Section 26 Emergency Medical Systems
26.1 Pre-hospital emergency medicine
Dispatch
In order to have consistent, accurate dispatch of the appropriate skill set in the optimal time frame, many ambulance services are now using computer-aided dispatch programs. These computer programs have structured questions for use by call-takers with some limited medical training. Pivotal to accurate dispatch is identification of the chief complaint, followed by subsequent structured questions to determine the severity of the illness. The answers to these questions allow the computerized system to recommend the optimal skill set and speed of response. This computer algorithm is medically determined according to local protocols and practices and provides consistency of dispatch.
Despite continuous developments in computer algorithms, accurate telephone identification of life-threatening conditions may be difficult. For example, identification of patients who are deceased (beyond resuscitation),1 in cardiac arrest2 or suffering acute coronary syndrome3 has been shown to lack the very high sensitivity and specificity that might be expected.
The dispatch centre also has a role for telephone instructions on bystander cardiopulmonary resuscitation4 and first aid.
Clinical skills
The rationale for common pre-hospital interventions is outlined in the following sections.
Trauma care
Basic trauma life support
The initial assessment of the airway and breathing includes the application of cervical immobilization in patients who have a mechanism of injury that suggests a risk of spinal column instability. Although decision instruments have been developed to identify patients in the ED who require radiographic imaging,5 the accuracy of these guidelines in the pre-hospital setting is uncertain. On the other hand, spinal immobilization of many patients with minimal risk of spinal cord injury is uncomfortable, mandates transport and possibly leads to unnecessary radiographic studies.6 Therefore, ambulance officers are generally instructed to immobilize the neck in all cases of suspected spinal-column injury based largely on mechanism of injury.
Accurate triage of major trauma patients is an important component of trauma care in cities with designated major trauma centres. Triage tools based on vital signs, injuries and modifying factors such as age, comorbidities and mechanism of injury are used.7 Paramedic judgement may also have a role, although some injuries such as occult intra-abdominal injuries are difficult to detect on clinical grounds.8
Advanced trauma life support
Advanced trauma life support (ATLS) by ambulance paramedics, particularly intubation of the trachea and i.v. cannulation for fluid therapy, is controversial. Although these interventions are routinely used after hospital admission, studies to date indicate that the provision of ATLS provided by paramedics does not improve outcomes.9,10 On the other hand, few studies conducted to date have been sufficiently rigorous to allow definitive conclusions, and many were conducted in cities with predominantly penetrating trauma rather than blunt trauma. Many ambulance services therefore continue to authorize advanced airway management and i.v. fluid resuscitation in selected trauma patients.
Intubation
Following severe head injury, many unconscious patients have decreased oxygenation and ventilation during pre-hospital care, and this secondary brain injury is associated with worse neurological outcome.11 In addition, a depressed gag or cough reflex may lead to aspiration of vomit and this may cause a severe pneumonitis, which may be fatal or result in a prolonged stay in an intensive-care unit. To prevent these complications of severe head injury, endotracheal intubation may be performed. This facilitates control of oxygen and carbon dioxide, provides airway protection and is recommended for patients with Glasgow Coma Score <9 following severe head injury.12 However, most patients with severe head injury maintain a gag or cough reflex, and successful intubation requires the use of drugs to facilitate laryngoscopy and placement of the endotracheal tube.
There is some evidence that pre-hospital intubation in head injury is beneficial.13,14 In a study of 671 patients with severe head injury, intubation in the field using RSI was associated with a decrease in mortality rate from 56% to 36%.13 In another study of 799 patients with severe head injury, patients intubated using RSI in the field were compared with those not intubated.14 When adjusted for confounding variables, the RSI patients were more likely to survive (odds ratio, 0.63; 95% confidence interval, 0.41–0.97; P = 0.04) and have a good outcome (odds ratio, 1.7; 95% confidence interval, 1.2–2.6; P = 0.006) than those in the no-RSI group.
However, two studies have suggested that pre-hospital intubation may be associated with worse outcome in head trauma patients.15,16 In a review of registry data of patients admitted to an urban trauma centre with severe head injury, patients were stratified by pre-hospital methods of airway management (not intubated, intubated or unsuccessful intubation).15 This study showed that patients requiring pre-hospital intubation or in whom intubation was attempted had an increased mortality (81% and 77%, respectively) when compared with non-intubated patients (43%).
In a second study, RSI was introduced as a protocol for adult patients with severe head injury in San Diego, USA, and the effect on outcome was assessed compared with historical controls.16 Each study patient was hand-matched to three non-intubated historical controls from a trauma registry using the following parameters: age, sex, mechanism of injury, trauma centre and AIS score for each body system. The study enrolled 209 trial patients who were hand-matched to 627 controls. Both groups were similar with regard to all matching parameters, admission vital signs, frequency of specific head injury diagnoses and incidence of invasive procedures. Mortality was significantly increased in the RSI cohort versus controls for all patients (33.0% versus 24.2%, P < 0.05).
Given the limited evidence of benefit and potential for harm with intubation in head injury, it has been proposed that intubation not be introduced into paramedic practice until prospective randomized, controlled trials have been conducted.17
Intravenous fluid
Intravenous fluid resuscitation has been shown to worsen outcome in patients with penetrating trauma and hypotension.18 However, this finding has recently been questioned.19 In any case, most major trauma in Australasia and Europe is blunt rather than penetrating and few patients require urgent surgical control of haemorrhage. The issue of pre-hospital i.v. fluid for the treatment of hypotension therefore remains the subject of debate.
Supporters of pre-hospital i.v. fluid therapy suggest that this treatment is intuitively beneficial and that any delay of this therapy increases the adverse effects of prolonged hypotension, which may result in end-organ ischaemia, leading to multiorgan system failure and increased morbidity and mortality. In particular, hypotension after severe head injury is associated with an adverse outcome and should be promptly treated.20
There is no evidence from clinical trials for benefit of the administration of i.v. fluid to bleeding patients in the pre-hospital setting. Studies to date suggest that pre-hospital i.v. fluid does not improve outcomes.8,9 Nevertheless, if i.v. fluid is given to patients with hypotension and severe head injury, crystalloid rather than colloid should be given.21
Analgesia
Inhaled analgesic treatments include methoxyflurane and oxygen/nitrous oxide. However, while the former is reasonably effective,22 there are concerns with the administration of these in enclosed spaces such as ambulances because of the perceived risk of repeated exposures of these analgesics to the ambulance officers.
Alternatively, the training of ambulance officers in the insertion of an i.v. cannula and administration of small increments of i.v. morphine is increasingly regarded as a feasible alternative to inhalation analgesia. Alternative routes of narcotic administration such as intranasal administration are the subject of current studies. For example, the use of intranasal fentanyl has been shown to be equivalent to i.v. morphine.23
Cardiac care
Cardiac arrest
The patient in cardiac arrest represents the most time-critical patient attended by ambulance services. For the patient with ventricular fibrillation, each minute increase from time of collapse to defibrillation is associated with an increase in mortality of approximately 10%. However, most ambulance services have urban response times that average 8–9 min. Since there may be 2 min between collapse and dispatch, and 1 min between arrival at the scene to delivery of the first defibrillation, total time from collapse to defibrillation would usually be approximately 12 min, therefore current survival rates for witnessed cardiac arrest due to defibrillation in urban areas are low24 and there are even fewer survivors in rural areas.25
The most effective strategy to improve outcomes would be to decrease ambulance response times. However, this would require very significant increases in ambulance resources and would be an expensive strategy in terms of cost per life saved. Alternatively, response times to cardiac arrest patients may be reduced with the use of co-response by first responders equipped with defibrillators. Such first responder programmes have been introduced in Melbourne, Australia,26 and Ontario, Canada,27 with promising results.
The role of advanced life support (intubation and i.v. drug therapy) during cardiac arrest remains controversial. When these skills were introduced into Ontario, Canada, there was an increase in the numbers of patients with return of spontaneous circulation (10.9% versus 14.6%, P < 0.001) but the rate of survival to hospital discharge did not significantly increase (5.0% versus 5.1%, P = 0.83).28
Acute coronary syndromes
Most ambulance services have protocols for the management of the patient with chest pain where the cause is suspected as an acute coronary syndrome. These protocols usually include supplemental oxygen, administration of sublingual trinitrates and aspirin, followed by rapid transfer to an ED for definitive diagnosis and management. In addition, pain relief using i.v. morphine may be given by advanced life-support paramedics. Whilst these interventions may decrease symptoms, more recent strategies to improve outcomes involve triage of patients with ST-segment elevation myocardial ischaemia (using 12-lead electrocardiography) by paramedics to centres for interventional cardiology.29
Cardiac arrhythmias
Some patients with an acute coronary syndrome develop a cardiac arrhythmia during ambulance care. Pulseless ventricular tachycardia is treated with immediate defibrillation, and amiodarone is recommended for ventricular tachycardia where a pulse is palpable.30 However, the pre-hospital drug treatment of supraventricular tachycardia is more controversial. Whilst the use of verapamil or adenosine appears to be equivalent in efficacy,31 many ambulance services require the patient to be transported for 12-lead electrocardiography and management of the tachyarrhythmia in an ED.
Pulmonary oedema
During myocardial ischaemia, the patient may develop pulmonary oedema and in these patients the use of oxygen and glyceryl trinitrates is regarded as useful.32 Despite common use in the ED, pre-hospital continuous positive airways pressure for acute pulmonary oedema has not been widely adopted, since the equipment is expensive, oxygen consumption is high and there is no proven benefit with pre-hospital administration of continuous positive airways pressure at this time.
Other medical emergencies
Hypoglycaemia
The patient with hypoglycaemia due to relative excess of exogenous injected insulin will suffer neurological injury unless the blood glucose level is promptly corrected. Treatment involves orally or intravenously administered dextrose. For ambulance officers who are not trained to insert i.v. cannulae, or where i.v. access is not possible, the administration of intramuscular glucagon is also effective, although this is associated with an increase in the time to full consciousness.33
Narcotic overdose
Patients who inject narcotic drugs may suffer coma and respiratory depression which is readily reversed by naloxone. However, the administration of i.v. naloxone by paramedics is somewhat problematic, since i.v. access may be difficult and the half-life of i.v. naloxone (approximately 20 min) may be shorter than the injected narcotic. If the patient awakens and leaves medical care, there may also be a recurrence of sedation. Many ambulance services therefore administer naloxone via the intramuscular or subcutaneous route. Whilst the absorption via this route may be slower, overall the time to return of normal respirations is equivalent. To avoid the use of needles, naloxone may also be administered via the intranasal route and this has an equivalent onset time to intramuscular naloxone.34
Anaphylaxis
Many patients with known severe anaphylaxis are prescribed adrenaline (epinephrine) by their physician for self-administration. The use of intramuscular adrenaline (epinephrine) by ambulance officers is a safe and effective pre-hospital therapy.35
Seizures
Out-of-hospital status epilepticus is also regarded as a time critical medical emergency. The first-line treatment of status epilepticus is usually a benzodiazepine. There are supportive data on the use of intramuscular midazolam, which may have an initial success rate of 80%.36 Intravenous benzodiazepines may given if seizures persist.
Controversies and future directions
1 Harvey L, Woollard M. Outcome of patients identified as dead (beyond resuscitation) at the point of the emergency call. Emergency Medicine Journal. 2004;21:367-369.
2 Flynn J, Archer F, Morgans A. Sensitivity and specificity of the medical priority dispatch system in detecting cardiac arrest emergency calls in Melbourne. Prehospital and Disaster Medicine. 2006;21:72-76.
3 Deakin CD, Sherwood DM, Smith A, et al. Does telephone triage of emergency (999) calls using Advanced Medical Priority Dispatch (AMPDS) with Department of Health (DH) call prioritisation effectively identify patients with an acute coronary syndrome? An audit of 42,657 emergency calls to Hampshire Ambulance Service NHS Trust. Emergency Medicine Journal. 2006;23:232-235.
4 Vaillancourt C, Verma A, Trickett J, et al. Evaluating the effectiveness of dispatch-assisted cardiopulmonary resuscitation instructions. Academic Emergency Medicine. 2007;14:877-883.
5 Hoffman JR, Mower WR, Wolfson AB, et al. Validity of a set of clinical criteria to rule out injury to the cervical spine in patients with blunt trauma. National Emergency X-Radiography Utilization study (NEXUS) Group. New England Journal of Medicine. 2000;343:94-99.
6 Armstrong BP, Simpson HK, Crouch R, et al. Prehospital clearance of the cervical spine: does it need to be a pain in the neck? Emergency Medicine Journal. 2007;24:501-503.
7 Markovchick VJ, Moore EE. Optimal trauma outcome: trauma system design and the trauma team. Emergency Medicine Clinics of North America. 2007;25:643-654.
8 Mulholland SA, Gabbe BJ, Cameron P. Victorian State Trauma Outcomes Registry and Monitoring Group (VSTORM). Is paramedic judgement useful in prehospital trauma triage? Injury. 2005;36:1298-1305.
9 Isenberg DL, Bissell R. Does advanced life support provide benefits to patients? A literature review. Prehospital Disaster Medicine. 2005;20:265-270.
10 Liberman M, Mulder D, Lavoie A. Multicenter Canadian study of prehospital trauma care. Annals of Surgery. 2003;237:153-160.
11 Chi JH, Knudson MM, Vassar MJ, et al. Prehospital hypoxia affects outcome in patients with traumatic brain injury: a prospective multicenter study. Journal of Trauma. 2006;61:1134-1141.
12 www.braintrauma.org/prehospital. (accessed January 2008)
13 Winchell RJ, Hoyt DB. Endotracheal intubation in the field improves survival in patients with severe head injury. Archives of Surgery. 1997;132:592-597.
14 Bulger EM, Copass MK, Sabath DR. The use of neuromuscular blocking agents to facilitate prehospital intubation does not impair outcome after traumatic brain injury. Journal of Trauma. 2005;58:718-723.
15 Murray JA, Demetriades D, Berne TV, et al. Prehospital intubation in patients with severe head injury. Journal of Trauma. 2000;49:1065-1070.
16 Davis DP, Hoyt DB, Ochs M, et al. The effect of paramedic rapid sequence intubation on outcome in patients with severe traumatic brain injury. Journal of Trauma. 2003;54:444-453.
17 Bernard SA. Paramedic intubation of patients with severe head injury: a review of current Australian practice and recommendations for change. Emergency Medicine of Australasia. 2006;18:221-228.
18 Bickell W, Pepe P, Mattox K, et al. Immediate versus delayed fluid resuscitation for hypotensive patients with penetrating torso injuries. New England Journal of Medicine. 1994;331:1105-1108.
19 Yaghoubian A, Lewis RJ, Putnam B. Reanalysis of prehospital intravenous fluid administration in patients with penetrating truncal injury and field hypotension. American Surgicals. 2007;73:1027-1030.
20 Chesnut RM, Marshall LF, Klauber MR, et al. The role of secondary brain injury in determining outcome from severe head injury. Journal of Trauma. 1993;34:216-222.
21 SAFE Study Investigators. Saline or albumin for fluid resuscitation in patients with traumatic brain injury. New England Journal of Medicine. 2007;357:874-884.
22 Buntine P, Thom O, Babl F, et al. Prehospital analgesia in adults using inhaled methoxyflurane. Emergency Medicine Australasia. 2007;19:509-514.
23 Rickard C, O’Meara P, McGrail M, et al. A randomized controlled trial of intranasal fentanyl vs intravenous morphine for analgesia in the prehospital setting. American Journal of Emergency Medicine. 2007;25:911-917.
24 Fridman M, Barnes V, Whyman A, et al. A model of survival following pre-hospital cardiac arrest based on the Victorian Ambulance Cardiac Arrest Register. Resuscitation. 2007;75:311-322.
25 Jennings PA, Cameron P, Walker T, et al. Out-of-hospital cardiac arrest in Victoria: rural and urban outcomes. Medical Journal of Australia. 2006;185:135-139.
26 Smith KL, McNeill JJ. The Emergency Medical Response Steering Committee. Cardiac arrests treated by ambulance paramedics and fire fighters. Medical Journal of Australia. 2002;177:305-309.
27 Stiells IG, Wells GA, Field BJ, et al. Improved out-of-hospital cardiac arrest survival through the inexpensive optimization of an existing defibrillation program. Journal of American Medical Association. 1999;281:1175-1181.
28 Stiell IG, Wells GA, Field B, et al. Advanced cardiac life support in out-of-hospital cardiac arrest. New England Journal of Medicine. 2004;351:647-656.
29 Le May MR, Davies RF, Dionne R, et al. Comparison of early mortality of paramedic-diagnosed ST-segment elevation myocardial infarction with immediate transport to a designated primary percutaneous coronary intervention center to that of similar patients transported to the nearest hospital. American Journal of Cardiology. 2006;98:1329-1333.
30 Morley PT, Walker T. Australian Resuscitation Council. Australian Resuscitation Council: adult advanced life support (ALS) guidelines 2006. Critical Care and Resuscitation. 2006;8:129-131.
31 Madsen CD, Pointer JE, Lynch TG. A comparison of adenosine and verapamil for the treatment of supraventricular tachycardia in the prehospital setting. Annals of Emergency Medicine. 1995;25:649-655.
32 Stiell IG, Spaite DW, Field B, et al. Advanced life support for out-of-hospital respiratory distress. New England Journal of Medicine. 2007;356:2156-2164.
33 Howell MA, Guly HR. A comparison of glucagon and glucose in prehospital hypoglycaemia. Journal of Accident Emergency Medicine. 1997;14:30-32.
34 Kelly AM, Kerr D, Dietze P, et al. Randomised trial of intranasal versus intramuscular naloxone in prehospital treatment for suspected opioid overdose. Medical Journal of Australia. 2005;182:24-27.
35 Kane KE, Cone DC. Anaphylaxis in the prehospital setting. Journal of Emergency Medicine. 2004;27:371-377.
36 Vilke GM, Sharieff GQ, Marino A. Midazolam for the treatment of out-of-hospital pediatric seizures. Prehospital Emergency Care. 2002;6:215-217.
26.2 Retrieval
Introduction
Retrieval medicine is a term used to describe the branch of emergency medicine involved with the retrieval and transport of patients from remote locations to primary hospital treatment sites. Retrieval is the process whereby medical teams are transported from central hospitals to peripheral areas with the intention of treating, stabilizing and transporting patients back to these centres. The most common form is a secondary retrieval where the patient is transferred from one healthcare facility to another. A primary retrieval is where the patient is retrieved direct from the scene or incident. However, the clinical distinction between primary and secondary retrieval becomes less clear in smaller healthcare settings where staff are unfamiliar with management of critically ill or injured patients.
Principles of transport
Another principle of retrieval is that the level of medical care should be maintained or increased at each stage of transfer from the peripheral to central institution. This principle has been adopted in the recommendations of the specialties involved in critical care transport in Australasia.1 In general the retrieval team can provide a level of care above that available at the referring hospital whereas a team sent from the peripheral hospital will be unable to provide the same level of care in transit as in their own hospital. Utilizing peripheral location staff as transport escorts may also significantly affect the level of care available at that location. For smaller locations this effect may be critical.
Modes of transport
When road transport can be achieved in less than 1–2 h this is normally the cost-effective choice. Transport by air has the disadvantages of increased cost and problems associated with altitude. The greatest benefit of aerial transport is in reducing the time spent in transit and therefore minimizing the time at greatest risk. Figure 26.2.1 shows the time differences between road and helicopter transport. Overall time from incident to arrival at a central hospital may not be significantly reduced by use of a helicopter if road transport is immediately available. The time spent in transit, however, and therefore the time the patient is exposed to maximum risk is significantly reduced. The time taken for the retrieval team to reach a patient is also minimized by use of helicopter transport where road distances are prolonged. The choice between helicopter and fixed wing is a balance between avoiding secondary transfers with helicopters and more rapid flight with fixed wings. Beyond helicopter flight times of 1 h (~250 km), fixed wing becomes increasingly preferable. Helicopter use beyond 400 km is unusual. The transport distance, terrain covered and the specific condition of the patient all influence the decision on the mode of transportation. It is inappropriate to transport some patients by air whilst others require pressurization to ground level in order to be transported safely. The final decision will rest with the retrieval team familiar with the options available. The team should be contacted early in order to arrange the most appropriate transport as quickly as possible.
Preparation for transport
It is very difficult to perform even simple tasks in a moving vehicle. Planes and helicopters are particularly noisy, with unexpected movements and light fluctuations. Listening to heart sounds, respiratory sounds or even taking a pulse may be impossible. Despite this there are several examples in the literature of the successful performance of intubation, i.v. cannulation and other procedures in aircraft.2,3 Although this is reassuring, it should be considered a failure of pre-flight preparation to be put in the position where such a procedure becomes necessary in transit. If a procedure is considered to be likely or possibly needed before arrival at the destination, serious consideration should be given to performing that procedure prior to departure. It is essential to have lines secured, redundant lines operational in case of emergencies and contingency plans prepared for common and potentially serious complications. In practice this means checking that each vascular access is patent, operational and sufficiently secured to withstand sudden turbulence. Some lines may require suturing to achieve this. It is prudent to have at least one i.v. line that is patent but not in use in order to provide access in an unexpected emergency. Wherever practicable, a single port at a secure site can be used for several infusions, thus freeing up other ports.
Common problems in transit
Monitoring and equipment used in transport should be in accordance with recommended standards. Detailed guidelines are available.1 Most patients require continuous ECG, pulse oximetry and blood pressure monitoring as a minimum. Equipment must be selected carefully. Display screens must be visible in daylight and battery life must be appropriate for duration of transport with a large capacity for additional work. It should always be assumed that the next task will occur immediately without the opportunity to recharge batteries. Consideration should be given to the placement of invasive lines for potentially unstable patients. Use of arterial lines significantly reduces battery requirements for non-invasive blood pressure monitoring. Equipment alarms must be clearly visible as auditory alarms are difficult or impossible to hear in moving vehicles, especially helicopters.
Special problems associated with travelling at altitude
All transport is associated with motion sickness. Staff tend to become accustomed whilst first-time travellers (including patients) are most affected. Antiemetics should be discussed and administered early whenever possible. Another related condition reducing performance of medical attendants is the sopite syndrome. This condition is characterized by yawning, drowsiness, disinclination for either physical or mental work and lack of participation in group activities.4 It is not directly related to the degree of turbulence, is not responsive to anti-motion-sickness medications and, unlike motion sickness, there appears to be little adaptation with time. As many as two-thirds of air attendants are affected to some degree, making this condition an important cause of reduced performance during transport.5
Expansion of trapped gas can produce disastrous consequences. All pneumothoraces, no matter how small, require venting prior to elevation to altitude. Heimlick valves are preferable to underwater seal drains during transport as underwater seal apparatus is disturbed by movement and is at greater risk of damage and breakage. Air trapped in small bowel (for instance in ileus) can produce considerable discomfort. Gas at either end of the gastrointestinal tract can vent spontaneously. Middle ear gas can be particularly painful on ascent and descent. Patients should be taught equalization techniques such as the Valsalva, Frenzel or Toynbee manoeuvres. The Valsalva manoeuvre reduces cardiac venous return and may be associated with syncope. The most effective technique is the Frenzel manoeuvre, which is carried out with the mouth, nostrils and epiglottis closed. Air in the nasopharynx is then compressed by the action of the muscles of the mouth and tongue. This technique not only generates higher nasopharyngeal pressures than the Valsalva manoeuvre, it opens the eustachian tubes at lower pressures. The easiest taught technique is the Toynbee manoeuvre, which raises pharyngeal pressure by swallowing with the mouth closed and nostrils occluded. In babies and young children, the angle of entry of the eustachian tubes into the nasopharynx is less acute and hence ear problems in flight are less common. Older children and some adults are unable to learn the various techniques.6 Nasal decongestants prior to departure or sweets to suck on during descent may be of benefit. If a patient is unable to clear the ears, pain increases until the tympanic membrane ruptures with sudden relief of discomfort.
Expansion of gases has consequences for medical equipment. Air in an endotracheal tube cuff expands, increasing cuff pressure on ascent. Although modern high-volume, low-pressure cuffs minimize this effect, the increased pressure may cause tracheal damage if left unchecked for long periods. On descent the volume falls, making tube displacement more likely and increasing the likelihood of leakage around the cuff. Loss of ventilatory volumes and aspiration may result. The cuff pressure should be monitored on ascent and descent and adjusted as necessary. An alternative practice is to fill the cuff with sterile fluid such as normal saline. This makes it impossible to monitor the pressure in the cuff and is not necessary if the air-filled cuff is monitored appropriately.
Future directions
1 Joint Faculty of Intensive Care Medicine and Australasian and New Zealand College of Anaesthetist and Australasian College for Emergency Medicine. Minimum Standards for the Transport of Critically Ill Patients, 2003. http://acem.org.au/media/policies_and_guidelines/min_standard_crit_ill.pdf. (updated February accessed August 2007)
2 Boyle MF, Hatton D, Sheets C. Surgical cricothyrotomy performed by air ambulance flight nurses: a 5-year experience [see comments]. Journal of Emergency Medicine. 1993;11(1):41-45.
3 Harrison T, Thomas SH, Wedel SK. In-flight oral endotracheal intubation. American Journal of Emergency Medicine. 1997;15(6):558-561.
4 Graybiel A, Knepton J. Sopite syndrome: a sometimes sole manifestation of motion sickness. Aviation, Space Environmental Medicine. 1976;47(8):873-882.
5 Wright MS, Bose CL, Stiles AD. The incidence and effects of motion sickness among medical attendants during transport. Journal of Emergency Medicine. 1995;13(1):15-20.
6 Harding MH, Mills FJ. Aviation Medicine, 2nd edn. London: British Medical Association, 1988.
7 Nicholl JP, Brazier JE, Snooks HA. Effects of London helicopter emergency medical service on survival after trauma [see comments]. British Medical Journal. 1995;311(6999):217-222.
26.3 Medical issues in disasters
Definitions and classification
There is no internationally accepted definition of disaster or disaster classification. There are, however, increasingly consistent uses of terms among stakeholder organizations. Common to most definitions is the concept that following a disaster the capacity of the impacted community to respond is exceeded and there is, therefore, a need for external assistance. The World Health Organization characterizes a disaster as a phenomenon that produces large-scale disruption of the normal healthcare system, presents an immediate threat to public health and requires external assistance for response. The Australian Emergency Manual defines disaster as an event that overwhelms normal community and organizational arrangements and requires extraordinary responses to be instituted. The Center for Research on the Epidemiology of Disasters (CRED), which compiles the data behind the annual World Disasters Report of the International Federation of Red Cross and Red Crescent Societies, stipulates a quantitative surveillance definition involving one of the following: 10 or more people killed, 100 or more people affected, declaration of state of emergency or an appeal for international assistance.1
Disaster management is the range of activities designed to establish and maintain control over disaster and emergency situations, and to provide a framework for helping at-risk populations avoid or recover from the impact of a disaster. It addresses a much broader array of issues than health alone, including hazard identification, vulnerability analysis and risk assessment. Disaster medicine can be defined as the study and application of clinical care, public health, mental health and disaster management to the prevention, preparedness, response and recovery from the health problems arising from disasters.2 This must be achieved in cooperation with other agencies and disciplines involved in comprehensive disaster management. In practice, emergency medicine and public health are the two specialties most intimately involved in disaster medicine.
Disasters are commonly classified as natural versus technological/human-generated (Box 26.3.1).1 Disasters may also be classified according to other characteristics, including acute versus gradual onset, short versus long duration, unifocal versus multifocal distribution, common versus rare and primary versus secondary. Classifications of disaster magnitude exist for selected natural hazards, such as earthquakes and hurricanes/cyclones; however, there is currently no standard classification of severity of disaster impact.
Box 26.3.1 Classification of disasters
Natural | Human-generated |
---|---|
Acute onset | Technological |
Hydrometeorological | Industrial accidents |
Avalanches, landslides | Explosions |
Bush fires, forest fires | Fires |
Extreme temperatures Floods | Hazardous material releases |
Windstorms | Structural collapses |
Transportation crashes | |
Geophysical | Air |
Earthquakes | Rail |
Tsunamis | Road |
Volcanic eruptions | Water |
Other | Terrorism |
Epidemics | |
Gradual/chronic onset Desertification | War/Complex emergencies |
Droughts/famines | |
Insect/pest infestations (e.g. locusts) |
Epidemiology
Globally, the types of disasters associated with the greatest numbers of deaths are complex emergencies (CEs). These are crises characterized by political instability, armed conflict, large population displacements, food shortages and collapse of public health infrastructure. Because of insecurity and poor access to the affected population, aggregate epidemiological data for CEs are somewhat limited. However, between 1998 and 2004 in the eastern region of the Democratic Republic of Congo, 3.9 million people lost their lives due to the consequences of the major humanitarian crisis afflicting that country.3 Incredibly, this was more than three times the total number of deaths globally due to natural and technological disasters during the decade of the 1990s. Based on United Nations definitions, there were 27 ongoing CEs in 2007, involving over 30 countries and impacting on the lives of hundreds of millions of people.4 Thirteen (44%) of these crises were ongoing in Africa, with 10 (37%) occurring in Asia.
According to information compiled by the International Federation of the Red Cross, there has been a significant increase in the total number of natural and technological disasters worldwide during the past 30 years. From 1996 to 2005, an average of approximately 641 such disasters was documented annually, peaking at 801 in 2000. While the total number of people killed by natural and technological disasters is approximately 93 000 per year, there is a wide annual range (21 888 in 2000 to 251 768 in 2004 due to the Indian Ocean tsunami). Moreover, the total number affected has almost trebled over the past three decades. It is estimated that approximately 250 million people are directly affected on an annual basis. Selected data are presented in Figures 26.3.1 and 26.3.2.
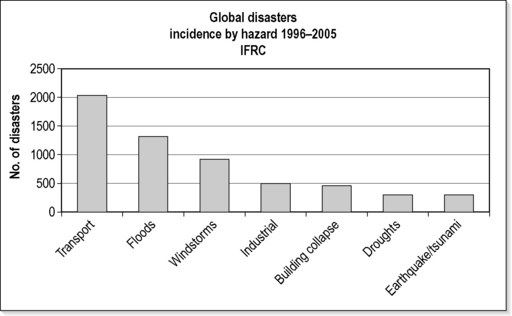
Fig. 26.3.1 Global disasters incidence by hazard 1996–2005.
Adapted from: International Federation of the Red Cross. World Disasters Report 2006: Focus on neglected crises. IFRC, Geneva; 2006.
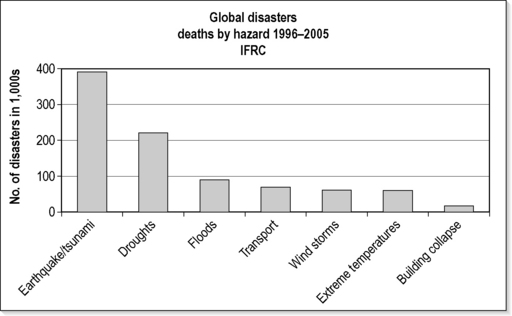
Fig. 26.3.2 Global disasters deaths by hazard 1996–2005.
Adapted from: International Federation of the Red Cross. World Disasters Report 2006: Focus on neglected crises. IFRC, Geneva; 2006.
The commonest types of disasters across the globe are transportation incidents, floods, windstorms, industrial incidents, building collapses, droughts/famines and earthquakes/tsunamis (see Fig. 26.3.1). Asia is the region of the world most prone to natural and technological disasters, recording 41% of such incidents between 1996 and 2005. It is followed by Africa (22%), the Americas (20%), Europe (14%) and Oceania (3%). Compared with other regions of the world, Australasia and Oceania clearly have a relatively low incidence of disasters. Over the past 10 years the commonest causes of natural disasters in Australia have been floods, severe storms and cyclones. Nationally, an average of 33 lives are lost per year due to disasters in Australia. In addition, over 68 000 people are affected annually, through injury, displacement, financial loss, damage or loss of homes and businesses. Historically, the leading causes of death from natural disasters have been heatwaves, followed by cyclones, floods and bushfires. Human-generated disasters resulting in multiple casualties have occurred more frequently in Australia in recent years. The commonest causes of mass casualty incidents have been bus crashes, structural fires, mining incidents, aviation incidents and train crashes.
Data reporting on the incidence of terrorism has recently been complicated by changing definitions and political motivations of the reporting agencies. In spite of the controversies and complexities surrounding the reporting, the number of international terrorist attacks has increased significantly since 2000, following a steady decline during the latter half of the 1990s.5 There were on average 229 international terrorist attacks between 1997 and 2006, with a peak of 395 in 2004. Over that period, an average of 694 persons per year were killed, with a peak of 3184 in 2001 (including 2982 deaths due to the September 11 attacks by Al Qaeda in the USA). This is just a very small fraction of the total number deaths attributed to natural and technological disasters and CEs. The regions documenting the highest number of international terrorist attacks over that period have been the Middle East (51%), Western Europe (13%), South Asia (11%), Africa (6%), and Southeast Asia and Oceania (4%).
Disaster epidemiology globally, including the Australasian region, is being impacted by climate change. Global warming has already been associated with an increase in the frequency and unpredictability of weather-related disasters, such as heatwaves, floods and droughts. There is also evidence of increased intensity of tropical cyclones,6 as has been reflected by recent experience with hurricane Katrina (2005) and cyclone Larry (2006). Rising temperatures have already been implicated in the spread of infectious disease vectors, including malaria-carrying mosquitoes. Other important diseases are also sensitive to changing temperatures and rainfall, including dengue, malnutrition and diarrhoea. The health-related and other impacts of climate change will not be evenly distributed. Disasters associated with global warming are particularly likely to threaten the lives and livelihoods of coastal communities, those living in low-lying islands (e.g. due to rising sea levels), and in arid and high mountain zones.
Socioeconomic impact
Disasters have the potential for major socioeconomic impact, costing the international community billions of dollars annually. In developing countries, years of development work and investment can be devastated by a single disaster. During the 10 years to 2005, disasters caused a global average of approximately US$73.4 billion damage per year. Windstorms were the costliest disaster over the decade, accounting for 43% of disaster-associated costs, led by hurricane Katrina at US$125 billion, which caused 40% of all windstorm damage. Terrorist attacks on major financial centres, such as the World Trade Center in New York have demonstrated the potential for tens of billions of direct economic impact, enormous social consequences and political repercussions for mismanaged disaster response. These figures may be overshadowed by pandemic disease, such as from avian influenza, for which economic cost estimates range to upwards of US$1 trillion.7
Disaster management/emergency management
All agencies (integrated) approach
The basis for the Australian system for managing disasters is a partnership between the Commonwealth, state/territory and local governments, and the community. Under legislation, state and territory governments have the primary responsibility for coordinating disaster-management activities. The major role of the Australian Federal Government is to assist state and territory governments in developing their capacity to deal with disasters, for example by providing training courses and developing manuals of best practice. The Commonwealth also provides physical assistance to a state or territory in the event that a disaster exceeds that state or territory’s response capability. Federal assistance in the area of health would most likely be medical resources provided by the Australian Defence Force (ADF). The ADF also has special expertise in the management of incidents involving chemical and biological agents.
Disaster planning
Planning and responding for international disasters have become more relevant for Australasian health professionals in light of the recent terrorist attacks in Bali (2002 and 2005), the Indian Ocean tsunami (2004) and the earthquake in Pakistan and India (2005). Such planning and response can be advised by the internationally recognized Sphere Minimum Standards in Disaster Response8 and in collaboration with important international agencies, such as the United Nation’s Office for Coordination of Humanitarian Affairs. Sphere specifies standards in six sectors of disaster response: water and sanitation, food security, food aid, nutrition, shelter and health services. These standards are relevant for all disasters and represent an extremely useful reference to guide planning and response for domestic incidents as well.
Disaster response activities
Incident management
Scene assessment and stabilization
The initial scene assessment will be conducted by first responders, such as police or ambulance personnel. It is important for the first medical responder, generally an ambulance officer, to rapidly report findings to the Ambulance Communications Centre. An accurate, timely assessment is critical to initiating an appropriate and effective response. Key information that should be related from the scene includes the nature and magnitude of the disaster, the presence of ongoing hazards, the estimated number of deaths and injuries, the need for further assistance, and the most appropriate routes of access to the scene. In large-scale disasters that affect entire populations, such as cyclones or earthquakes, a rapid health assessment followed by broader epidemiological assessments will be required, including an evaluation of the impact on the health infrastructure, public utilities and shelter.
Medical management
Personnel
Provider roles in disasters continue to evolve. Dedicated disaster medical response teams have been extensively studied.9 These teams form an integral part of national response plans in many developed countries notwithstanding lack of data attesting to any reduction in disaster-associated mortality associated with their deployments.10 Considerations of such teams for Australia are presently under discussion at Commonwealth level.
Casualty-flow plan
Disaster epidemiology has refined the expectations of casualty flow plans. Current epidemiological evidence indicates that 50–80% of people acutely injured in a mass casualty disaster will arrive at the closest medical facilities generally within 90 min after the event.11 Moreover, the vast majority of disaster-affected patients will self-evacuate without benefit of prehospital triage, transport or decontamination. A casualty-flow plan remains crucial to optimize patient care and transportation of those remaining at the scene. A casualty collection area should be established at a site that is close enough to the disaster scene to allow easy access, but far enough away to ensure protection from potential hazards. Patients are assembled and triaged here prior to transfer to a nearby patient treatment post, where they are once again triaged, and basic medical care provided. An ambulance loading point and ambulance holding point also need to be clearly marked so that patient transportation is conducted efficiently, and to ensure that scene convergence and congestion is minimized. Landing zones for helicopters are established away from the incident site for safety reasons, to limit noise and to reduce down-wash from rotor blades. A temporary morgue may need to be established in a nearby area when many fatalities have occurred.
Triage
Different triage systems have emerged in different parts of the world. In British and Australasian health systems, ‘sieve and sort’ triage processes have become the preferred approach through major incident medical management and support (MIMMS) training courses.12 In North America, ‘start and save’ triage processes have become incorporated into the national disaster medical system.13 More recently, a national disaster life support consortium in the USA has promulgated a triage approach based on ‘move, assess, sort and send’.14 These different systems rely on different assessment approaches with different vital sign thresholds to assign triage priority.
Decontamination
Chemical, biological, radiological and nuclear (CBRN) agents have the potential to contaminate individuals, property and the general environment. In practice, industrial accidents represent by far the most common cause of exposure to hazardous materials that may require decontamination. A small number of high-profile chemical-biological terrorist incidents over the past 15 years have also prompted medical as well as lay attention to this potential threat. Regardless of the cause, the principles guiding the process of decontamination remain consistent. Decontamination is the process of removing or neutralizing a hazard from the victim or environment. Detailed management protocols exist for these hazards.15–17 Fundamental principles involve:
Transportation
Efficient and rational transportation of patients to appropriate health facilities is dependent on good communications between hospitals and the incident transport officer. Capabilities of the affected community’s hospitals should be identified and documented in the regional disaster plan. Hospitals will be required to regularly update the incident commander and transport officer of their bed availability status. The closest hospitals are often flooded by ‘walking wounded’ who have made their own way from the scene, and by victims transported by well-meaning civilians. This has the potential of overwhelming local emergency departments, and the transport officer must take this into consideration when determining the appropriate distribution of patients. It is essential that the disaster not be relocated to the nearest hospitals.
Hospitals
Generic hospital plans for disaster management have become widely available from the World Health Organization18 as well as domestic stakeholders.19 Emergency physicians are expected to be familiar with their own hospital disaster plan and have contributed significantly to its development. The plan should address both internal and external disasters.
Mental health
It is easy to overlook the mental health needs of affected individuals during the emergency response, when rescue and life-saving interventions receive top priority. Emergency physicians should be aware of the significant psychological impact of disasters on victims, families and rescue personnel. Psychological support is recommended as first-level assistance to disaster-affected communities and personnel.20 Mental health consequences, such as depression, anxiety states and post-traumatic stress syndrome, are well described following disasters and need to be considered when developing the disaster plan. Crisis counselling may play an important role in the overall medical care provided to patients following a disaster. In addition, rescue personnel may well suffer psychological consequences from their own involvement in the disaster response and should therefore be provided with access to appropriate services, including critical incident debriefing.
Public health issues in disasters
The interface between emergency medicine and public health becomes increasingly important following technological disasters or terrorist events involving biological, chemical or nuclear agents. The terrorist attacks with anthrax in the USA during 2001 and their aftermath demonstrated the vital importance of key public health tools such as disease surveillance and outbreak investigation and control. Following incidents with chemical or radiological agents, public health officials may be required to provide guidance on issues such as evacuation of the public, mass decontamination and the mass distribution of iodine. Emergency physicians should become more familiar with the skills, roles and responsibilities of their public health colleagues, especially as they relate to disaster management and infectious disease control.
Conclusion
Controversies and future directions
1 International Federation of Red Cross and Red Crescent Societies. World Disasters Report. Geneva: International Federation of Red Cross and Red Crescent Societies, 2006;197.
2 Murray V, Clifford J, Seynaeve G, et al. Disaster health education and training: a pilot questionnaire to understand current status. Prehospital and Disaster Medicine. 2006;21(3):156-167.
3 Coghlan B, Brennan RJ, Ngoy P, et al. Mortality in the Democratic Republic of Congo: a nationwide survey. Lancet. 2006;367:44-51.
4 United Nations Office for the Coordination of Humanitarian Affairs. 2007. http://www.reliefweb.int. (accessed 8 October)
5 Memorial Institute for the Prevention of Terrorism. 2007. http://www.tkb.org/chwiz1.jsp. (accessed 13 September)
6 Intergovernmental Panel on Climate Change. Climate Change 2007. The Physical Science Basis. Contribution of Working Group I to the Fourth Assessment Report of the Intergovernmental Panel on Climate Change, 5 February 2007, 2007. Available at http://www.ipcc.ch/SPM2feb07.pdf (accessed 8 October)
7 Brahmbhatt M. Economic Impacts of Avian Influenza Propagation, 2006. Available from URL http://web.worldbank.org/WBSITE/EXTERNAL/NEWS/0,contentMDK:20978927~menuPK:34472~pagePK:34370~piPK:34424~theSitePK:4607,00.html (accessed August)
8 Sphere Project. Humanitarian Charter and Minimum Standards in Disaster Response. Oxford: Sphere Project, 2004.
9 Anton Breil Centre for Public Health and Tropical Medicine, James Cook University. Disaster Medical Assistance Teams: a literature review. Western Australia, Australia: Department of Health, 2006. Available from the Health Protection Group
10 Bradt DA. Site management of health issues in the 2001 World Trade Center disaster. Academic Emergency Medicine. 2003;10:650-660.
11 US Centers for Disease Control and Prevention. Mass trauma casualty predictor, 2007. http://www.cdc.gov/masstrauma/preparedness/predictor.htm. (accessed January)
12 Advanced Life Support Group. Major incident medical management and support – the practical approach. London: BMJ Publishing, 1998.
13 Benson M, Koenig KL, Schultz CH. Disaster Triage: START, then SAVE – a new method of dynamic triage for victims of a catastrophic earthquake. Prehospital Disaster Medicine. 1996;11:117-124.
14 National Disaster Life Support Education Consortium. Basic Disaster Life Support Provider Manual. Available from the NDLSEC. Chicago: American Medical Association, 2003.
15 Agency for Toxic Substances and Disease Registry. CD-ROM available from ATSDR. Hospital emergency departments: a planning guide for the management of contaminated patients. In: Managing Hazardous Materials Incidents, Vol. 2. Atlanta, GA, 2001. revised. September http://www.atsdr.cdc.gov
16 US Army Medical Research Institute of Infectious Diseases. Medical Management of Biological Casualties Handbook, 6th edn, April 2005. Available from http://www.usamriid.army.mil/education/bluebookpdf/USAMRIID%20BlueBook%206th%20Edition%20-%20Sep%202006.pdf (accessed August 2007)
17 US Army Medical Research Institute of Chemical Defense. Medical Management of Chemical Casualties Handbook, 3rd edn, 2000 July. Available from www.gmha.org/bioterrorism/usamricd/Yellow_Book_2000.pdf (accessed August 2007)
18 World Health Organization Western Pacific Region. Field manual for capacity assessment of health facilities in responding to emergencies. WHO Regional Office for the Western Pacific: WHO Western Pacific Regional Publications Office, 2006. Available from the
19 Qureshi K, Gebbie KM, Gebbie EN. 1st edn. Public Health Incident Command System: A Guide for the Management of Emergencies or Other Unusual Incidents within Public Health Agencies, Vol. 1 & 2. October 2005. Available from http://www.ualbanycphp.org/pinata/phics/guide/default.cfm
20 Interagency Standing Committee. IASC Guidelines on Mental Health and Psychosocial Support in Emergency Settings. Geneva: IASC, 2007.
26.4 Triage
Introduction
Origins of triage
The word ‘triage’, arising from the French trier meaning ‘to sort’ has its origins in Latin. It has entered English at least three times: from the 18th century wood industry, the 19th century coffee industry and 20th century emergency medicine. The process understood today as triage was first described by Baron Dominique Jean-Larrey (1766–1842),1 the surgeon to Napoleon, who also developed the ambulance volante, the first field ambulance. This delivered large numbers of injured but salvageable cases to medical units, mandating a more efficient system than treatment in order of military rank. Jean-Larrey’s ‘order of dressing and arrangement’ by urgency was also in keeping with the egalitarian spirit of the French revolution, although there is no evidence that he actually used the word triage. His concept was embraced and refined by military surgeons over the next 150 years, usually with the primary intent of returning soldiers to battle in the most efficient manner.
Civilian triage developments
There was certainly some sorting of patients from the moment ‘casual wards’ opened in 19th century hospitals, but the first systematic description in civilian medicine was by E. Richard Weinerman in Baltimore in 1966.2 Since that time, there has been a huge growth in emergency medicine as a specialty and a number of workers have undertaken formal investigation of triage, particularly in Australasia. The Australasian experience formed the basis of ED triage development in Canada and the UK, whilst some other jurisdictions have developed systems independently.
Process of triage
Triage is an ongoing process that may change in response to alterations in patient status and resource availability, but it is efficient to undertake a formal process once, early in the patient’s encounter, and then review only as necessary. ED triage is normally undertaken by trained nursing staff at the time of arrival, and the assigned urgency is then used to guide treatment order. The overall efficiency and effectiveness of such a system depends not only on the allocated priority but also on the treatment strategy, that is, the way in which the next patient is chosen from the different queues. A more urgent case should wait less time than a less urgent case, but when resources become available to treat the next patient, choice may still be required between a new arrival and a slightly less urgent patient who has already been waiting for some time.
Australasian triage development
The first Australasian description was of the Box Hill Triage Scale by Pink and Brentnall in 1977.3 They used verbal descriptions without time consideration and classified patients into five categories: immediate, urgent, prompt, non-urgent and routine. Fitzgerald modified this scale in 1989,4 to produce the Ipswich Triage Scale. This used five colours to categorize patients according to the question: ‘This patient should under optimal circumstances be seen within….’ The five categories were seconds, minutes, an hour, hours and days. Fitzgerald found his triage scale to have good interobserver reliability on formal testing and to be a practical predictor of ED outcome and length of intensive care stay, but a relatively poor predictor of outcome at hospital discharge.
Jelinek5 investigated the relationship between the Ipswich Triage Scale and resource use in a stratified sample of 2900 presentations. He observed a strong correlation between triage categorization and overall use of resources in the ED and validated possible funding models. He proposed two possible casemix classifications: urgency and disposition groups (UDGs – 12 groups), and urgency-related groups (URGs – 73 groups) based on urgency, disposition and diagnosis. After trimming for outliers, these were found to account for 47% and 58% of the cost variance in large hospitals.
In 1994, the Australasian College for Emergency Medicine formalized the National Triage Scale (NTS),6 derived from the Ipswich Triage Scale. This used colours, names or numerical categories to represent five groups, based on the answer to the question: ‘This patient should wait for medical care no longer than….’ The categories were immediate, 10 min, 30 min, 1 h and 2 h. The definition document also proposed Jelinek’s concept5 of performance indicators based on the proportion of patients whose care fell within the desired time threshold, and audit by means of admission rates and sentinel diagnoses. It influenced treatment strategies by indicating the need to achieve performance indicators in a high proportion of patients in every category (higher in the more urgent) and it clearly established the need for EDs to employ systematic, accountable and audited triage processes.
Over the next few years the NTS was widely accepted and recognized by all Australian State Governments as an appropriate measure of access to emergency care. It was also adopted in the performance indicators promulgated by the Australian Council on Healthcare Standards.7 Research repeated the findings of Fitzgerald and Jelinek with reference to the new five-point scale and investigated many more of the subtleties of triage scale use.
The Australasian triage scale
The ATS8 is the current refinement of the NTS. It has been jointly developed by the Australasian College for Emergency Medicine, emergency nursing organizations and other interested parties. For practical purposes the scale concept itself is unchanged, but the ATS uses numeric classification only, better defines waiting time and includes associated implementation guidelines and educational material, partly derived from work on the NTS in areas such as mental health triage.9
The ATS categorizes patients presenting to EDs in response to the question: ‘This patient should wait for medical assessment and treatment no longer than…’ (Table 26.4.1).
Table 26.4.1 ATS categorization of patients presenting to EDs
ATS category | Treatment acuity (maximum waiting time) |
---|---|
ATS 1 | Immediate |
ATS 2 | 10 min |
ATS 3 | 30 min |
ATS 4 | 60 min |
ATS 5 | 120 min |
Other triage scales
Use beyond waiting time
Triage is based on a brief assessment, and an individual triage categorization can reflect only the probability of certain outcomes. Large populations of triaged patients, however, exhibit predictable patterns. There is a very strong, almost linear relationship between triage category and total rate of admission, transfer, or death, ranging from 80–100% in ATS 1 to 0–20% in ATS 5. This pattern is repeated across hospitals of different size and different patient mix.13 Admission rates by triage category follow the pattern of overall admission rates in relation to age, giving a flattened U-shaped distribution. The inter-rater reliability studies performed using the Ipswich Triage Scale have been repeated using the NTS/ATS, which has been found to be slightly better.14 Further, admission rates by triage category have been shown to be constant over time in individual institutions.15
The NTS/ATS has been extensively studied as a casemix tool. ED outcome (admission/transfer/death versus discharge) accounts for the largest variance in cost, but triage categorization comes a close second, with age third. The mean cost of care for a Category 1 patient is approximately 10 times that of a Category 5 patient. UDGs, described by Jelinek using the Ipswich Triage Scale, have been validated using the NTS by Erwich on 17 819 attendances.16,17 Age has been included to derive urgency, disposition, and age groups (UDAGs – 32 groups), which account for 51% of the cost variance and are not susceptible to different diagnostic approaches.14 These studies may not be valid in the era of overcrowding and access block, because staff costs for admitted patients reflect length of time in the ED, which may now be driven by outside factors. Furthermore, the costs for discharged patients are skewed by increased pressure to keep complex patients out of hospital.
Triage categorization is a very strong predictor of ED outcome and a good predictor of utilization of critical care resources. However, it is a relatively poor predictor of outcome at hospital discharge.18,19 Many patients with chronic or subacute conditions that frequently cause death are triaged to less urgent categories because there is no benefit from earlier treatment within the time scales available in the ED.
Attainment of performance indicators for patients seen within triage thresholds has been shown to be a measure of resource allocation within the ED. A longitudinal comparative study has demonstrated a significant improvement with an increase in ED staff and funding.20
Triage categorization alone is insufficient to direct patients to fast-track services designed for low-complexity patients, but triage staff are appropriate and are able to assess complexity.21
Structure and function of a triage system
Pre-hospital triage
1 Larrey DJ. Mercer JC, editor. Surgical memoirs of the campaigns in Russia, Germany, and France. Carey and Lea, Philadelphia; 1832: Cited in Winslow G. Triage and Justice. University of California Press, 1982.
2 Weinerman ER, Ratner RS, Robbins A. Yale studies in ambulatory care V. Determinants of use of hospital emergency services. American Journal of Public Health Nations Health. 1966;56(7):1037-1056.
3 Pink N. Triage in the accident and emergency department. Australian Nurses Journal. 1977;6(9):35-36.
4 Fitzgerald GJ. Emergency department triage. University of Queensland: Doctor of Medicine Thesis, 1989.
5 Jelinek GA. Casemix classification of patients attending hospital emergency departments in Perth, Western Australia. University of Western Australia: Doctor of Medicine Thesis, 1995.
6 Australasian College for Emergency Medicine. National Triage Scale. Emergency Medicine (Australia). 1994;6(2):145-146.
7 Australian Council on Healthcare Standards. Clinical indicators – a user’s manual. Zetland: NSW: ACHS, 1996.
9 Smart D, Pollard C, Walpole B. Mental health triage in emergency medicine. Australian and New Zealand Journal of Psychiatry. 1999;33:57-66.
10 Beveridge R, Ducharme J, James L, et al. Beaulieu S, Walter S. Reliability of the Canadian Emergency Department Triage and Acuity Scale: interrater agreement. Annals of Emergency Medicine. 1999;34(2):155-159.
11 Manchester Triage Group. London: Emergency triage. Publishing Group, 1997.
12 Wuerz RC, Milne LW, Eitel DR. Reliability and validity of a new five-level triage instrument. Academic Emergency Medicine. 2000;3:236-242.
13 Whitby S, Ieraci S, Johnson D, et al. Analysis of the process of triage: the use and outcome of the National Triage Scale. Liverpool Health Service: Liverpool, NSW, 1997.
14 Jelinek GA, Little M. Inter-rater reliability of the National Triage Scale over 11 500 simulated occasions of triage. Emergency Medicine (Australia). 1996;8:226-230.
15 Richardson DB. No relationship between emergency department activity and triage categorization. Academic Emergency Medicine. 1998;5:141-145.
16 Erwich MA, Bond MJ, Phillips DG. The identification of costs associated with emergency department attendances. Emergency Medicine (Australia). 1997;9:181-187.
17 Erwich MA, Bond MJ, Baggoley CJ. Costings in the emergency department. Report to the Commonwealth Department of Health and Human Services (Australia). 1996.
18 Dent A, Rofe G, Sansom G. Which triage category patients die in hospital after being admitted through emergency departments? A study in one teaching hospital. Emergency Medicine (Australia). 1999;11:68-71.
19 Doherty SR, Hore CT, Curran SW. Inpatient mortality as related to triage category in three New South Wales regional base hospitals. Emergency Medicine (Australia). 2003;15(4):334-340.
20 Rogers IR, Evans L, Jelinek GA. Using clinical indicators in emergency medicine: documenting performance improvements to justify increased resource allocation. Journal of Accident and Emergency Medicine. 1999;16:319-321.
21 Vance J, Sprivulis P. Triage nurses validly and reliably estimate emergency department patient complexity. Emergency Medicine Australasia. 2005;17(4):382-386.
22 Richardson DB, Kelly AM, Baggoley CJ, et al. Variation in triage categorisation: does daily activity make a difference? [abstract]. Academic Emergency Medicine. 1999;6:397-398.
23 Durojaive L, O’Meara M. A study of triage in paediatric patients in Australia. Emergency Medicine (Australia). 2002;14:67-76.
24 Kelly AM, Richardson D. Training for the role of triage in Australasia. Emergency Medicine (Australia). 2001;13:230-232.
26.5 Refugee health
Introduction
Caring for refugees is not a new problem. Since World War II up to 100 000 000 civilians have been forced to flee their homes due to unrest. The major factors that cause people to flee their country, conflict, political repression and persecution, are as old as humanity. In 1573, the term ‘refugee’ was first used for Calvinists fleeing political repression in the Spanish-controlled Netherlands. Refugee numbers are now higher than ever and seem to be relentlessly increasing. The United Nations High Commission for Refugees (UNHCR) is currently responsible for the welfare of some 33 million refugees and other persons of concern (January 2007 figures). These consist mostly of internally displaced persons (refugees inside their country of origin), asylum seekers and recently returned refugees. This equates to about 1:200 persons on the planet. The problem is massive.
Emergency phase
Shelter and site planning
Proper shelter and adequate clothing are essential early priorities. Overcrowding can lead to or worsen disease outbreaks as well as affecting the mental health of refugees. Protection from the elements is also essential for wellbeing, especially in extreme climates. Again well-defined standards for living space and shelter construction exist. Planning the location of the camp is also essential. A good site needs to be large enough and secure and have adequate infrastructure like good road or air access. It should also have access to water supply and be relatively protected from the elements.
Post-emergency phase
Past problems
In the past there have been important problems with the response to a refugee crisis. Often these have their root in poor coordination between the agencies that respond to a particular crisis, which may lead to inappropriate interventions and even frank competition. Often in a dramatic disaster such as an earthquake, which has considerable media coverage, there is a frenzy of intervention as agencies attempt to get their image across to international viewers to assist in fundraising. In the 2001 earthquake in Gujarat province, India, it was estimated that there were as many as 200 different government and non-government agencies in the field. There is no doubt that this has resulted in unnecessary death, most notably in the great lakes region of Africa following the Rwandan genocide.
Innovations in refugee care
Overcoming the problems of lack of planning and coordination has been the major thrust of more recent developments. Importantly, in 1997 it was decided to establish a set of minimum standards and rights to which refugees were entitled. The collaborative project called Sphere involved numerous organizations involved in humanitarian care including Red Cross, MSF and Oxfam. It produced a manual that is available at no cost from the website www.sphere.org. Individual organizations, such as MSF, have several excellent manuals describing in detail the approach to humanitarian emergencies.
Attributes of a refugee doctor
Controversies and future directions
Emergency Relief Items. United Nations Development Program, Vol. 1 & 2., 2000.
Hospitals for War Wounded. International Committee of the Red Cross, 1998. Available www.refiefweb.int This is the entry point into the UN agencies such as OCHA and its various branches. Also publishes situation reports of evolving disasters
www.unhcr.org. Refugee facts, figures and histories.
8 Humanitarian Charter and Minimum Standards in Disaster Response. Available free on website. www.sphereproject.org, 2004.
www.ifrc.org. International Federation of Red Cross and Red Crescent societies.
www.icrc.org. International Committee of the Red Cross site. This is more concerned with war zones.
www.msf.org. The MSF website, which is a very useful resource with several free publications on refugee healthcare.
www.sphere.org, from which you can download the sphere manual.
www.redcross.org.au. The Australian Red Cross site for information on where Australians are currently posted overseas. www.msf.org.au has similar data for MSF.
Médecins Sans Frontières. Refugee health, an approach to emergency situations. McMillan Education Ltd, 1997. This and many other invaluable MSF texts on treatment protocols, basic kits are all available free on the MSF website www.msf.org
26.6 Emergency department observation wards
Introduction
An observation ward (OW) is an essential part of a modern emergency department (ED). OWs are a valuable tool in the safe and efficient management of patients and are rapidly being embraced by EDs worldwide. In a 1989 survey it was found that of 44 EDs in major Australian hospitals 50% had beds designated as an OW. A further 25% expressed an intention to establish one.1 Although there is much local variability, OWs are defined by the following general characteristics:
OW policies and protocols
Admission criteria
Time-limited intensive treatment:
Patients requiring a period of observation before a decision about final disposition is made:
Patients for whom discharge is inappropriate for social or other reasons:
‘Safety net’ for junior staff working out of hours:
Exclusion criteria
Patients who will clearly need >24 h admission:
Patients who require intensive nursing care:
Admission means that proper assessments are not done:
Efficiency of patient care
Audit and feedback
As with any other medical activity auditing admissions to the OW and monitoring adverse events is important. Results can then be fed back through ED doctors as a quality improvement exercise. Firm key performance indicators for OWs have not been established yet but data worth collecting and analysing might include numbers of OW admissions subsequently admitted under an inpatient team (internationally 10–20% is considered acceptable2), patients discharged who re-present within 48 h, and adverse events and outcomes.
Overall impact of observation wards
OWs are generally well accepted by ED staff who easily see the benefits to efficient functioning of the department. The improved efficiency, however, goes beyond the ED and can affect the hospital as a whole. In a recent study performed in a major Australian ED3 it was found that the introduction of an OW had significant effects on the hospital as a whole. Using diagnostic related grouping (DRG) codes for the most frequently admitted OW patient types a comparison on numbers admitted to OW and inpatient wards was done. Total inpatient days for these groups were also analysed. It was found that the introduction of an OW resulted in a decrease of these patients admitted to the inpatient wards associated with an increase in admissions to the OW. Overall the total numbers of patients increased, but, despite this increase, the total number of bed days decreased. Effectively, more patients were treated using fewer days in hospital, a clear indication of increased efficiency throughout the hospital.
Short-stay medicine
Controversies and future directions
1 Brillman J, Mathers-Dunbar L, Graff L, et al. Management of observation units. American College of Emergency Physicians. Annals of Emergency Medicine. 1995;25:823-830.
2 Jelinek GA, Galvin GM. Observation wards in Australian hospitals. Medical Journal of Australia. 1989;151:509-511.
3 Williams A, Jelinek GA, Rogers IR. The effect of establishment of an observation ward on hospital admission profiles. Medical Journal of Australia. 2000;173:411-414.
26.7 Emergency department overcrowding and access block
Theoretical basis of overcrowding
EDs can be considered as overcrowded when treatment is dysfunctional, that is the treatment rate is reduced or the treatment quality suffers. This has been termed the ‘cardiac analogy’ model,1 where ED function is regarded as a starling curve, which increases to a peak but then starts to decrease with overwhelming workload. Some authorities consider that an ED can be purely overcrowded with patients waiting to be seen whilst the treatment function remains optimal, others regard this situation as a ‘surge’ – a subset of disaster medicine, rather than an overcrowding problem.
Definition of overcrowding
The Australasian College for Emergency Medicine (ACEM) defines ED overcrowding2 as the situation where ED function is impeded primarily because the number of patients waiting to be seen, undergoing assessment and treatment, or waiting for departure exceeds either the physical or the staffing capacity of the ED. Access block is quantified as the proportion of admissions to hospital, transfers to other hospitals, and deaths that have a total ED time of greater than 8 h.2
The American College of Emergency Physicians defines crowding3 as occurring when the identified need for emergency services exceeds available resources for patient care in the ED, hospital, or both, a definition deliberately closer in spirit to that of disaster medicine. Most research on the subject, however, is concerned with the balance between daily fluctuations and ED occupancy, rather than the response to mass-casualty surges.
There are multiple scales proposed and used to define overcrowding:4,5 EDWIN,6 NEDOCS,7 READI8 and Work score.9 Validation studies are difficult and many rely on ambulance diversion as an outcome measure, which is only suitable for multi-ED urban centres. The few Australasian studies have not shown them to be clinically useful in real time.10
Causes of overcrowding
The single most important factor affecting ED overcrowding is the availability of inpatient beds.11,12 ED overcrowding is best seen as a marker of whole-of-hospital dysfunction which requires a whole-of-hospital response.13,14 Bed availability depends not only on the number of physical beds but also on the way the bedstock is managed.
Results of overcrowding
Adverse effects of hospital overcrowding have been described since the birth of modern medicine,15 and ED overcrowding had been seen as undesirable since before the recognition of emergency medicine as a specialty.16 In Australasia, access block was recognized as a quality issue from 1998,17 first shown to be associated with decreased ED function in 200018 and defined by the ACEM from 2002.2 Worldwide, properly conducted research started in 2001 and since that time multiple studies in different centres have found an association between overcrowding and reduced access to care, decreased quality measures and lesser outcomes.
Associations between overcrowding and outcomes demonstrated in peer-reviewed studies are shown in the Table 26.7.1 Whilst there is probably some publication bias, there remain no published studies equating overcrowding with improvements in care. The association between overcrowding and poor outcomes is accepted to be causative by most medical authorities.11,12
Strategies to deal with overcrowding
EDs have an obligation to reduce overcrowding and to mitigate its effects. As noted, any reduction in overcrowding will be largely achieved through whole-of-hospital changes. Long time-series suggest that, in the absence of hospital-wide changes, access block tends to continue to increase even after mitigation efforts within the ED.39,40
Increases in the number and seniority of ED staff are associated with improvements in process measures41,42 and are a widely used initial response to overcrowding. Physical rebuilding is used to increase patient care spaces but changes in flow dynamics are highly dependent on the rest of the hospital.43 Analysis of flow and system redesign can allow better use of existing resources.44 Deployment of senior medical staff to assist early in the patient’s journey through ED (at triage) reduces total ED time.45 None of these responses can be used indefinitely if access block keeps increasing.
Discretionary, low-complexity presentations by patients who might reasonably be managed elsewhere, often incorrectly called ‘GP-type’ patients, constitute a significant number but an insignificant workload in most EDs.46,47 Such presentations have a short assessment and treatment time and do not need fixed capacity spaces such as resuscitation rooms, so their contribution to occupancy with patients under treatment is low. However, being of lower triage urgency their contribution to the number waiting at any given time is relatively high.
Telephone advice services have not been shown to reduce ED workload in Australasia48,49 but are highly regarded by the public. Dedicated ED fast-track areas50 address the management of low-complexity patients in an efficient manner and thus tend to improve overall waiting time performance and staff and patient satisfaction. Their contribution to reducing occupancy with patients under treatment, and hence improving ambulance offload, is low.
EDs also have a small but significant role in reducing hospital occupancy. Observation medicine within the ED is a useful adjunct or alternative to formal inpatient admission.51 Multidisciplinary assessment and discharge is effective at reducing representation at least in the elderly.52
Conclusions
Controversies/future directions
1 Richardson SK, Ardagh M, Gee P. Emergency department overcrowding: the Emergency Department Cardiac Analogy Model (EDCAM). Accident and Emergency Nursing. 2005;13:18-23.
2 Australasian College for Emergency Medicine. Policy document standard terminology. Emergency Medicine (Australia). 2002;14:337-340.
3 American College of Emergency Physicians. Crowding. Annals of Emergency Medicine. 2006;47(6):585.
4 Hwang U, Concato J. Care in the Emergency Department: How crowded is overcrowded? Academic Emergency Medicine. 2004;11(10):1097-1101.
5 Jones SS, Allen TL, Flottemesch TJ, et al. An independent evaluation of four quantitative emergency department crowding scales. Academic Emergency Medicine. 2006;13(11):1204-1211.
6 Bernstein SL, Verghese V, Leung W, et al. Development and validation of a new index to measure emergency department crowding. Academic Emergency Medicine. 2003;10(9):938-942.
7 Weiss SJ, Derlet R, Arndahl J, et al. Estimating the degree of emergency department overcrowding in academic medical centers: results of the National ED Overcrowding Study (NEDOCS). Academic Emergency Medicine. 2004;11(1):38-50.
8 Reeder TJ, Burleson DL, Garrison HG. The overcrowded emergency department: a comparison of staff perceptions. Academic Emergency Medicine. 2003;10:1059-1064.
9 Epstein SK, Tian L. Development of an emergency department work score to predict ambulance diversion. Academic Emergency Medicine. 2006;13(4):421-426.
10 Raj K, Baker K, Brierley S, et al. National Emergency Department Overcrowding Study tool is not useful in an Australian emergency department. Emergency Medicine Australasia. 2006;18(3):282-288.
11 Hostetler MA, Mace S, Brown K, et al. Subcommittee on Emergency Department Overcrowding and Children, Section of Pediatric Emergency Medicine, American College of Emergency Physicians. Emergency department overcrowding and children. Pediatric Emergency Care. 2007;23(7):507-515.
12 Trzeciak S, Rivers EP. Emergency department overcrowding in the United States: an emerging threat to patient safety and public health. Emergency Medical Journal. 2003;20(5):402-405.
13 Cameron PA. Hospital overcrowding: a threat to patient safety? Managing access block involves reducing hospital demand and optimising bed capacity. Medical Journal of Australia. 2006;184(5):203-204.
14 Richardson DB. Reducing patient time in the Emergency Department. Medical Journal of Australia. 2003;179(10):516-517.
15 Nightingale F. Notes on Hospitals, 3rd edn. London: Longman, Green, Longman, Roberts and Green, 1863.
16 Shah CP, Carr LM. Triage: a working solution to over crowding in the emergency department. Canadian Medical Association Journal. 1974;110:1039-1043.
17 Baggoley C. President’s message. Emergency Medicine (Australia). 1998;10:169-271.
18 Richardson DB. Quantifying the effects of access block [abstract]. Emergency Medicine (Australia). 2001;13:A10.
19 Fatovich DM, Nagree Y, Sprivulis P. Access block causes emergency department overcrowding and ambulance diversion in Perth, Western Australia. Emergency Medicine Journal. 2005;22(5):351-354.
20 Schull MJ, Morrison LJ, Vermeulen M, et al. Emergency department overcrowding and ambulance transport delays for patients with chest pain. Canadian Medical Association Journal. 2003;168:277-283.
21 Weiss SJ, Ernst AA, Derlet R, et al. Relationship between the National ED Overcrowding Scale and the number of patients who leave without being seen in an academic ED. American Journal of Emergency Medicine. 2005;23(3):288-294.
22 Vieth TL, Rhodes KV. The effect of crowding on access and quality in an academic ED. American Journal of Emergency Medicine. 2006;24(7):787-794.
23 Dunn R. Reduced access block causes shorter emergency department waiting times: an historical control observational study. Emergency Medicine (Australia). 2003;15(3):232-238.
24 Schull MJ, Vermeulen MJ, Stukel TA. The risk of missed diagnosis of acute myocardial infarction associated with emergency department volume. Annals of Emergency Medicine. 2006;48:647-655.
25 Schull MJ, Vermeulen MJ, Slaughter G, et al. Emergency department crowding and thrombolysis delays in acute myocardial infarction. Annals of Emergency Medicine 2004;44(6):577-585. Erratum in Annals of Emergency Medicine. 2005;45(1):84.
26 Fishman PE, Shofer FS, Robey JL, et al. The impact of trauma activations on the care of emergency department patients with potential acute coronary syndromes. Annals of Emergency Medicine. 2006;48(4):347-353.
27 Diercks DB, Roe MT, Chen AY, et al. Prolonged emergency department stays of non-ST-segment-elevation myocardial infarction patients are associated with worse adherence to the American College of Cardiology/American Heart Association guidelines for management and increased adverse events. Annals of Emergency Medicine. 2007;50(5):489-496.
28 Pines JM, Hollander JE, Localio AR, et al. The association between emergency department crowding and hospital performance on antibiotic timing for pneumonia and percutaneous intervention for myocardial infarction. Academic Emergency Medicine. 2006;13(8):873-878.
29 Fee C, Weber EJ, Maak CA, et al. Effect of emergency department crowding on time to antibiotics in patients admitted with community-acquired pneumonia. Annals of Emergency Medicine. 2007;50(5):501-509. 509.e1
30 Pines JM, Localio AR, Hollander JE, et al. The impact of emergency department crowding measures on time to antibiotics for patients with community-acquired pneumonia. Annals of Emergency Medicine. 2007;50:510-516.
31 Hwang U, Richardson LD, Sonuyi TO, et al. The effect of emergency department crowding on the management of pain in older adults with hip fracture. Journal of the American Geriatrics Society. 2006;54:270-275.
32 Pines JM, Hollander JE. Emergency department crowding is associated with poor care for patients with severe pain. Annals of Emergency Medicine. 2008;51(1):1-5. discussion 6-7. Epub 2007 Oct 25
33 Carr BG, Kaye AJ, Wiebe DJ, et al. Emergency department length of stay: a major risk factor for pneumonia in intubated blunt trauma patients. Journal of Trauma. 2007;63(1):9-12.
34 Richardson DB. The access block effect: relationship between delay to reaching an inpatient bed and inpatient length of stay. Medical Journal of Australia. 2002;177:492-495.
35 Liew D, Liew D, Kennedy MP. Emergency department length of stay independently predicts excess inpatient length of stay. Medical Journal Australia. 2003;179:524-526.
36 Chalfin DB, Trzeciak S, Likourezos A, et al. DELAY-ED study group. Impact of delayed transfer of critically ill patients from the emergency department to the intensive care unit. Critical Care Medicine. 2007;35(6):1477-1483.
37 Richardson DB. Increase in patient mortality at 10 days associated with emergency department overcrowding. Medical Journal of Australia. 2006;184(5):213-216.
38 Sprivulis PC, Da Silva JA, Jacobs IG, et al. The association between hospital overcrowding and mortality among patients admitted via Western Australian emergency departments. Medical Journal of Australia. 2006;184(5):208-212.
39 Richardson DB. Responses to access block in Australia: Australian Capital Territory. Medical Journal of Australia. 2003;178(3):103-104.
40 Fatovich DM. Responses to access block in Australia: Royal Perth Hospital. Medical Journal of Australia. 2003;178(3):108-109.
41 Rogers IR, Evans L, Jelinek GA, et al. Using Clinical indicators in emergency medicine: documenting performance improvements to justify increased resource allocation. Journal of Accidents and Emergency Medicine. 1999;16:319-321.
42 Cardin S, Afilalo M, Lang E, et al. Intervention to decrease emergency department crowding: does it have an effect on return visits and hospital readmissions? Annals of Emergency Medicine. 2003;41:173-185.
43 Han JH, Zhou C, France DJ, et al. The effect of emergency department expansion on emergency department overcrowding. Academic Emergency Medicine. 2007;14:338-343.
44 King DL, Ben-Tovim DI, Bassham J. Redesigning emergency department patient flows: application of Lean Thinking to health care. Emergency Medicine Australasia. 2006;18:391-397.
45 Holroyd BR, Bullard MJ, Latoszek K, et al. Impact of a triage liaison physician on emergency department overcrowding and throughput: a randomized controlled trial. Academic Emergency Medicine. 2007;14:702-708.
46 Schull MJ, Kiss A, Szalai JP. The effect of low-complexity patients on emergency department waiting times. Annals of Emergency Medicine. 2007;49:257-264.
47 Sprivulis P, Grainger S, Nagree Y. Ambulance diversion is not associated with low acuity patients attending Perth metropolitan emergency departments. Emergency Medicine Australasia. 2005;17(1):11-15.
48 Graber DJ, Ardagh MW, O’Donovan P, et al. A telephone advice line does not decrease the number of presentations to Christchurch Emergency Department, but does decrease the number of phone callers seeking advice. New Zealand Medical Journal. 2003;116(1177):U495.
49 Sprivulis P, Carey M, Rouse I. Compliance with advice and appropriateness of emergency presentation following contact with the HealthDirect telephone triage service. Emergency Medicine Australasia. 2004;16(1):35-40.
50 O’Brien D, Williams A, Blondell K. Impact of streaming ‘fast track’ emergency department patients. Australian Health Review. 2006;30(4):525-532.
51 Williams AG, Jelinek GA, Rogers IR, et al. The effect on hospital admission profiles of establishing an emergency department observation ward. Medical Journal of Australia. 2000;173(8):411-414.
52 Caplan GA, Williams AJ, Daly B. A randomized, controlled trial of comprehensive geriatric assessment and multidisciplinary intervention after discharge of elderly from the emergency department-the DEED II study. Journal of American Geriatrics Society. 2004;52(9):1417-1423.
26.8 Patient safety
Introduction
Patient safety, or the freedom from accidental injury due to medical care or from medical error, is increasingly being recognized as a critical consideration in the delivery of acute and emergency healthcare.1 Several OECD countries have examined the proportion of acute care admissions during which an adverse event (an unexpected medical problem that happens during treatment with a drug or other therapy) is identifiable using a standard medical chart review. They typically report that 1 in 10 admitted patients experiences an adverse event, of which half are considered preventable with the current state of medical knowledge (i.e. are due to medical error).1 Typically, a third of adverse events lead to moderate, or greater, disability or death.1 An important consideration for the emergency care of admitted patients is that the day of greatest risk of an adverse event is usually the first day of admission to hospital. This is when knowledge of the patient’s clinical condition is often incomplete, the clinical condition is least stable and when most patients experience the greatest number of procedures and interventions.2
Specific emergency department factors that may compromise patient safety
Safe patient care is challenged by several specific emergency department (ED) factors that include:
Common safety problems encountered in emergency departments
The factors described above interact to create a wide range of risks to patients needing emergency care.4 Some of the errors observed in the emergency setting include:
Improving safety in the emergency department
Specific actions to improve patient safety should be undertaken in the context of a comprehensive organizational framework for clinical governance and quality improvement.1 The development of a programme of safety improvement for an ED should be undertaken methodically, in accordance with existing Australasian and international standards that usually encompass the following process elements:
Conclusion
Patients seeking emergency care are at significant risk of harm, in part due to their clinical situation and in part due to the challenges of delivery of emergency care itself. Improving patient safety in the ED requires a systematic approach to risk identification, risk analysis and evaluation and the implementation of safer processes of care. Monitoring is an essential component of patient safety improvement. An open, communicative culture that promotes reporting and minimizes blame supports patient safety improvement.
1 Botwinick L, Bisognano M, Haraden C. Leadership Guide to Patient Safety. Cambridge, MA: Institute for Healthcare Improvement, 2006.
2 Weissman J, Rothschild J, Bendavid E, et al. Hospital workload and adverse events. Medical Care. 2007;45:448-455.
3 Feddock CA, Hoellein AR, Wilson JF, et al. Do pressure and fatigue influence resident job performance? Medical Teach. 2007;29(5):495-497.
4 Croskerry P, Sinclair D. Emergency medicine: a practice prone to error? Canadian Journal of Emergency Medicine. 2001;3(4):271-276.
5 Brown AF. Do we realize when we do not know? Recognizing uncertainty in clinical medicine. Emergency Medicine of Australasia. 2005;17(5–6):413-415.
6 Brixey JJ, Tang Z, Robinson DJ, et al. Interruptions in a level one trauma center: a case study. International Journal of Medical Informatics. 2007.
7 Bomba DT, Prakash R. A description of handover processes in an Australian public hospital. Australia Health Review. 2005;29(1):68-79.
8 Sprivulis PC, Da Silva JA, Jacobs IG, et al. The association between hospital overcrowding and mortality among patients admitted via Western Australian emergency departments. Medical Journal of Australia. 2006;184(5):208-212.
26.9 The medical emergency team
Introduction and definitions
Medical emergency teams (METs) are composed of doctors and nurses that review acutely unwell hospital ward patients in an attempt to reduce cardiac arrests and other serious adverse events (SAEs). The team should have a number of competencies, including abilities in the following areas:1
The term ‘rapid response system’ (RRS) has been proposed to represent an entire system that provides both an ‘afferent’ component to identify patient deterioration and an ‘efferent’ component to assess and treat the patient. The most common efferent component is the MET. Other types of review team include the rapid response team (RRT) and critical care outreach team (CCO), which differ in their staff composition, skill set and mechanism of activation.1 The remainder of this chapter will focus on the MET, which is the predominant team used in hospitals in Australia and New Zealand.
Additional components of the RRS include quality improvement and clinical governance arms, which permit audit and evaluation of SAEs and implementation of hospital-wide strategies to prevent their recurrence.1
Epidemiology and principles underlying the MET
SAEs are common in hospitalized patients
Studies in Australia,2 New Zealand,3,4 England,5 and Canada6 have assessed the incidence of SAEs in hospitalized patients. These studies defined an SAE as ‘unintended injury or complication resulting from medical management rather than the underlying disease process’. They reported an incidence of SAEs ranging between 7.5% and 16.6% and suggested that 36.9–51% were preventable.
A recent single-centre study in an Australian hospital7 found that 16.9% of 1125 patients undergoing major surgery suffered at least one of 11 predefined SAEs (which included myocardial infarction, stroke, arrest and respiratory failure).
SAEs are preceded by signs of clinical instability
At least four studies8–11 have demonstrated that patients suffering SAEs develop new complaints, deterioration of commonly measured vital signs or derangement in laboratory investigations in up to 84% of cases prior to the event. It is for this reason that common triggers for MET activation are based on derangements in vital signs. More importantly, three studies12–14 have confirmed that patients who develop vital signs that satisfy MET criteria are at increased risk of death.
Deterioration of the MET patient is typically gradual
Unexpected out-of-hospital cardiac arrest is usually sudden and due to cardiac arrhythmias, pulmonary embolism or major vascular catastrophe. In contrast, progression to in-hospital cardiac arrests and other SAEs is typically gradual.8 This allows sufficient time for intervention and, potentially, prevention of the event.
Early intervention improves outcome
One of the tenets underlying the MET principle is that early intervention in the course of critical illness is associated with improved outcome. This observation has been made in patients suffering trauma,15,16 myocardial infarction17 and in resuscitation of patients presenting to the emergency department (ED) with sepsis.18
Skilled staff already exist in the hospital
METs are usually composed of critical care staff with skills in advanced airway management, insertion of invasive vascular lines, and with knowledge of therapies commonly used in acute care medicine (Table 26.9.1). Staff need to be available 24 h per day, 7 days a week to manage acutely unwell patients anywhere in the hospital.19
Staff member | Roles |
---|---|
Intensive care registrar |
How MET services and RRS work
Different roles of the MET
The MET was originally described in 1995 when Lee and coworkers reported the introduction of a MET service into Liverpool Hospital in Sydney, Australia.19 The MET superseded the existing cardiac arrest team and was modelled on rapid detection and correction of abnormal vital signs indicative of trauma teams. In this model, the MET is merely an expansion of the existing cardiac arrest team and reviews all medical emergencies including arrests.
In other hospitals20,21 two separate RRTs operate: a cardiac arrest team to review patients who have suffered cardiorespiratory arrest and a MET that reviews all medical emergencies other than cardiac arrest.
Activation of the MET – the afferent ARM
The MET service is activated when one or more predefined criteria are reached. Typical criteria involve derangement of commonly measured vital signs (Table 26.9.2). Other criteria include conditions such as uncontrolled seizures or chest pain. Finally, some hospital MET criteria contain a ‘staff member worried’ criterion to permit activation of the MET service for any possible medical emergency.
System | Criteria |
---|---|
Airway | Stridor |
Threatened airway | |
Breathing | Acute change in RR <8 or >30 bpm |
Acute change in saturation <90% despite oxygen | |
Difficulty breathing | |
Noisy breathing | |
Circulation | Acute change in heart rate <40 or >130 bpm |
Acute change in systolic BP <90 mmHg | |
Uncontrolled chest pain | |
Neurology | Acute change in conscious state |
Agitation or delirium | |
Other | Staff member is worried about the patient |
Acute change in UO to <50 mL in 4 h |
RR, respiratory rate; BP, blood pressure; UO, urine output.
Composition of the MET – the effector ARM
The precise composition of each MET varies between hospitals. Each member will have a predefined role (Table 26.9.1), and simulation or mock sessions may be held as part of their training.22 The teams typically bring their own equipment to the MET call, either on a trolley or in a carry bag, which includes equipment needed for endotracheal intubation, invasive vascular access and medicines and fluids used in advanced resuscitation.
Clinical features of MET patients
Characteristics of triggers leading to MET activation
A number of studies13,21,23 have reported the relative frequency of MET call criteria leading to MET calls (Table 26.9.3). Variations between hospitals are likely to represent differences in the limits of the criteria as well as differences in local case mix. Hypoxaemia, hypotension and altered conscious state were the commonest causes of MET calls in these studies.
Medical conditions leading to MET calls
The clinical cause of MET calls has also been assessed in a number of studies, and the concept of ‘MET syndromes’ (e.g. the ‘hypoxic MET syndrome’) has recently been raised.23,24 Again, variations in case mix and MET calling criteria are likely to account for these differences. In the original description of the MET, Lee and coworkers reported that acute respiratory failure, status epilepticus, coma and pulmonary oedema were the most common causes of MET calls.19 In a district general hospital, Daly and coworkers reported that chest pain, respiratory distress, seizures and cardiopulmonary arrest caused most MET calls.25 Finally, a study of 400 MET calls at the Austin hospital23 revealed that infections, pulmonary oedema and arrhythmias caused 53% of all MET calls.
Management of MET call patients
One of the most underinvestigated aspects of the MET system is the details of the management undertaken by the staff during a MET call. De Vita and coworkers have recently reported that use of a detailed curriculum and a computerized human patient simulator resulted in increased task completion rate and improved survival of the simulated patient.22
An analysis of the MET syndromes associated with 400 MET calls and an approach to their management has been reported.23 The ‘A to G’ approach described can be used to manage any possible medical emergency and can be used in the management of specific MET syndromes (Table 26.9.4).
Table 26.9.4 A to G approach to management of a hypoxic MET call
Step of A to G approach | Approach for hypoxic MET call |
---|---|
Ask |
MET, medical emergency team; ECG, electrocardiogram; CCF, congestive cardiac failure; COAD, chronic obstructive airways disease.
Other issues – the MET and the ED
MET services involving the ED
In the original description of the MET system, the ED was one of the hospital areas serviced by the MET.19 This approach involves review of ED patients by intensive care unit (ICU) staff and is most appropriate during periods when there are limited senior emergency medical staff, particularly out of hours. In contrast, Daly and coworkers described a MET model for a district general hospital in which staff from the ED formed the core of the MET and reviewed patients in the general wards when the MET service was activated.25
The MET philosophy in the ED
The MET involves a coordinated multidisciplinary approach to the management of acute deterioration of hospital ward patients.1 As outline above (Table 26.9.1), each member has a designated role which is coordinated by the team leader. This is similar to the team-based approach to trauma management seen in most EDs and trauma centres (Table 26.9.5).
Table 26.9.5 Similarities and differences between MET services and trauma teams
Variable | Trauma team | MET service |
---|---|---|
Location of patient | Emergency department or trauma centre | Hospital ward |
Team leader | Typically emergency department doctor | Typically intensive care unit registrar |
Patient profile | Young with few comorbidities | Elderly with multiple comorbidities |
Presenting problem | Trauma | Hypoxia, hypotension and tachycardia |
Need for early intervention | Concept of ‘golden hour’ | Shown for sepsis and myocardial ischemia |
The MET system principle is equally applicable in the ED as it is on the hospital ward. For example, a multidisciplinary programme was shown to reduce the hospital mortality rate of patients presenting to a community hospital with non-traumatic shock.26
Controversies
The MET service and deskilling of ward staff
The increasing use of MET services to manage acutely unwell hospital ward patients has the potential to deskill ward nursing and medical staff.27 However, in a survey conducted at a hospital with a well-established MET service, most of the nurses questioned stated that the MET actually taught them how to better manage sick ward patients.28
Improved outcome is demonstrated only in single-centre studies
Reduction in cardiac arrests, unplanned ICU admission and other SAEs following introduction of MET services and RRSs has been shown in a number of single-centre studies.20,21,29–31 A cluster randomized trial of 23 Australian hospitals was recently reported in which 12 hospitals introduced a MET system and 11 continued with usual care.32 The study did not demonstrate that introduction of a MET service reduced the incidence of cardiac arrests, unplanned ICU admissions or unexpected deaths. While this finding may suggest that MET services do not improve the outcome of acutely unwell ward patients, the negative result is at least in part due to other factors. First, the education period preceding introduction of the MET was brief (4 months), and the subsequent call rate was only 8.3 calls/1000 admissions.32 At the Austin hospital, a 1-year education period resulted in a progressive increase in the use of the MET to a call rate of >40 calls/1000 admissions.33
Most importantly, only 30% of the patients admitted to the ICU who had MET criteria actually received a MET call.32 Combined, these findings suggest that the negative result of the MERIT study is at least in part due to a failure of MET use as opposed to a failure of the process of MET review.
Other controversies
A number of other problems of the MET service have been proposed27,34 including inappropriate patient management because the MET is unfamiliar with the patient, diversion of attention away from adequate ward staffing and development of other strategies that might benefit acutely ill ward patients, diversion of critical care staff from their usual duties and conflict between MET staff and the ward staff caring for the patient.
Likely future developments
Despite the absence of level I evidence of the effectiveness of MET services, RRSs have been introduced into thousands of hospitals worldwide. RRSs are a key component of the Institute of Health Improvement’s 100k campaign, which aims to save 100 000 lives across American hospitals.35 For theses reasons, it is unlikely that further randomized trails will be conducted to assess the effectiveness of METs in improving the outcome of acutely unwell hospitalized patients.
1 Devita MA, Bellomo R, Hillman K, et al. Findings of the first consensus conference on medical emergency teams. Critical Care Medicine. 2006;34(9):2463-2478.
2 Wilson RM, Runciman WB, Gibberd RW, et al. The quality in Australian health care study. Medical Journal of Australia. 1995;163(9):458-471.
3 Davis P, Lay-Yee R, Briant R, et al. Adverse events in New Zealand public hospitals I: occurrence and impact. New Zealand Medical Journal. 2002;115(1167):U271.
4 Davis P, Lay-Yee R, Briant R, et al. Adverse events in New Zealand public hospitals II: preventability and clinical context. New Zealand Medical Journal. 2003;116(1183):U624.
5 Vincent C, Neale G, Woloshynowych M. Adverse events in British hospitals: preliminary retrospective record review. British Medical Journal. 2001;322(7285):517-519.
6 Baker GR, Norton PG, Flintoft V, et al. The Canadian Adverse Events Study: the incidence of adverse events among hospital patients in Canada. Canadian Medical Association Journal. 2004;170(11):1678-1686.
7 Bellomo R, Goldsmith D, Russell S, et al. Postoperative serious adverse events in a teaching hospital: a prospective study. Medical Journal of Australia. 2002;176(5):216-218.
8 Buist MD, Jarmolowski E, Burton PR, et al. Recognising clinical instability in hospital patients before cardiac arrest or unplanned admission to intensive care. A pilot study in a tertiary-care hospital. Medical Journal of Australia. 1999;171(1):22-25.
9 Hodgetts TJ, Kenward G, Vlackonikolis I, et al. Incidence, location and reasons for avoidable in-hospital cardiac arrest in a district general hospital. Resuscitation. 2002;54(2):115-123.
10 Nurmi J, Harjola VP, Nolan J, et al. Observations and warning signs prior to cardiac arrest. Should a medical emergency team intervene earlier. Acta Anaesthesiologica Scandinavica. 2005;49(5):702-706.
11 Schein RM, Hazday N, Pena M, et al. Clinical antecedents to in-hospital cardiopulmonary arrest. Chest. 1990;98(6):1388-1392.
12 Bell MB, Konrad D, Granath F, et al. Prevalence and sensitivity of MET-criteria in a Scandinavian University Hospital. Resuscitation. 2006;70(1):66-73.
13 Buist M, Bernard S, Nguyen TV. Association between clinically abnormal observations and subsequent in-hospital mortality: a prospective study. Resuscitation. 2004;62(2):137-141.
14 Goldhill DR, White SA, Sumner A. Physiological values and procedures in the 24 h before ICU admission from the ward. Anaesthesia. 1999;54(6):529-534.
15 Hedges J, Adams A, Gunnels M. ATLS practices and survival at rural level trauma hospitals, 19951999. Prehospital Emergency Care. 2002;6:299-305.
16 Nardi G, Riccioni L, Cerchiari E, et al. Impact of an integrated treatment approach to the severely injured patients (ISS > 16) on hospital mortality and quality of care. Minerva Anesthesiology. 2002;68:25-35.
17 Fresco C, Carinci F, Maggioni AP, et al. Very early assessment of risk for in-hospital death among 11,483 patients with acute myocardial infarction. GISSI investigators. American Heart Journal. 1999;138(6 Pt 1):1058-1064.
18 Rivers E, Nguyen B, Havstad S, et al. Early goal-directed therapy in the treatment of severe sepsis and septic shock. New England Journal Medicine. 2001;345(19):1368-1377.
19 Lee A, Bishop G, Hillman KM. The medical emergency team. Anaesthesia and Intensive Care. 1995;23(2):183-186.
20 Bellomo R, Goldsmith D, Uchino S, et al. Prospective controlled trial of effect of medical emergency team on postoperative morbidity and mortality rates. Critical Care Medicine. 2004;32(4):916-921.
21 Bellomo R, Goldsmith D, Uchino S, et al. A prospective before-and-after trial of a medical emergency team. Medical Journal of Australia. 2003;179(6):283-287.
22 De Vita M, Schaefer J, Lutz J, et al. Improving medical emergency team (MET) performance using a novel curriculum and a computerized human simulator. Quality and Safe Health Care. 2005;14:326-331.
23 Jones D, Duke G, Green J, et al. Medical emergency team syndromes and an approach to their management. Critical Care. 2006;10(1):R30.
24 DeVita M. Medical emergency teams: deciphering clues to crises in hospitals. Critical Care. 2005;9(4):325-326.
25 Daly FF, Sidney KL, Fatovich DM. The medical emergency team (MET): a model for the district general hospital. Australian New Zealand Journal of Medicine. 1998;28(6):795-798.
26 Sebat F, Johnson D, Musthafa A, et al. A multidisciplinary community hospital program for early and rapid resuscitation of shock in non-trauma patients. Chest. 2005;127:1729-1743.
27 Brown D, Bellomo R. Are medical emergency teams worth the cost. In: DeVita MA, Hillman K, Bellomo R, editors. Medical emergency teams: a guide to implementation and outcome measurement. New York: Springer, 2006.
28 Jones D, Baldwin I, McIntryre T, et al. Nurses’ attitudes to a medical emergency team service in a teaching hospital. Quality & Safety in Health Care. 2006;15:427-432.
29 Bristow PJ, Hillman KM, Chey T, et al. Rates of in-hospital arrests, deaths and intensive care admissions: the effect of a medical emergency team. Medical Journal of Australia. 2000;173(5):236-240.
30 Buist MD, Moore GE, Bernard SA, et al. Effects of a medical emergency team on reduction of incidence of and mortality from unexpected cardiac arrests in hospital: preliminary study. British Medical Journal. 2002;324(7334):387-390.
31 DeVita MA, Braithwaite RS, Mahidhara R, et al. Use of medical emergency team responses to reduce hospital cardiopulmonary arrests. Quality and Safe Health Care. 2004;13(4):251-254.
32 Hillman K, Chen J, Cretikos M, et al. Introduction of the medical emergency team (MET) system: a cluster-randomised controlled trial. Lancet. 2005;365(9477):2091-2097.
33 Jones D, Bates S, Warrillow S, et al. Effect of an education programme on the utilization of a medical emergency team in a teaching hospital. Internal Medicine Journal. 2006;36(4):231-236.
34 Joyce C, McArthur C. Rapid response systems: have we MET the need? Critical Care Resuscitation. 2007;9:127-128.
35 Overview of the 100,000 Lives Campaign [cited 2007 16th January]. Available from http://www.ihi.org/IHI/Programs/Campaign/100kCampaignOverviewArchive.htm, 2007. (cited 16 January