equipment (e.g., backboards, gurneys, or beds for magnetic resonance imaging [MRI] or computed tomography [CT]) are available. Some examples include the log roll, the Haines maneuver, and the multihand (fireman’s) lift.20,33,34 All are equally effective for moving patients from the scene of an accident to a backboard. The choice should depend on the individual circumstances.
Clinical Assessment
Even when the suggestion of a spinal cord injury is high, at the initial clinical assessment the examiner must consider the basics involved with evaluation of traumatically injured patients. The initial evaluation consists of the primary and secondary surveys from the Advanced Trauma Life Support (ATLS) guidelines.20 The primary survey is usually initiated at the accident scene and is focused on the basics of life support: airway, breathing, and circulation. Once these issues have been addressed, the secondary survey is used to evaluate patients for significant injuries from head to toe.
An adequate airway and ventilation are the most important factors in the initial survey of traumatically injured patients. An injured spinal cord is susceptible to further injury from hypoxemia. Even if patients are alert and breathing adequately, supplemental oxygen is recommended if a spinal cord injury is suspected. In obtunded patients with a suspected spinal cord injury, early placement of an endotracheal or nasotracheal tube is critical to limit secondary injury from hypoxemia. A dedicated airway can be placed safely in patients with a cervical spine injury, although neck extension should be minimized during its placement.35
Treatment of hypotension is part of the resuscitation phase of the ATLS protocol, which parallels the primary and secondary surveys. Immediate aggressive treatment of hypotension is important to maintain adequate perfusion to the injured spinal cord to reduce further secondary injury from tissue ischemia.36 However, patients in neurogenic shock should not be overloaded with fluid. This problem is most common in elderly patients who have less cardiovascular capacity for high intravascular volumes than younger individuals. Once patients reach a dedicated trauma center, pressors and intravascular fluid monitors such as a Swan-Ganz catheter can be used to control blood pressure within a narrow range.
Neurologic Assessment
After the initial evaluation of traumatically injured patients, it is crucial to determine the presence of a spinal cord or column injury. A rapid examination in the secondary survey usually unmasks pronounced neurologic deficits, but a more detailed neurologic examination may be necessary to uncover subtle deficits. Once patients’ respiratory and cardiovascular systems are stabilized, a full neurologic examination can be performed. The examination must incorporate established classification scales for spinal cord injury. Outside specific clinical trials, the most widely used spinal cord injury scales are the Frankel and American Spinal Injury Association (ASIA) scales (Table 81–1). In fact, recent guidelines recommend use of the ASIA scale, although strictly as an option.37
A detailed neurologic assessment not only helps classify an injury but also guides further radiographic assessment and treatment. The neurologic examination will determine whether an injury is complete or incomplete. With the improvements in prehospital emergency systems during the past 30 years, the ratio of incomplete-to-complete injuries has increased considerably.16,17 An incomplete injury is a predictor of a better outcome than a complete injury. Therefore urgent treatment is assumed to be necessary, although no randomized trials have verified this assumption.38
Spinal Cord Injury Syndromes
Several types of incomplete spinal cord injuries are based on the functional anatomy of the spinal cord in relation to the mechanism of injury. A common type of incomplete spinal cord injury is the central cord syndrome, which is identified by more motor and sensory deficits involving the upper extremities than the lower extremities.39 Initially, a burning dysesthesia of the upper extremities often accompanies the other deficits and gradually resolves within a few days. This injury is unique to the cervical spinal cord with the pattern of injury related to edema of the central gray matter at the cervical level. This edematous reaction is caused by crushing or stretching the spinal cord in patients with significant stenosis, either congenital or degenerative. Hyperextension combined with stenosis is the most common mechanism underlying this injury. A similar injury occurs in children while their interlaminar and intervertebral ligaments are still lax enough to allow extreme hyperextension. An acute traumatic cervical disc herniation can also cause the same type of injury.
Central cord syndrome causes multilevel dysfunction in motor and sensory neurons at the cervical level while relatively sparing the axons communicating with lower extremity neurons. This pattern is further compounded by the medial location of axons innervating the cervical neurons compared with the lateral location of lumbar neurons. The central portion of the spinal cord may be susceptible because the area is a vascular watershed.40 In the most severe cases, the edema leads to severe ischemia, capillary destruction, and hematomyelia. The most useful imaging study for these patients is a T2-weighted MRI sequence, which can visualize edema and hemorrhage, if present (Fig. 81–1). Acute decompression is recommended for patients with an acutely herniated disc or traumatic fracture.41 In patients with cervical stenosis or spondylosis, we recommend waiting until the initial edema recedes or until the neurologic impairment has plateaued. Depending on the pathology, anterior or posterior decompression can then be performed.
A rare type of spinal cord injury is the Brown-Séquard syndrome, or hemicord syndrome. It is usually caused by penetrating injuries but may be associated with lateral bony fractures or acutely herniated disc fragments. The hallmark of the syndrome is ipsilateral motor deficits and dorsal column dysfunction and contralateral spinothalamic dysfunction.42 It is rarely seen in isolation, which sometimes makes it difficult to diagnose definitively.
Radiographic Assessment
One of the earliest dilemmas in evaluating traumatically injured patients is how far the radiographic analysis should proceed to rule out spinal injury. In the current medicolegal environment, this decision can be daunting, given the cost of some of the studies involved. Patients with no neurologic symptoms or signs, no spinal pain or tenderness, no distracting injuries, and no impairment by drugs or ethanol should require no radiographic assessment.43
The most compelling support for this guideline is from the National Emergency X-radiography Utilization Study Group (NEXUS) in 2000.44 The study called for three-view radiography, CT, and MRI as needed in each patient to rule out spinal injuries. Of 34,069 patients enrolled, 4309 met the asymptomatic criteria. Only two patients were found to have a clinically significant spinal column injury, and none was found to have significant neurologic abnormalities. On the basis of the negative predictive value of 99.9% and the positive predictive value of 1.9%, the current standard is to clear asymptomatic patients of cervical spine injuries on the basis of the clinical examination alone if the previously mentioned criteria are met. This guideline, however, is valid only for cervical spine clearance and must be extrapolated carefully to patients with potential thoracic and lumbar injuries. The presence of thoracolumbar fractures, however, is always associated with pain in awake patients because significant force is necessary to produce fractures at these levels.
In all other symptomatic patients, an initial radiographic analysis should be performed with lateral, anteroposterior, and open-mouth views of the cervical spine. Thoracic and lumbar two-view radiographs should be obtained in patients with pain in these regions or who have a significant mechanism of injury. In patients with blunt trauma, these screening studies are critical because the rate of significant cervical, thoracic, or lumbar spine injury ranges between 2% and 6%.45,46 When properly performed, simple screening cervical radiography has a negative predictive value between 93% and 98%.47–49 The most common cause of a missed cervical spine injury is the failure to visualize the area of injury adequately. Therefore the spine must be imaged from the occiput to the first thoracic vertebrae (Fig. 81–2).
Over the past 20 years, the use of CT to evaluate for spinal injuries after trauma has increased significantly. Currently, CT is the modality most often used to assess areas not adequately visualized with radiography. This combination leads to an almost 100% sensitivity in detecting significant spinal injuries in symptomatic patients.50 The increased cost of CT compared with radiography is the main issue involved with its use. CT, however, is cost effective when used as an adjunct to radiography.51 We routinely obtain cervical CT scans in symptomatic trauma patients, and almost all comatose patients undergo CT of the cervical, thoracic, and lumbar spine on admission.
Rapid advances in MRI technology and the recent increased availability of scanners have led to the use of this modality for the evaluation of spinal injuries in trauma patients. Although the ability of MRI to image bony structures is limited, it is sensitive to abnormalities in soft tissue, especially ligamentous structures, intervertebral discs, and the spinal cord itself. A study by the Eastern Association for the Surgery of Trauma found a high rate of detection of cervical abnormalities in trauma patients using MRI.52 Because MRI is extremely sensitive to any soft tissue injury, the rate of false-positive scans for significant spinal injury in trauma patients is thought to be elevated.53 However, MRI can help determine the absence of spinal injury because the rate of false-negative findings is low when used acutely.54 Consequently, we believe that MRI should be used in the presence of a neurologic deficit, if time permits, or to rule out any type of spinal injury when other studies are inconclusive or unavailable.
Trauma patients must also be screened for the presence of instability. In the thoracic and lumbar spine, the presence of instability is usually related to significant bony trauma. Instability in the cervical spine can be subtle such as a hairline fracture of a facet or ligamentous injury that may be undetected on radiography or even CT. Symptomatic or obtunded patients should always be evaluated for cervical instability. The best test is an adequate series of flexion-extension lateral radiographs. Two recent studies analyzed the efficacy of flexion-extension views in trauma patients.55,56 Both showed a low rate of false-negative findings (99% and 100% negative predictive value, respectively), but both were limited by inadequate studies in up to 30% of the examinations. When the incidence of inadequate studies is considered, the sensitivity of this modality remains in question. If dynamic radiographs are inadequate or inconclusive, MRI can be used to rule out instability but not necessarily to diagnose it because of the high rate of false-positive findings.54 For comatose patients, the options to determine instability are MRI or passive dynamic radiography with fluoroscopy. Passive dynamic radiographs can be a viable first option, as shown by the relatively high number of obtunded patients with unstable cervical spines discovered by this method.57
Clinical Management
The Edwin Smith papyrus, the world’s oldest surgical document, states that an injury of the spinal cord that renders the patient “unconscious of his two legs and his two arms” is “an ailment not to be treated.”58 Although outcomes from spinal cord injuries are still usually poor, our understanding of these injuries and potential treatments has advanced considerably in the 5000 years since this document was written.
Hypoxemia in spinal cord injury can be caused by many factors. The two most common causes are decreased respiratory drive due to unconsciousness and decreased mechanics of ventilation due to a cervical spinal cord injury. In each case, early intubation and aggressive oxygenation should be implemented. Some authors recommend oxygenation to a PaO2 of more than 100 mm Hg, at least for an initial period.10 Patients with a complete injury above C5 should seriously be considered for prophylactic intubation because of the high rate of ventilatory decompensation in these patients.59 In patients with adequate initial ventilation and an injury at a lower level of the spinal cord, noninvasive measures can be used to ensure adequate oxygenation. These measures include monitoring oxygen saturation and periodic PaO2 levels in an intensive care setting. The authors also recommend other measures such as early nasogastric decompression, head-of-bed elevation if possible, and early start of a bowel regimen to assist with respiratory effort by decreasing the work of breathing. If ventilatory decompensation begins, prophylactic intubation should be undertaken to prevent hypoxemia and its additive effect on the secondary injury cascade.60
Hypotension can be related to the spinal cord injury itself or to hypovolemia from another traumatic injury. When sympathetic outflow is disrupted in the cervical or upper thoracic spinal cord, peripheral vascular resistance decreases profoundly, leading to hypotension. This is the primary mechanism underlying neurogenic shock and can usually be differentiated from hypovolemic shock by the relative lack of a tachycardic response. These patients must be treated aggressively to avoid prolonged hypotension and accentuation of the secondary injury cascade. The effect of hypotension is compounded by the dysfunctional hemodynamic autoregulation found in the spinal cord parenchyma immediately after a traumatic injury.61 Animal studies have shown that maintaining adequate systemic blood pressure maintains spinal cord perfusion pressure in the physiologic range and limits the amount of ischemic damage to the site of injury.62
To address the hemodynamic alterations that follow spinal cord injury, several prospective studies have evaluated the role of hemodynamic stabilization and its effect on long-term outcomes after spinal cord injury.30,36,63 One study used invasive hemodynamic monitoring with Swan-Ganz catheters and aggressively treated hypotension with fluids, volume expanders, and vasopressors to maintain mean arterial pressure above 85 mm Hg for the first 7 days after injury.36 This aggressive management led to better-than-expected neurologic outcomes including 30% of patients with a complete cervical spinal cord injury regaining the ability to walk. However, none of these studies included control groups, owing to the obvious ethical problems. Based on the extensive experimental evidence and prospective clinical data, systemic blood pressure should be maintained above a mean of 85 to 90 mm Hg immediately after injury and for at least 1 week thereafter.
Neurologic Management
Secondary injury includes many factors that ultimately lead to tissue destruction over the ensuing hours, days, and weeks. These factors include vascular compromise that reduces blood flow to the spinal cord, loss of vascular autoregulation, capillary destruction, loss of microcirculation, vasospasm, thrombosis, and hemorrhage.64 The ensuing cellular hypoperfusion shifts the balance of electrolytes and changes the permeability of cell membranes. Excitatory amino acid neurotransmitters accumulate, arachidonic acid is released, free radicals and prostaglandins are produced, and lipid peroxidation occurs.65 The result is cellular and axonal death from these mechanisms, as well as from apoptotic events (Fig. 81–3). Microglia infiltration and scarring, potent inhibitors of axonal regrowth, follow. Over the past 2 decades, major research efforts have addressed the attenuation of this secondary injury cascade and several treatment paradigms have arisen from this research.66
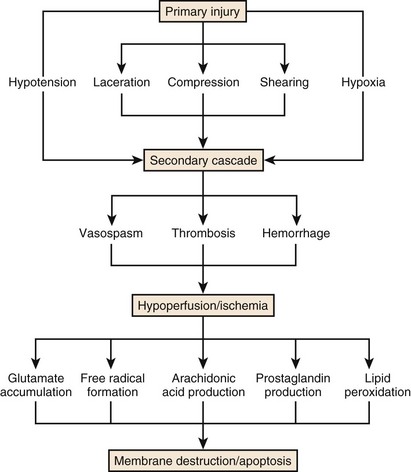
FIGURE 81–3 Schematic demonstrating the primary and secondary injury cascades in spinal cord injury.
(Used with permission from Barrow Neurological Institute.)
Acute Management of Dislocations
A common type of cervical dislocation occurs due to extreme flexion during axial loading leading to subluxation of the facet joints. Dislocations can be unilateral or bilateral and can lead to incomplete or complete neurologic deficits. More than a fifth of patients will have no neurologic deficits.67 The subluxation is often accompanied by a fracture of the facet complex or other part of the vertebrae. In the absence of any other compressive or more rostral fracture, the primary treatment for these injuries is to restore spinal alignment by closed reduction.
Closed reduction can be accomplished with rapid, manual reduction or gradually by increasing the weight applied during distraction. The two types of skull fixation are the halo ring with four to six points of fixation or Gardner-Wells tongs with two fixation points. Rapid, manual reduction is best performed with the halo ring to help assist flexion during distraction. It can be performed rapidly in the emergency department under sedation or in the operating room under general anesthesia, depending on the clinical scenario. Gradual reduction can be performed with either fixator and usually involves adding 3 to 5 pounds of weight per rostral vertebral level to the pulley system. Weight is added gradually, and a lateral cervical radiograph is usually obtained to verify the alignment of the spine after each increase (Fig. 81–4). This method should only be used when frequent neurologic examinations are possible such as in an intensive care unit. The amount of weight that can safely be used may reach as high as 80% of the patient’s total weight before reduction is achieved.67 If patients complain of pain, their neurologic status worsens, or overdistraction is seen on fluoroscopy, no more weight should be applied. Most patients (73% to 97%) can be realigned successfully using these techniques, which is why they are recommended as first-line treatments.67,68 When closed reduction is unsuccessful, open reduction should be accomplished as rapidly as possible in patients with a significant neurologic deficit. The subluxed levels should be fixated internally and fused after reduction to compensate for significant ligamentous damage. The choice of anterior or posterior fixation depends on the need for a discectomy and on the ability to reduce the patient before fixation. In severe subluxations, however, combined anterior and posterior fixation is commonly applied (Fig. 81–5).
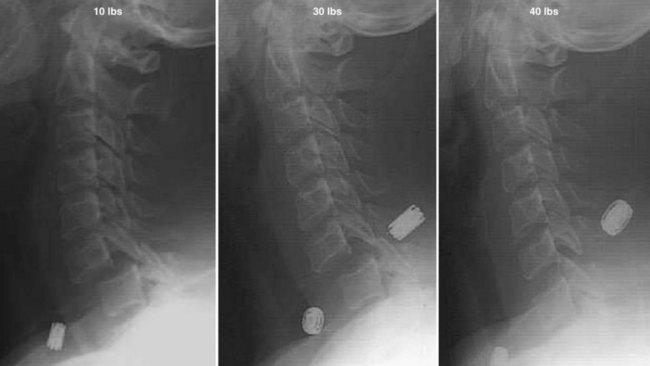
FIGURE 81–4 Lateral radiographic sequence demonstrating the gradual reduction of a cervical spine subluxation at C6-C7.
Recently, the safety of closed reduction has been questioned after several reports of deterioration after reduction.67,69,70 The most worrisome complication from closed reduction is a herniated disc fragment and the potential for further spinal cord compression.71 Indeed, when a prereduction MRI is available, herniated discs are detected in about half the patients (Fig. 81–6).72,73 When, however, reduction has been performed in awake patients, the presence of a herniated disc has not correlated with neurologic deterioration. According to the recently published guidelines, the use of prereduction MRI is strictly an option.74,75 The overall risk of permanent neurologic worsening after closed reduction is less than 1% and thus appears to be relatively safe.
Acute Surgical Management
The indications for surgical management of spinal injuries are decompression of compressive lesions and stabilization of unstable spinal segments. In the acute setting, there is little need for surgical stabilization alone because external orthotics or immobilization is sufficient to prevent further injury in the short term. In the case of compressive lesions, however, surgical decompression during the acute period may be necessary. Early surgical management of spinal cord injury is controversial because it has never been proven to improve long-term neurologic outcomes.1,76 Such data, however, are based solely on retrospective, nonrandomized studies with significant biases in terms of the surgical indications. However, a randomized, controlled trial to evaluate the efficacy of early surgery on spinal cord injury may never be viable in today’s medical environment.
Animal studies have provided the strongest data supporting the effectiveness of early decompression in spinal cord injury.77,78 Based on these studies, the critical window for decompression is within at least the first 24 hours and more likely much sooner. An inherent problem with evaluating the effectiveness of early surgery is the time it takes to perform the surgery after injury. Given the time needed for patient transport, clinical and radiographic evaluation, and mobilization of an operative theater, this critical period may have already elapsed. In most trauma systems a reasonable time from injury to decompressive surgery is at least 8 hours and more likely to be 12 to 24 hours. This delay may increase in cases of lumbar or thoracic anterior spinal injuries when a general or thoracic surgeon is necessary to help assist the approach to the spinal column. Unfortunately, most studies evaluating surgery for spinal cord injury define “early” as anywhere from 24 to 72 hours after injury.76,79,80 Given this variability, most evidence still points to a need for decompression as soon as possible, preferably within 12 hours to 24 hours.
Several studies have demonstrated improvements in neurologic outcomes after surgery, but they were plagued with the same poor study design as the negative studies. One of the more compelling human studies was performed by Papadopoulos and coworkers,79 who demonstrated a significant benefit from immediate surgery compared with nonoperative treatment. Their patients also had shorter stays in the hospital and intensive care units. However, the selection process was biased: Patients believed to have no chance of improvement were less likely to undergo surgery. Although admission Frankel grades were similar in the two groups, the inherent bias of this study must be considered in the interpretation of its results. One-year results from the Surgical Treatment of Acute Spinal Cord Injury Study (STASCIS) have been presented in abstract form. This study obtained favorable results for patients undergoing decompressive surgery within 24 hours compared with a delayed-treatment group.81
A critical factor in the decision to operate emergently in patients with a spinal cord injury is the severity of injury. Most clinicians consider an incomplete injury to be a more urgent priority for decompression than a complete injury (Fig. 81–7). The rationale for early decompression of incomplete injuries is that continued compression will lead to further tissue injury and loss of neurologic function in an already compromised spinal cord. Indeed, the neurologic outcome of patients with a spinal cord injury correlates highly with the severity of their neurologic deficit at admission, regardless of their treatment.1 This notion is further supported by the effectiveness of decompressive surgery in a select group of patients with incomplete spinal cord injuries from a combination of minor trauma and preexisting spondylosis.82 Some clinicians, however, still worry that surgical manipulation during early treatment of an incomplete injury may further damage a compromised cord and create a bias toward early surgery in patients with complete injuries.76 On the basis of available evidence, we recommend urgent, decompressive surgery in patients with a neurologic deficit related to spinal cord compression. Doing so, however, is strictly an option, and the individual clinical scenario must be considered for each patient.
Neuroprotective Agents
A recent explosion in basic research has focused on attenuating the secondary injury cascade in spinal cord injury. The cascade is a promising target for acute treatment of spinal cord injury because it develops hours to weeks after injury. In various experimental animal models of spinal cord injury, neuroprotective agents that limit excitotoxicity and membrane breakdown have been studied extensively. Significant neurologic improvement has followed treatment with sodium channel modulators, glutamate-receptor blockers, glucocorticoids, and gangliosides.83–86 Only a few treatments, however, have been tested in human trials of acute spinal cord injury.
A controversial treatment for spinal cord injury is the use of high-dose steroids. Glucocorticoids such as methylprednisolone stabilize cellular membranes, reduce vasogenic edema, enhance spinal cord blood flow, alter the concentration of electrolytes at the site of injury, inhibit endorphin release, scavenge damaging free radicals, and limit the inflammatory response after injury.87 Based on these basic properties of methylprednisolone and on the promising results from animal trials, the first randomized trial in humans was reported as the National Acute Spinal Cord Injury Study (NASCIS) in 1984.88 One year after injury, however, this study showed no differences in the neurologic outcomes of patients receiving low and high doses of methylprednisolone. Subsequent animal studies, however, showed that the dose used in the trial was too low to produce significant differences in long-term functional outcomes.89
To address the issue of underdosing with methylprednisolone in the first trial, a second trial (NASCIS 2) was undertaken with a high dose of methylprednisolone to assess neurologic improvement after acute spinal cord injury.90 This trial demonstrated a small but significant improvement in motor scores 1 year after injury compared with a placebo group. However, several aspects of the study have been criticized strongly. The primary complaints were the lack of a standardized assessment of functional outcome (as opposed to basic motor scores) and the use of post hoc analysis to determine statistical significance.91 A third trial (NASCIS 3) was then undertaken and showed that methylprednisolone had a greater benefit if given within 3 hours rather than within 8 hours.92
Methylprednisolone has also been associated with medical complications, primarily an increased incidence of infections, gastrointestinal problems, pulmonary issues, and myopathy.93,94 The evidence concerning its long-term effects is mixed.95 Several groups have reexamined the results of the NASCIS trials and reached different conclusions about the efficacy of methylprednisolone.96–99 According to current published guidelines, methylprednisolone for the treatment of acute spinal cord injury is only considered an option with the caveat that “the evidence suggesting harmful side effects is more consistent than any suggestion of ‘clinical benefit.’ ”74,75 Many physicians continue to use high-dose methylprednisolone, and surveys have shown that the major motivating factor behind its use is fear of litigation.100
Other agents tested in human clinical trials include tirilazad, naloxone, and GM1 ganglioside. The opiate antagonist naloxone was included in the second methylprednisolone trial but showed no significant clinical benefit.90 In the third trial, the 21-aminosteroid tirilazad was compared with methylprednisolone. No benefit was demonstrated, but the trial lacked a true placebo group.92 Two randomized clinical trials have analyzed the effectiveness of the ganglioside GM1 on neurologic improvement after spinal cord injury. The first, smaller study showed a marked improvement in functional neurologic outcome in the GM1 group compared with controls.101 This improvement failed to materialize in the larger study. This treatment is only considered an option for acute spinal cord injury and is not used in routine clinical practice.102,103
Several other potential treatment modalities have been shown to be effective in animal studies or safe in human phase I trials. Agents focused on neuroprotection such as BA-210 (Cethrin) (inhibits glial scar formation), minocycline (multifactorial effects), and activated macrophage injection show early promise and are undergoing further evaluation.103–105 Studied treatments to induce regeneration and repair include stem cell therapy, as well as oscillating electrical field stimulation.106,107 Moreover, the first use of human embryonic stem cells to treat acute spinal cord injury has been approved by the U.S. Food and Drug Administration, and the Geron corporation began recruitment in 2009.108
Summary
Pearls
Pitfalls
Key Points
1 Guidelines for the Management of Acute Cervical Spine and Spinal Cord Injuries. Neurosurgery. 2002;50(Suppl):S1-S199.
2 Hoffman JR, Mower WR, Wolfson AB, et al. Validity of a set of clinical criteria to rule out injury to the cervical spine in patients with blunt trauma. National Emergency X-Radiography Utilization Study Group. N Engl J Med. 2000;343:94-99.
3 Papadopoulos SM, Selden NR, Quint DJ, et al. Immediate spinal cord decompression for cervical spinal cord injury: Feasibility and outcome. J Trauma. 2002;52:323-332.
4 Toscano J. Prevention of neurological deterioration before admission to a spinal cord injury unit. Paraplegia. 1988;26:143-150.
5 Vale FL, Burns J, Jackson AB, Hadley MN. Combined medical and surgical treatment after acute spinal cord injury: Results of a prospective pilot study to assess the merits of aggressive medical resuscitation and blood pressure management. J Neurosurg. 1997;87:239-246.
1 Waters RL, Meyer PRJr., Adkins RH, et al. Emergency, acute, and surgical management of spine trauma. Arch Phys Med Rehabil. 1999;80:1383-1390.
2 Marshall LF. Epidemiology and cost of central nervous system injury. Clin Neurosurg. 2000;46:105-112.
3 Burke DA, Linden RD, Zhang YP, et al. Incidence rates and populations at risk for spinal cord injury: A regional study. Spinal Cord. 2001;39:274-278.
4 Spinal Cord Injury Information Network. www.spinalcord.uab.edu, 2008. Ref Type: Electronic Citation
5 Frankel HL, Coll JR, Charlifue SW, et al. Long-term survival in spinal cord injury: a fifty year investigation. Spinal Cord. 1998;36:266-274.
6 Spinal cord injury facts and figures at a glance. August 2004. J Spinal Cord Med. 2004;27(Suppl 1):S139-S140.
7 DeVivo MJ. Causes and costs of spinal cord injury in the United States. Spinal Cord. 1997;35:809-813.
8 Nobunaga AI, Go BK, Karunas RB. Recent demographic and injury trends in people served by the Model Spinal Cord Injury Care Systems. Arch Phys Med Rehabil. 1999;80:1372-1382.
9 Toscano J. Prevention of neurological deterioration before admission to a spinal cord injury unit. Paraplegia. 1988;26:143-150.
10 Dyson-Hudson TA, Stein AB. Acute management of traumatic cervical spinal cord injuries. Mt Sinai J Med. 1999;66:170-178.
11 Hauswald M, Ong G, Tandberg D, et al. Out-of-hospital spinal immobilization: its effect on neurologic injury. Acad Emerg Med. 1998;5:214-219.
12 Cervical spine immobilization before admission to the hospital. Neurosurgery. 2002;50:S7-17.
13 Brunette DD, Rockswold GL. Neurologic recovery following rapid spinal realignment for complete cervical spinal cord injury. J Trauma. 1987;27:445-447.
14 Prasad VS, Schwartz A, Bhutani R, et al. Characteristics of injuries to the cervical spine and spinal cord in polytrauma patient population: experience from a regional trauma unit. Spinal Cord. 1999;37:560-568.
15 Podolsky S, Baraff LJ, Simon RR, et al. Efficacy of cervical spine immobilization methods. J Trauma. 1983;23:461-465.
16 Garfin SR, Shackford SR, Marshall LF, et al. Care of the multiply injured patient with cervical spine injury. Clin Orthop. 1989:19-29.
17 Green BA, Eismont FJ, O’Heir JT. Spinal cord injury—a systems approach: prevention, emergency medical services, and emergency room management. Crit Care Clin. 1987;3:471-493.
18 Domeier RM, Evans RW, Swor RA, et al. The reliability of prehospital clinical evaluation for potential spinal injury is not affected by the mechanism of injury. Prehosp Emerg Care. 1999;3:332-337.
19 Domeier RM, Swor RA, Evans RW, et al. Multicenter prospective validation of prehospital clinical spinal clearance criteria. J Trauma. 2002;53:744-750.
20 American College of Surgeons CoT. Advanced Trauma Life Support Program for Doctors: ATLS Instructor Course Manual. Chicago: American College of Surgeons; 1997:pp 263-300.
21 Schriger DL. Immobilizing the cervical spine in trauma: should we seek an optimal position or an adequate one? Ann Emerg Med. 1996;28:351-353.
22 De Lorenzo RA, Olson JE, Boska M, et al. Optimal positioning for cervical immobilization. Ann Emerg Med. 1996;28:301-308.
23 Chan D, Goldberg R, Tascone A, et al. The effect of spinal immobilization on healthy volunteers. Ann Emerg Med. 1994;23:48-51.
24 Barney R, Cordell W. Pain associated with immobilization on rigid spine boards (abstract). Ann Emerg Med. 1989;18:918.
25 Kolb JC, Summers RL, Galli RL. Cervical collar-induced changes in intracranial pressure. Am J Emerg Med. 1999;17:135-137.
26 Davies G, Deakin C, Wilson A. The effect of a rigid collar on intracranial pressure. Injury. 1996;27:647-649.
27 Linares HA, Mawson AR, Suarez E, et al. Association between pressure sores and immobilization in the immediate post-injury period. Orthopedics. 1987;10:571-573.
28 Hachen HJ. Emergency transportation in the event of acute spinal cord lesion. Paraplegia. 1974;12:33-37.
29 Tator CH, Duncan EG, Edmonds VE, et al. Changes in epidemiology of acute spinal cord injury from 1947 to 1981. Surg Neurol. 1993;40:207-215.
30 Tator CH, Rowed DW, Schwartz ML, et al. Management of acute spinal cord injuries. Can J Surg. 1984;27:289-293. 296
31 Boyd CR, Corse KM, Campbell RC. Emergency interhospital transport of the major trauma patient: air versus ground. J Trauma. 1989;29:789-793.
32 Burney RE, Waggoner R, Maynard FM. Stabilization of spinal injury for early transfer. J Trauma. 1989;29:1497-1499.
33 Gunn BD, Eizenberg N, Silberstein M, et al. How should an unconscious person with a suspected neck injury be positioned? Prehospital Disaster Med. 1995;10:239-244.
34 Dick T, Land R. Spinal immobilization devices: Part I-Cervical extrication collars. J Emerg Med Serv JEMS. 1982;7:26-32.
35 Rhee KJ, Green W, Holcroft JW, et al. Oral intubation in the multiply injured patient: the risk of exacerbating spinal cord damage. Ann Emerg Med. 1990;19:511-514.
36 Vale FL, Burns J, Jackson AB, et al. Combined medical and surgical treatment after acute spinal cord injury: results of a prospective pilot study to assess the merits of aggressive medical resuscitation and blood pressure management. J Neurosurg. 1997;87:239-246.
37 Clinical assessment after acute cervical spinal cord injury. Neurosurgery. 2002;50:S21-S29.
38 Marino RJ, Ditunno JFJr., Donovan WH, et al. Neurologic recovery after traumatic spinal cord injury: data from the Model Spinal Cord Injury Systems. Arch Phys Med Rehabil. 1999;80:1391-1396.
39 Schneider RC, Cherry G, Pantek H. The syndrome of acute central cervical spinal cord injury; with special reference to the mechanisms involved in hyperextension injuries of cervical spine. J Neurosurg. 1954;11:546-577.
40 Turnbull IM. Chapter 5. Blood supply of the spinal cord: normal and pathological considerations. Clin Neurosurg. 1973;20:56-84.
41 Guest J, Eleraky MA, Apostolides PJ, et al. Traumatic central cord syndrome: results of surgical management. J Neurosurg Spine. 2002;97:25-32.
42 Brown-Séquard C. Lectures on the physiology and pathology of the nervous system and on the treatment of organic nervous affections: Lecture II, Part 1. Lancet. 1869;93:i1-i3.
43 Radiographic assessment of the cervical spine in symptomatic trauma patients. Neurosurgery. 2002;50:S36-S43.
44 Hoffman JR, Mower WR, Wolfson AB, et al. Validity of a set of clinical criteria to rule out injury to the cervical spine in patients with blunt trauma. National Emergency X-Radiography Utilization Study Group. N Engl J Med. 2000;343:94-99.
45 Goldberg W, Mueller C, Panacek E, et al. Distribution and patterns of blunt traumatic cervical spine injury. Ann Emerg Med. 2001;38:17-21.
46 Holmes JF, Miller PQ, Panacek EA, et al. Epidemiology of thoracolumbar spine injury in blunt trauma. Acad Emerg Med. 2001;8:866-872.
47 Ajani AE, Cooper DJ, Scheinkestel CD, et al. Optimal assessment of cervical spine trauma in critically ill patients: a prospective evaluation. Anaesth Intensive Care. 1998;26:487-491.
48 Berne JD, Velmahos GC, El Tawil Q, et al. Value of complete cervical helical computed tomographic scanning in identifying cervical spine injury in the unevaluable blunt trauma patient with multiple injuries: a prospective study. J Trauma. 1999;47:896-902.
49 MacDonald RL, Schwartz ML, Mirich D, et al. Diagnosis of cervical spine injury in motor vehicle crash victims: how many X-rays are enough? J Trauma. 1990;30:392-397.
50 Mace SE. Emergency evaluation of cervical spine injuries: CT versus plain radiographs. Ann Emerg Med. 1985;14:973-975.
51 Tan E, Schweitzer ME, Vaccaro L, et al. Is computed tomography of nonvisualized C7-T1 cost-effective? J Spinal Disord. 1999;12:472-476.
52 Ghanta MK, Smith LM, Polin RS, et al. An analysis of Eastern Association for the Surgery of Trauma practice guidelines for cervical spine evaluation in a series of patients with multiple imaging techniques. Am Surg. 2002;68:563-567.
53 Benzel EC, Hart BL, Ball PA, et al. Magnetic resonance imaging for the evaluation of patients with occult cervical spine injury. J Neurosurg. 1996;85:824-829.
54 Horn EM, Lekovic GP, Feiz-Erfan I, et al. Cervical magnetic resonance imaging abnormalities not predictive of cervical spine instability in traumatically injured patients. Invited submission from the Joint Section Meeting on Disorders of the Spine and Peripheral Nerves, March 2004. J Neurosurg Spine. 2004;1:39-42.
55 Lewis LM, Docherty M, Ruoff BE, et al. Flexion-extension views in the evaluation of cervical-spine injuries. Ann Emerg Med. 1991;20:117-121.
56 Insko EK, Gracias VH, Gupta R, et al. Utility of flexion and extension radiographs of the cervical spine in the acute evaluation of blunt trauma. J Trauma. 2002;53:426-429.
57 Cox MW, McCarthy M, Lemmon G, et al. Cervical spine instability: clearance using dynamic fluoroscopy. Curr Surg. 2001;58:96-100.
58 Breasted J. The Edwin Smith surgical papyrus. In: Wilkins R, editor. Neurosurgical Classics. New York: Johnson Reprint Corp.; 1965:1-5.
59 Velmahos GC, Toutouzas K, Chan L, et al. Intubation after cervical spinal cord injury: to be done selectively or routinely? Am Surg. 2003;69:891-894.
60 Soderstrom CA, Brumback RJ. Early care of the patient with cervical spine injury. Orthop Clin North Am. 1986;17:3-13.
61 Senter HJ, Venes JL. Loss of autoregulation and posttraumatic ischemia following experimental spinal cord trauma. J Neurosurg. 1979;50:198-206.
62 Tator CH, Fehlings MG. Review of the secondary injury theory of acute spinal cord trauma with emphasis on vascular mechanisms. J Neurosurg. 1991;75:15-26.
63 Levi L, Wolf A, Belzberg H. Hemodynamic parameters in patients with acute cervical cord trauma: description, intervention, and prediction of outcome. Neurosurgery. 1993;33:1007-1016.
64 Profyris C, Cheema SS, Zang D, et al. Degenerative and regenerative mechanisms governing spinal cord injury. Neurobiol Dis. 2004;15:415-436.
65 Kwon BK, Tetzlaff W, Grauer JN, et al. Pathophysiology and pharmacologic treatment of acute spinal cord injury. Spine J. 2004;4:451-464.
66 Lammertse DP. Update on pharmaceutical trials in acute spinal cord injury. J Spinal Cord Med. 2004;27:319-325.
67 Grant GA, Mirza SK, Chapman JR, et al. Risk of early closed reduction in cervical spine subluxation injuries. J Neurosurg Spine. 1999;90:13-18.
68 Lee AS, MacLean JC, Newton DA. Rapid traction for reduction of cervical spine dislocations. J Bone Joint Surg Br. 1994;76:352-356.
69 Robertson PA, Ryan MD. Neurological deterioration after reduction of cervical subluxation. Mechanical compression by disc tissue. J Bone Joint Surg Br. 1992;74:224-227.
70 Eismont FJ, Arena MJ, Green BA. Extrusion of an intervertebral disc associated with traumatic subluxation or dislocation of cervical facets. Case report. J Bone Joint Surg Am. 1991;73:1555-1560.
71 Doran SE, Papadopoulos SM, Ducker TB, et al. Magnetic resonance imaging documentation of coexistent traumatic locked facets of the cervical spine and disc herniation. J Neurosurg. 1993;79:341-345.
72 Vaccaro AR, Falatyn SP, Flanders AE, et al. Magnetic resonance evaluation of the intervertebral disc, spinal ligaments, and spinal cord before and after closed traction reduction of cervical spine dislocations. Spine. 1999;24:1210-1217.
73 Rizzolo SJ, Piazza MR, Cotler JM, et al. Intervertebral disc injury complicating cervical spine trauma. Spine. 1991;16:S187-S189.
74 Initial closed reduction of cervical spine fracture-dislocation injuries. Neurosurgery. 2002;50:S44-S50.
75 Pharmacological therapy after acute cervical spinal cord injury. Neurosurgery. 2002;50:S63-S72.
76 McKinley W, Meade MA, Kirshblum S, et al. Outcomes of early surgical management versus late or no surgical intervention after acute spinal cord injury. Arch Phys Med Rehabil. 2004;85:1818-1825.
77 Fehlings MG, Sekhon LH, Tator C. The role and timing of decompression in acute spinal cord injury: what do we know? What should we do? Spine. 2001;26:S101-S110.
78 Dimar JR, Glassman SD, Raque GH, et al. The influence of spinal canal narrowing and timing of decompression on neurologic recovery after spinal cord contusion in a rat model. Spine. 1999;24:1623-1633.
79 Papadopoulos SM, Selden NR, Quint DJ, et al. Immediate spinal cord decompression for cervical spinal cord injury: feasibility and outcome. J Trauma. 2002;52:323-332.
80 Mirza SK, Krengel WFIII, Chapman JR, et al. Early versus delayed surgery for acute cervical spinal cord injury. Clin Orthop. 1999:104-114.
81 Fehlings M, Arabi B, Dvorak M, et al: One year outcomes of the STASCIS study: A prospective, multicenter trial to evaluate the role and timing of decompression in patients with cervical spinal cord injury. 76th Annual Meeting of the American Association of Neurological Surgeons 2008.
82 Chen TY, Dickman CA, Eleraky M, et al. The role of decompression for acute incomplete cervical spinal cord injury in cervical spondylosis. Spine. 1998;23:2398-2403.
83 Gaviria M, Privat A, d’Arbigny P, et al. Neuroprotective effects of a novel NMDA antagonist, Gacyclidine, after experimental contusive spinal cord injury in adult rats. Brain Res. 2000;874:200-209.
84 Hains BC, Saab CY, Lo AC, et al. Sodium channel blockade with phenytoin protects spinal cord axons, enhances axonal conduction, and improves functional motor recovery after contusion SCI. Exp Neurol. 2004;188:365-377.
85 Behrmann DL, Bresnahan JC, Beattie MS. Modeling of acute spinal cord injury in the rat: neuroprotection and enhanced recovery with methylprednisolone, U-74006F and YM-14673. Exp Neurol. 1994;126:61-75.
86 Mao J, Hayes RL, Price DD, et al. Post-injury treatment with GM1 ganglioside reduces nociceptive behaviors and spinal cord metabolic activity in rats with experimental peripheral mononeuropathy. Brain Res. 1992;584:18-27.
87 Hall ED, Braughler JM. Glucocorticoid mechanisms in acute spinal cord injury: a review and therapeutic rationale. Surg Neurol. 1982;18:320-327.
88 Bracken MB, Collins WF, Freeman DF, et al. Efficacy of methylprednisolone in acute spinal cord injury. JAMA. 1984;251:45-52.
89 Braughler JM, Hall ED, Means ED, et al. Evaluation of an intensive methylprednisolone sodium succinate dosing regimen in experimental spinal cord injury. J Neurosurg. 1987;67:102-105.
90 Bracken MB, Shepard MJ, Collins WF, et al. A randomized, controlled trial of methylprednisolone or naloxone in the treatment of acute spinal-cord injury. Results of the Second National Acute Spinal Cord Injury Study. N Engl J Med. 1990;322:1405-1411.
91 Hurlbert RJ. Methylprednisolone for acute spinal cord injury: an inappropriate standard of care. J Neurosurg Spine. 2000;93:1-7.
92 Bracken MB, Shepard MJ, Holford TR, et al. Administration of methylprednisolone for 24 or 48 hours or tirilazad mesylate for 48 hours in the treatment of acute spinal cord injury. Results of the Third National Acute Spinal Cord Injury Randomized Controlled Trial. National Acute Spinal Cord Injury Study. JAMA. 1997;277:1597-1604.
93 Matsumoto T, Tamaki T, Kawakami M, et al. Early complications of high-dose methylprednisolone sodium succinate treatment in the follow-up of acute cervical spinal cord injury. Spine. 2001;26:426-430.
94 Qian T, Guo X, Levi AD, et al. High-dose methylprednisolone may cause myopathy in acute spinal cord injury patients. Spinal Cord. 2005;43:199-203.
95 Gerndt SJ, Rodriguez JL, Pawlik JW, et al. Consequences of high-dose steroid therapy for acute spinal cord injury. J Trauma. 1997;42:279-284.
96 Nesathuri S. Steroids and spinal cord injury: Revisiting the NASCIS 2 and NASCIS 3 trials. J Trauma Injury Infection Critical Care. 1998;45:1088.
97 Coleman WP, Benzel D, Cahill DW, et al. A critical appraisal of the reporting of the National Acute Spinal Cord Injury Studies (II and III) of methylprednisolone in acute spinal cord injury. J Spinal Disord. 2000;13:185-199.
98 Hurlbert RJ. Methylprednisolone for acute spinal cord injury: an inappropriate standard of care. J Neurosurg. 2000;93:1-7.
99 Short DJ, El Masry WS, Jones PW. High dose methylprednisolone in the management of acute spinal cord injury—a systematic review from a clinical perspective. Spinal Cord. 2000;38:273-286.
100 Hurlbert RJ, Moulton R. Why do you prescribe methylprednisolone for acute spinal cord injury? A Canadian perspective and a position statement. Can J Neurol Sci. 2002;29:236-239.
101 Geisler FH, Dorsey FC, Coleman WP. Recovery of motor function after spinal-cord injury–a randomized, placebo-controlled trial with GM-1 ganglioside. N Engl J Med. 1991;324:1829-1838.
102 Geisler FH, Coleman WP, Grieco G, et al. The Sygen multicenter acute spinal cord injury study. Spine. 2001;26:S87-S98.
103 Hurlbert RJ. Strategies of medical intervention in the management of acute spinal cord injury. Spine. 2006;31:S16-S21.
104 Teng Y, Choi H, Onario R, et al. Minocycline inhibits contusion-triggered mitochondrial cytochrome c release and mitigates functional deficits after spinal cord injury. Proc Natl Acad Sci USA. 2004;101:3071-3076.
105 Knoller N, Auerbach G, Fulga V, et al. Clinical experience using incubated autologous macrophages as a treatment for complete spinal cord injury: phase I study results. J Neurosurg Spine. 2005;3:173-181.
106 Iwanami A, Kaneko S, Nakamura M, et al. Transplantation of human neural stem cells for spinal cord injury in primates. J Neurosci Res. 2005;80:182-190.
107 Shapiro S, Borgens R, Pascuzzi R, et al. Oscillating field stimulation for complete spinal cord injury in humans: a phase 1 trial. J Neurosurg Spine. 2005;2:3-10.
108 Wadman M. Stem cells ready for prime time. Nature. 2009;457:516.