Imaging
Radiology and Catheter-Based Angiocardiography
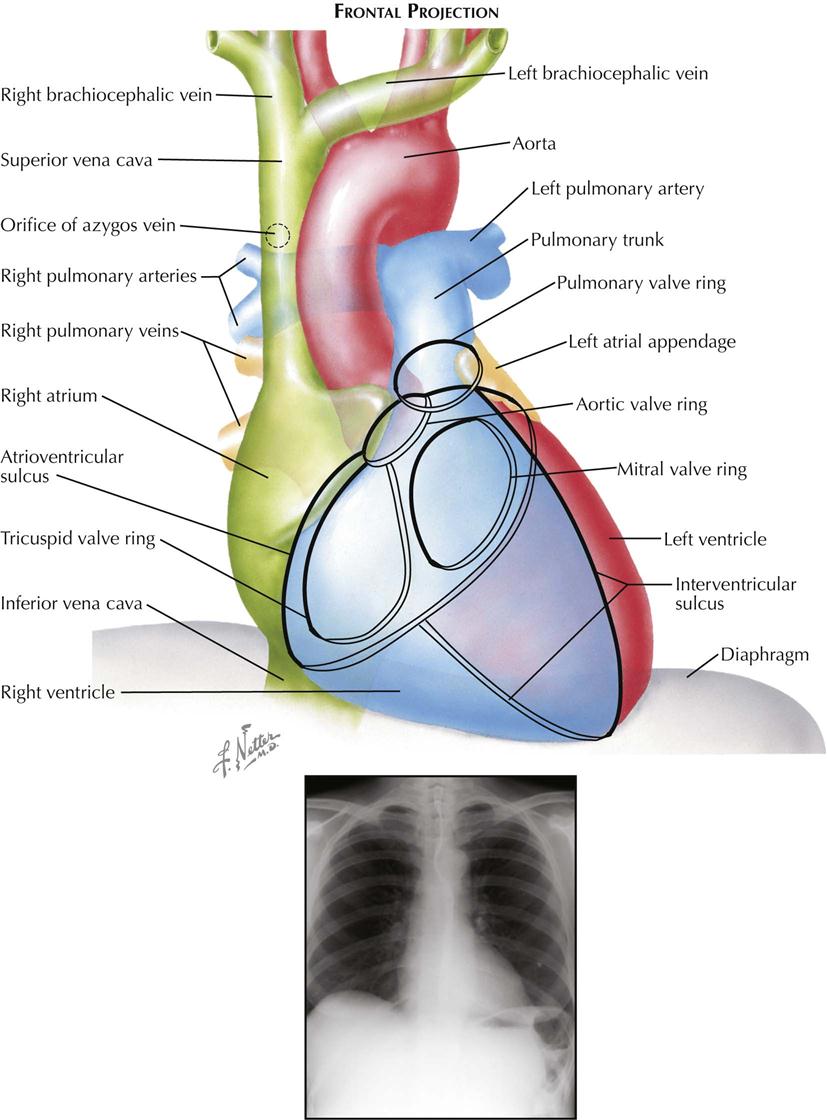
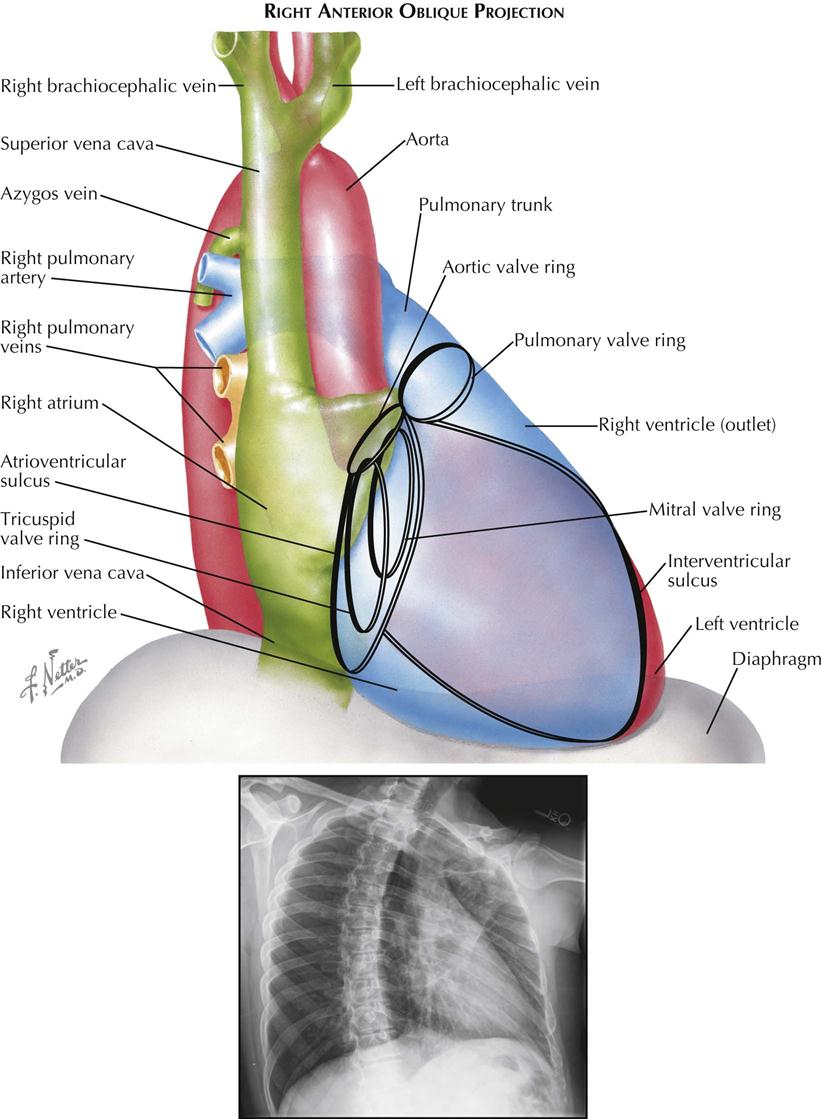
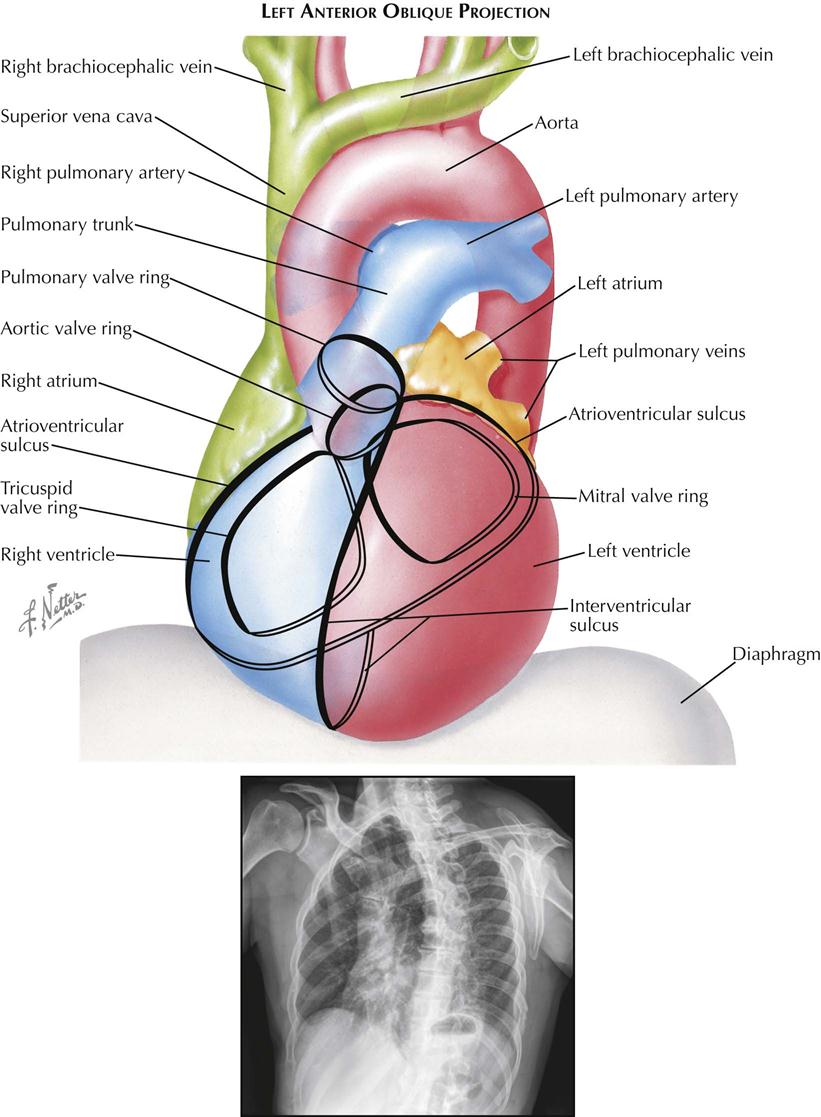
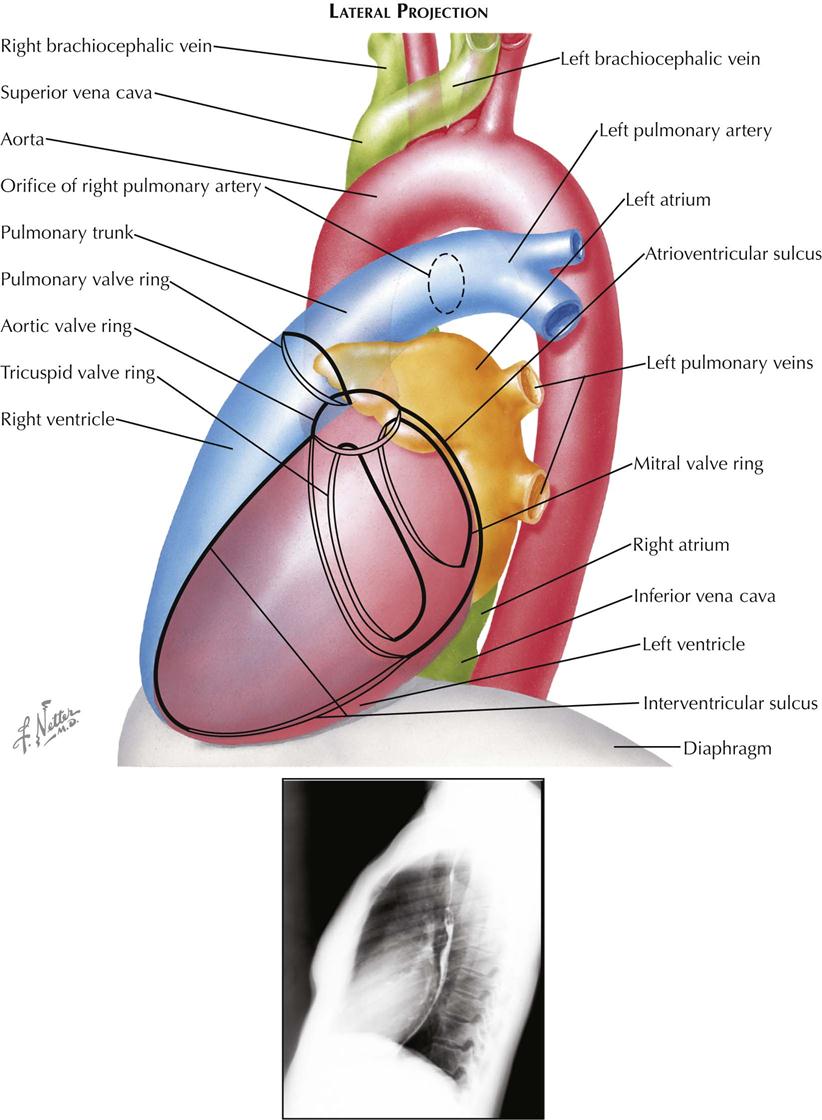
Radiology
Radiologic examination is an essential part of the evaluation of cardiac disease. The size of the heart and identification of chamber enlargement and pericardial, cardiac, and coronary calcification, as well as information on heart function and hemodynamics, can be determined from chest radiography, fluoroscopic examination, and angiocardiographic observations.
The myocardium, valves, and other heart structures have similar radiodensity and therefore cannot be distinguished radiologically unless calcified. Similarly, the walls of the cardiac chambers cannot be visually separated from the blood within unless the opacity of the blood is increased by the injection of a contrast material. The outer borders of the heart can be seen because the relatively homogeneous cardiac silhouette is contrasted against the lucent, air-containing lungs.
The shadow of the heart seen on standard radiographs or by fluoroscopy is magnified and somewhat distorted. At the target-to-film or screen distances customarily used, the x-ray beam diverges as it passes through the patient. The structures farther from the film are more magnified than those closer. The distortion can be largely eliminated if the x-ray beam is composed of parallel rather than divergent rays. For most purposes, heart size can be adequately estimated from the standard 6-foot chest film by comparing the apparent cardiac size with that of the thorax. Allowances must be made for the degree of inspiration—the higher the diaphragm, the larger the apparent size of the heart—and the age of the patient. An infant’s heart is relatively large compared with the chest, whereas an older patient’s chest is often small in relation to the heart.
Since the heart is a three-dimensional (3D) structure and only two borders are seen in any one view, radiographs need to be secured in several projections to bring the various chambers and great vessels into profile. Plain films of the chest also allow an evaluation of the pulmonary vasculature and the lung changes that may be associated with heart disease. Increased size and tortuosity of the pulmonary arteries and veins usually indicate a left-to-right shunt, whereas decreased prominence of these vessels is associated with a right-to-left shunt. When the pulmonary artery flow is greatly decreased, as in severe tetralogy of Fallot, the vascular pattern in the lungs often is reticular and nondirectional rather than an orderly radiation of vessels from the lung hilum. This indicates the presence of a significant collateral flow through the bronchial arteries.
In congestive failure or obstruction on the left side of the heart, as in mitral stenosis, the pulmonary veins become engorged. If this progresses to pulmonary hypertension, the veins, together with the peripheral pulmonary arteries, become quite small while the central pulmonary arteries dilate and become bulbous.
Frontal Projection
Most chest films are made in the frontal projection, so this is the view of the heart usually seen, often providing the first suggestion of cardiac disease. Frontal is the most easily reproducible projection, and thus heart size generally is evaluated in this view (see Plate 3-1). Enlargement of the left atrium and left ventricle, as well as the right atrium, generally can be recognized in the frontal projection. The right ventricle, although it forms no borders, can produce characteristic changes in the cardiac contour.
The upper half of the right contour of the cardiac silhouette is formed by the superior vena cava (SVC) and the lower half by the lateral wall of the right atrium. The margin of the SVC is straight, but that of the right atrium bulges outward. The angle between these two contours represents the superior aspect of the right atrium. If the patient takes a deep inspiration, an indentation on the right border of the heart can be seen just above the diaphragm, identifying the junction of the inferior vena cava (IVC) and right atrium.
On the left side, the uppermost part of the cardiovascular silhouette is formed by the distal arch of the aorta as it curves posteriorly and inferiorly to become the descending thoracic aorta. This is seen as a localized bulge extending from the left side of the mediastinum above the right tracheobronchial angle. This bulge usually produces a localized indentation on the left side of the esophagus. In the presence of a right aortic arch, the bulge will be on the right side and will displace the esophagus to the left. Immediately below the aortic bulge, the main pulmonary trunk and left main pulmonary artery are border forming. A small segment of the left cardiac silhouette below the pulmonary artery is formed by the left atrial appendage. This segment normally is flat or slightly convex and is continuous with the curve of the left ventricle, which forms the largest part of the left border of the cardiac contour. The point of transition between the normal left atrial appendage and the left ventricle usually cannot be identified on radiographs.
The apex of the heart is formed by the left ventricle. In the frontal projection the right ventricle is completely hidden within the cardiac silhouette. Occasionally on deep inspiration, a part of the diaphragmatic surface of the heart near the cardiac apex is disclosed. An indentation in this region marks the interventricular sulcus between the two ventricles.
Enlargement of the right atrium causes outward bowing and increased curvature of the border of the right side of the heart. When the right ventricle increases in size, the heart enlarges to the left, the apex is usually lifted, and the groove of the interventricular sulcus appears higher on the apex of the heart than normal. As it enlarges, the right ventricle elongates as well as widens, resulting in elevation of the main pulmonary artery. As the left ventricle enlarges, the cardiac apex is displaced downward and to the left. Often the entire left cardiac border is displaced to the left, becoming increasingly convex.
Left atrial enlargement is detected in the frontal view primarily by dilatation of the left atrial appendage, which produces a localized bulge of the left contour below the pulmonary artery segment. In addition, the enlarged left atrium often increases the density of the central part of the cardiac silhouette and, when sufficiently large, may elevate the left main bronchus. With increasing dilatation, the right border of the left atrium may be seen within the cardiac silhouette to the right of the spine, producing a second curved contour medial to the right atrial margin. With further enlargement, the left atrium may project behind and beyond the right atrium so that the left atrium will form the right border of the cardiac shadow. The border of the right atrium will then be seen within the shadow of the left atrium.
Calcification of the cardiac valves is most often caused by rheumatic valvulitis, calcific aortic stenosis, or bacterial endocarditis and involves the mitral and aortic valves. In the frontal projection the mitral valve lies to the left of the spine, and as the heart beats, calcifications on this valve will describe a flat, elliptical trajectory extending downward and to the left. The aortic valve is usually projected over the left side of the spine and moves in a relatively straight line upward and slightly to the right. Because of the overlapping shadows of the vertebral bodies, small calcific deposits on the aortic valve may be difficult to detect in the frontal view but are readily seen in the lateral projection. The pulmonic (pulmonary) valve is projected to the left of the spine, higher than the aortic and mitral valves, and moves vertically with the cardiac pulsation. The tricuspid valve lies over the spine and moves in a horizontal plane.
Right Anterior Oblique Projection
The right anterior oblique (RAO) view is used mainly to evaluate left atrial enlargement and abnormalities of the right ventricular outflow (outlet) tract (see Plate 3-2). RAO is also the best projection in which to study calcification of the mitral valve. During selective left ventricular angiocardiography, the RAO view is used to evaluate mitral stenosis or insufficiency, because the mitral valve is seen tangentially, and the left atrium is projected entirely behind the left ventricle. RAO is the only view in which these two chambers do not overlap.
In a properly positioned RAO view, the shadow of the spine lies to the left of the cardiac silhouette, and a thin vertical band of air-containing lung separates the two structures. The aortic arch is foreshortened in this view, and the descending aorta partially overlaps the vertebral column.
The right border of the heart is formed by the right posterior aspect of the left atrium above and the posterior border of the right atrium below. As the obliquity of the projection is increased, more of the left atrium comes into profile.
The uppermost part of the left border of the cardiovascular silhouette is almost vertical and represents the ascending aorta. Just below this segment, the cardiac contour slopes downward and to the left in a gentle curve and is formed by the outflow tract (outlet) of the right ventricle and pulmonary trunk. The inferior continuation of this curve is formed by the anterior wall of the left ventricle. As in the frontal view, the body of the right ventricle is in contact with the diaphragm and cannot be visualized.
The mitral valve is seen almost tangentially and is projected over the midpart of the cardiac silhouette. Because confusing overlapping shadows are absent and the direction of mitral valve motion is perpendicular to the x-ray beam, calcification of the mitral valve is best detected fluoroscopically in RAO projection. The elliptical orbit of the valve is mainly directed horizontally. The aortic valve is thrown clear of the spine, and although in contact with the upper border of the mitral valve, calcification of the aortic valve can be recognized as it moves mostly in an up-and-down direction. This projection also provides the greatest separation of the aortic and pulmonic valves. The pulmonary valve lies at a level higher than the aortic valve and to its left, touching the left border of the cardiac silhouette. The line of motion of the pulmonic valve is directed upward and to the right. The tricuspid valve is seen almost tangentially and slightly behind the mitral valve. The tricuspid valve moves horizontally with the cardiac pulsation, and in the rare case of tricuspid calcification, the valve is easily mistaken for a calcified mitral valve.
Left Anterior Oblique Projection
Although no longer used for plain chest radiographs, the left anterior oblique (LAO) view is useful in evaluating the size of the left atrium and left ventricle during angiography (see Plate 3-3). The aortic arch is seen clearly because it is oriented approximately parallel to the film and is projected with minimal foreshortening. A selective left ventricular angiocardiogram in the left oblique projection is useful in the detection of a ventricular septal defect, because most of the muscular interventricular septum and a part of the membranous septum are seen tangentially.
The right border of the cardiac contour is formed by the right atrium above and the right ventricle below. As the degree of obliquity is increased, more of the right ventricle becomes border forming. Enlargement of the right atrium increases the convexity of the upper right cardiac border, whereas enlargement of the right ventricle usually results in a more generalized increase in curvature of the upper right border.
The left cardiac contour is formed mostly by the left ventricle, except for the upper quarter, which is contributed by the left posterior aspect of the left atrium, directly beneath the left main bronchus. Usually, in a proper 45-degree oblique view, the shadow of a normal left ventricle will not extend to the left of the shadow of the spine; extension of this shadow indicates an enlarged left ventricle. However, this sign must be evaluated cautiously because with lesser degrees of obliquity, or if the film is not made in full inspiration, a normal left ventricle may not clear the spine. If the stomach is distended with air, the diaphragmatic surface of the left ventricle can also be evaluated.
The left atrial part of the cardiac contour is usually straight or minimally convex. An outward bulging in this region denotes left atrial enlargement, probably its most sensitive radiographic sign. The esophagus is projected directly over the left atrial segment; therefore barium must not be given until after the LAO film has been made, because significant degrees of left atrial enlargement could be obscured. With greater degrees of enlargement, the dilated left atrium will push the left main bronchus upward and horizontally. The dilated atrium will also encroach on the clear space below the aortic arch.
The greatest length of the aortic arch is seen in the LAO view, and the origins of the great vessels are maximally separated. Thus, LAO is the best projection for identifying aneurysms of the aortic arch and for opacification studies of the aorta and great vessels.
The mitral valve is seen almost directly en face. As a result, calcific deposits on the cusps may be difficult to see because these move on an axis perpendicular to the fluoroscopic screen, with minimal horizontal excursion. In addition, the mitral valve may be hidden partially by the shadow of the spine. Calcification of the tricuspid valve can be differentiated from that of the mitral valve by the complete separation of the two valves in the LAO projection, with the tricuspid valve lying in the right half of the cardiac silhouette. The LAO view is well suited for identifying calcification of the aortic valve, which lies in the upper part of the cardiac silhouette, clear of the spine, and moves along an axis directed upward and to the right. The pulmonic valve is situated slightly higher than the aortic valve and moves upward and toward the left.
Lateral Projection
In plain radiographs the lateral view is used primarily for evaluating the presence of right ventricular (RV) enlargement, left atrial enlargement, and combined enlargement of the left atrium and left ventricle. Lateral is also the best view for distinguishing between calcification of the aortic and mitral valves. A selective RV angiocardiogram in the lateral projection provides the best visualization of the outflow part of the right ventricle and the pulmonic valve (see Plate 3-4).
In the lateral view the heart is projected clear of the confusing shadows of the sternum and spine. The anterior margin of the cardiac silhouette is formed by the apex and the RV outflow tract. Normally, this abuts on the lower quarter or third of the anterior chest wall. The upper two thirds of the anterior cardiac contour is formed by two convex arcs, slanting upward and posteriorly. The lower, more oblique arc is formed by the RV outflow tract, and the upper arc is formed by the ascending aorta. The radiolucent lung is interposed between these two arcs and the sternum. As it enlarges, the right ventricle bulges forward and progressively obliterates the retrosternal space. Similarly, dilatation and tortuosity, or an aneurysm of the ascending aorta, will encroach on the upper retrosternal space. The upper margin of the aortic arch distal to the origin of the great vessels can usually be identified in the lateral view.
The posterior border of the heart is formed mostly by the posterior wall of the left atrium. Just above the diaphragm, small parts of the right atrium and IVC come into profile. When enlarged, however, the left ventricle may extend farther posteriorly than the right atrium, forming the lower part of the posterior heart border as well. The esophagus lies immediately behind the left atrium and left ventricle, and an enlargement of these chambers will indent the anterior wall of the esophagus and displace it posteriorly. If only the left atrium is enlarged, the indentation on the esophagus is localized at the level of the upper half of the cardiac silhouette; the lower part of the esophagus is in its normal position. When the left ventricle is enlarged as well, it also pushes the esophagus posteriorly, and the backward curve of the displaced esophagus is then continuous over the entire length of the cardiac silhouette to the diaphragm.
Identifying localization of a calcific deposit in the mitral or aortic valve may be difficult. This problem can be resolved in the lateral view. If a line is drawn from the anterior costophrenic sulcus to the point of bifurcation of the trachea, the aortic valve will lie above and in front of this line, whereas the mitral valve will be below and posterior. The mitral valve moves more or less horizontally in the lateral view, and the aortic valve moves on a vertical axis that is tilted slightly anteriorly and upward. The pulmonic valve is located above the aortic valve and more anteriorly, extending to the anterior border of the cardiac shadow.
Angiocardiography
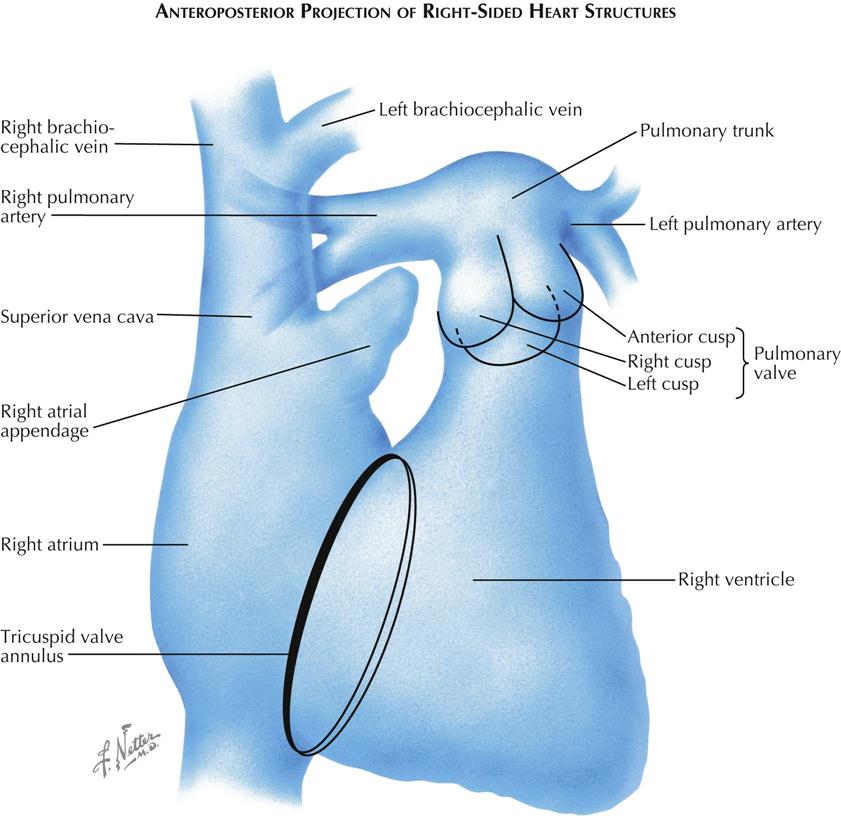
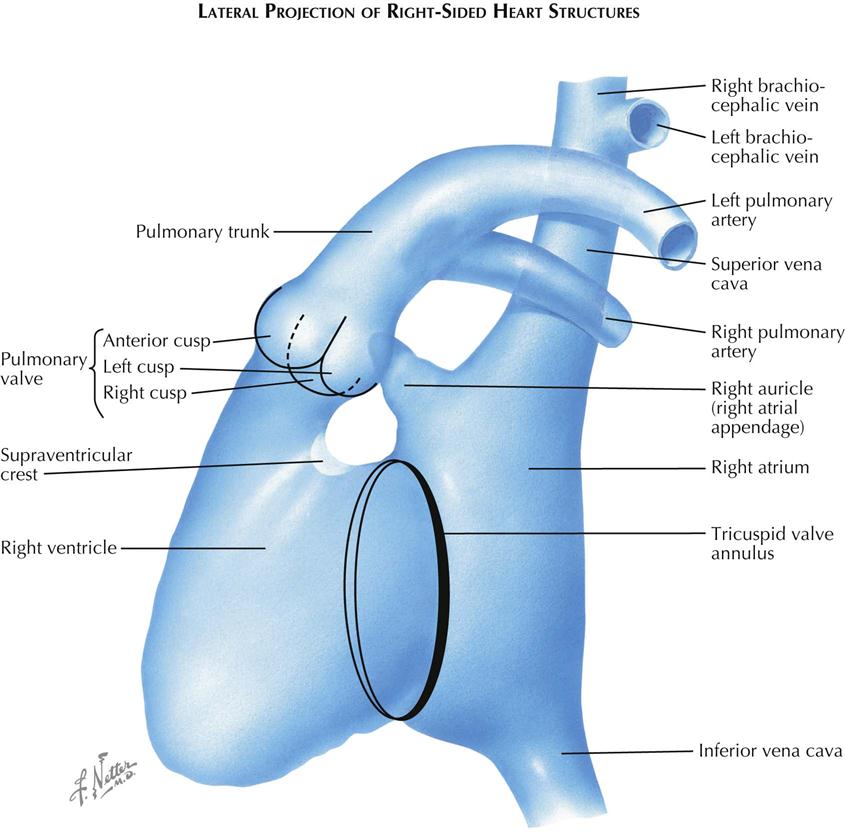
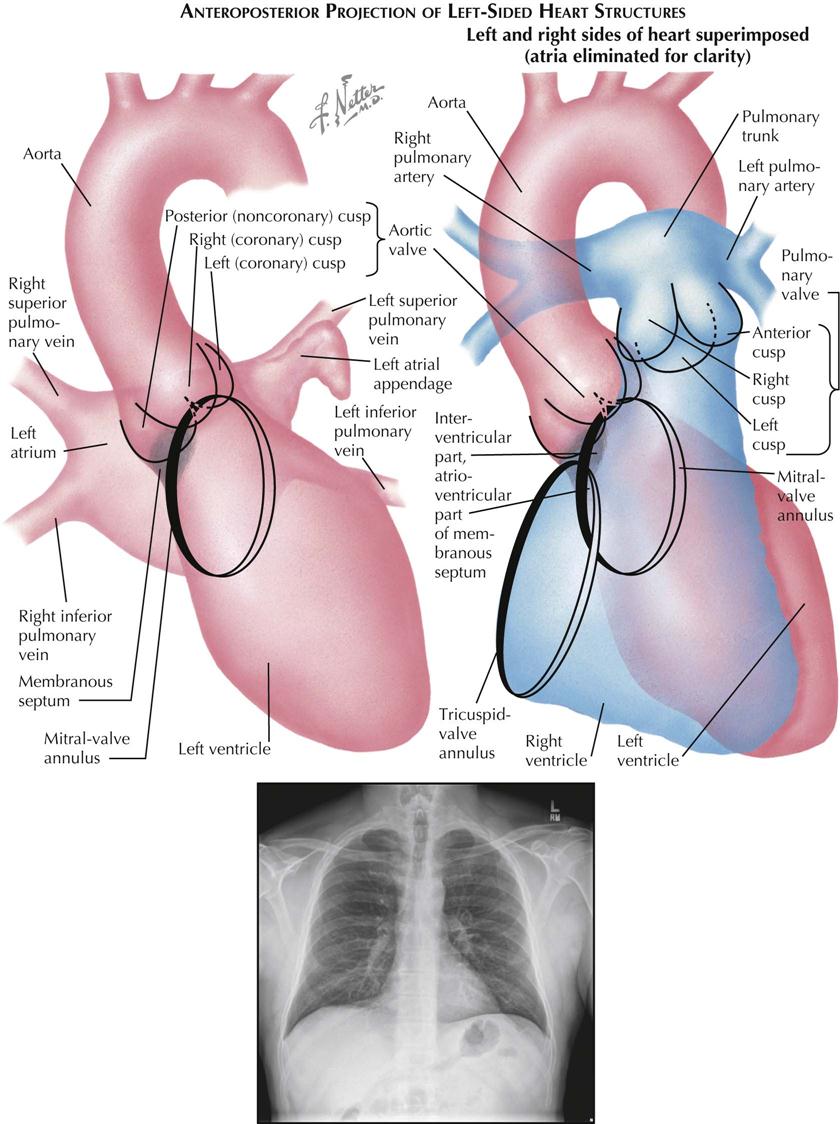
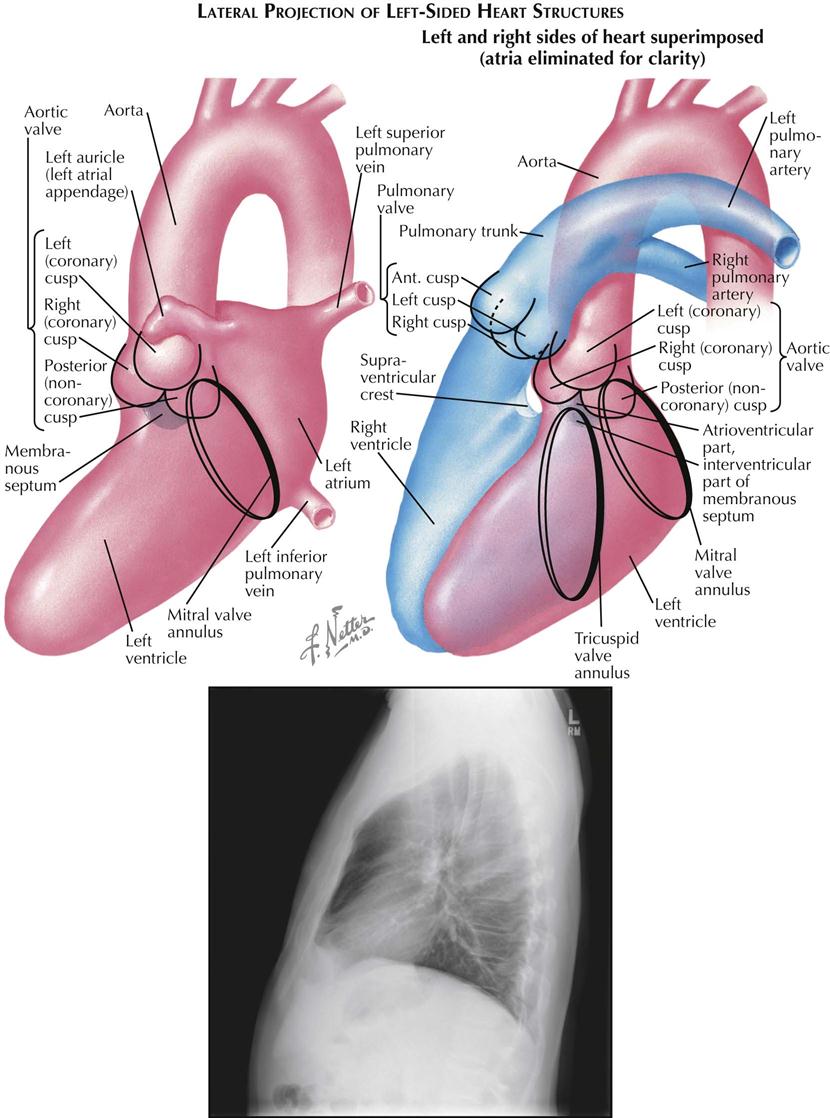
Chest radiography and fluoroscopy demonstrate only the outer borders of the heart and great vessels. Considerably more information is obtained when the blood is opacified by introducing a radiopaque contrast medium into the vascular system to visualize the inner borders of the cardiac chambers and vessels during catheter-based angiography. The structure and motion of the cardiac valves can be studied, as well as cardiac and pulmonary hemodynamics.
The basic requirements for successful catheter-based angiocardiography are (1) rapid injection of the radiopaque contrast material so that it flows as a bolus and (2) cineangiography of the heart to follow the course of the contrast material. The iodinated contrast medium can be injected into a peripheral vein, where it is carried to the heart through the SVC or IVC, or it can be injected through a catheter directly into a specific cardiac chamber, great vessel, or coronary artery. The latter technique, selective angiocardiography, provides greater anatomic detail because the contrast material reaches the chamber as a denser, more compact bolus and is not diluted in the right atrium by nonopaque blood.