CHAPTER 149
Pulmonary Rehabilitation for Patients with Lung Disease
Definition
Pulmonary rehabilitation (PR) is a comprehensive intervention for patients who remain symptomatic with chronic respiratory disease despite standard medical treatment. Chronic diseases of the lung can be manifested in many forms. Chronic obstructive pulmonary disease (COPD) is among the most common, with an estimated prevalence of at least 8% in the United States. Idiopathic pulmonary fibrosis (IPF) is less common, although the incidence increases with older age, with patients typically presenting after the age of 60 years. PR programs are designed to optimize functional status and to reduce symptoms. A multidisciplinary health care team evaluates each patient’s unique needs, with active collaboration among the patient, family members, and health care providers. Treatment programs are then devised and consist of education, physician-prescribed exercise training, nutritional and psychological counseling, and outcomes assessment. Each program includes a spectrum of intervention strategies that address both the primary condition and the secondary impairments and conditions associated with the respiratory disease, such as peripheral muscle dysfunction, anxiety, and depression. The American Thoracic Society and the European Respiratory Society, in a joint consensus statement, have endorsed the use of PR in the management of chronic respiratory disease regardless of cause [1]. Each prescription is individually specific for PR candidates with the conditions listed in Table 149.1 who have limited exercise tolerance despite standard medical treatment. This includes frequently hospitalized patients and patients undergoing lung volume reduction surgery or lung transplantation, who require PR both before and after surgery.
Table 149.1
Conditions Associated with Inadequate Oxygen Supply
Airway Disease | Interstitial Lung Disease* |
COPD, emphysema | Idiopathic |
COPD, chronic bronchitis | Environmental |
Chronic asthma | Occupational |
Cystic fibrosis | Granulomatous diseases |
Bronchiectasis† | Collagen vascular diseases |
Constrictive bronchiolitis | Drug-induced, radiation |
Hypersensitivity pneumonitis† | Inherited |
COPD, chronic obstructive pulmonary disease.
* Interstitial diseases are categorized by causes.
† Presents with mixed obstruction and restriction.
Symptoms
Symptoms of patients with chronic respiratory diseases such as COPD, due to persistent airflow limitation, and IPF, a condition associated with progressive scarring of the lungs (Fig. 149.1), include dyspnea, exercise intolerance, cough, and airway congestion. Initially, symptoms may be attributed to other conditions or age itself [2,3]. Whereas COPD patients are relieved by expectorating sputum, IPF patients typically are not. Both COPD and IPF patients may also have chest pain, orthopnea, sleep disordered breathing, poor endurance, anxiety, depression, and difficulty with concentration [3–5]. Inhalation of cigarette smoke is a risk factor for both conditions [3,6]. Disease progression is variable. Fatigue and any complicating conditions can further exacerbate restrictions in activities of daily living (ADLs) and quality of life [7,8]. Late-stage chronic lung disease patients tend to be home bound.
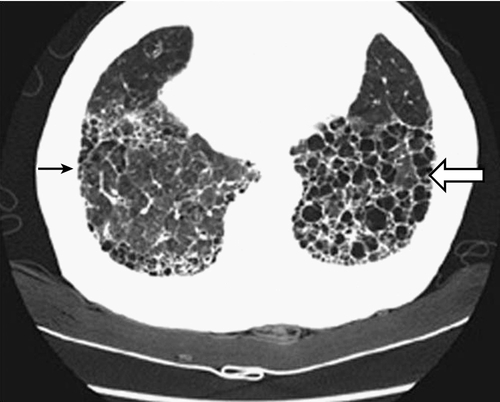
Physical Examination
Abnormal physical findings are not usually detected early on. Auscultation in COPD may eventually reveal wheezing, hyperresonant lung sounds, prolonged expiratory phase, and rales. Chest percussion may reveal hyperresonance and hyperinflation. The chest may be barrel shaped, which is consistent with hyperinflation. Auxiliary respiratory muscle use and pursed-lip breathing are common with end-stage disease.
The most common finding in patients with IPF is inspiratory “Velcro-like” crackles heard on lung auscultation, mostly in the lower lung fields. Clubbing is found in up to 50% of patients. In advanced IPF, a loud pulmonic second heart sound and peripheral edema may be found, consistent with pulmonary hypertension or right-sided heart failure. Associated signs of arthritis and proximal muscle weakness are uncommon and may suggest interstitial lung disease occurring secondary to a collagen vascular disease.
Functional Limitations
Exercise tolerance and the ability to perform ADLs may be diminished by respiratory and skeletal muscle impairment including all limbs and the trunk [9–11]. Aerobic capacity is typically reduced with early lactate accumulation and muscle fatigue [9]. With diminished muscle strength, diminished mobility, and ADL restriction, patients become further deconditioned and dependent on others, leading to decreased social interaction, increased anxiety and depression, and being home bound [3,8]. In one study, 41% of COPD patients left the house less than once per month or never in their last year of life [12]. A cycle of diminished mobility leads to further deconditioning and, in turn, worsening exertional tolerance.
Diagnostic Studies
COPD and IPF are suspected for anyone with dyspnea, cough, or exercise intolerance. For IPF, a complete medical history with appropriate serologic tests is warranted to exclude secondary causes of pulmonary fibrosis.
Spirometry is most valuable for diagnosis of COPD and IPF. Spirometry provides quantifiable and reproducible parameters of airflow obstruction and lung restriction. The Global Initiative for Chronic Obstructive Lung Disease has established that a postbronchodilator forced expiratory volume in 1 second (FEV1) to forced vital capacity (FVC) ratio (FEV1/FVC) of less than 70% is required for the diagnosis of COPD to be made [3]. COPD severity is stratified by symptoms, postbronchodilator FEV1 values, and frequency of exacerbations [3]. COPD patients demonstrate an increased total lung capacity, which is consistent with hyperinflation, and an increased residual volume/total lung capacity ratio, which suggests air trapping. IPF patients, in contrast, exhibit severe restrictive physiology with reduced FEV1 and FVC but a normal or increased FEV1/FVC ratio and reduced total lung capacity.
Patients with emphysema or IPF have decreased lung diffusion capacity for carbon monoxide consistent with loss of effective gas exchange. Oxyhemoglobin saturation and arterial blood gas values are usually normal at rest until the later disease stages. With exercise, hypoxemia and oxygen desaturation commonly occur.
Chest radiography in patients with advanced COPD commonly reveals evidence of hyperinflation. Other findings may include bullae and increased basilar lung markings. Computed tomography (CT) scan of the chest is not routinely used to diagnose COPD but may show evidence of emphysema and/or bullae.
Bilateral, symmetric reticular opacities, with lower lung field predominance, are usually seen in IPF. High-resolution CT (HRCT) scanning can demonstrate a reticular abnormality with a subpleural, basal predominance; honeycombing with or without bronchiectasis; and absence of findings suggestive of an alternative diagnosis, such as ground-glass opacities, nodules, or cysts [6]. When a patient presents with progressive dyspnea and dry cough without known cause and is found on HRCT to show these “typical” findings, surgical biopsy is not necessary to make the diagnosis. In most other cases, histopathologic correlation is warranted along with pulmonary function testing.
Other diagnostic studies can include testing for α1-antitrypsin deficiency for COPD, especially if there is a family history of it. Pulse oximetry allows demonstration of worsening oxygenation with activity. Reduced oxyhemoglobin saturation is an indication for supplemental oxygen therapy. Last, echocardiography is performed when right-sided heart failure is suspected.
Treatment
Management of COPD and IPF includes a comprehensive evaluation for contributing conditions as well as pharmacologic and nonpharmacologic approaches and rehabilitation as noted in Table 149.2. Pharmacologic treatment for COPD is considered in Table 149.3; it is used to reduce dyspnea and frequency of exacerbations and to improve health-related quality of life. For treatment of COPD, short-acting β-agonists and short-acting anticholinergics are equipotent in reducing dyspnea and improving exertional tolerance. In patients with more advanced disease, long-acting bronchodilators are indicated in addition to short-acting bronchodilators [3]. Inhaled corticosteroids are indicated for severe disease or repeated exacerbations despite use of long-acting bronchodilators [3]. Systemic steroids should not be prescribed for stable COPD patients because of an unfavorable risk-benefit ratio. There is insufficient evidence to support the use of any specific pharmacologic therapy for IPF [6].
Nonpharmacologic treatments include airway secretion mobilization, vaccinations (influenza and pneumococcal pneumonia), and education on smoking cessation interventions and the importance of medical regimen adherence and inhaler use techniques. Smoking cessation reduces chronic phlegm production and decreases the rate of loss of FEV1 compared with continued smokers. Training for proper administration of nebulizers and inhalers promotes optimal medication deposition and efficacy. Thick respiratory secretions can augment dyspnea. N-Acetylcysteine is the only mucolytic readily available in clinical practice in the United States, but there is inconclusive evidence for its efficacy [13].
Patients with advanced COPD have a prevalence of osteoporosis of 36% to 60% and should be treated for osteoporosis [3]. Vertebral fractures have been found in 29% of patients [14]. Immobility as well as smoking, vitamin D deficiency, and the use of glucocorticoids can contribute to osteoporosis.
Long-term oxygen therapy is indicated for COPD patients with hypoxemia, demonstrated by arterial blood gas analysis (PO2 < 55 mm Hg) or by pulse oximetry (oxygen saturation < 88%). Long-term oxygen therapy has been shown to increase endurance and to improve survival [15] as well as to improve sleep and cognitive performance [16]. Data for the use of long-term oxygen therapy in patients with IPF are lacking. Extrapolating from data of COPD patients, supplemental oxygen is recommended for clinically significant resting hypoxemia (SpO2 < 88%).
Rehabilitation
Goals and Objectives
The goals of a PR program are to reverse deconditioning and to optimize the individual’s independence and functioning. A PR service aims to alleviate symptoms, to increase physical and social participation, to maximize independent functioning, and to enhance quality of life, all in the framework of reducing health care costs [1]. Specific objectives to improve exercise tolerance, strength, and flexibility as well as coping strategies and stress management require periodic reassessment. Patients, their families, and a multidisciplinary PR team work together to achieve the objectives.
Staffing
Specific PR team staffing varies and depends on resources and availability. All programs have a physician medical director who is responsible for initial patient assessment to determine candidacy for PR, to identify comorbidities, and to ensure an optimal medical regimen [17]. The physician must be accessible for emergencies when inpatient or outpatient services are being furnished.
A well-structured PR team also consists of therapists (physical, occupational, respiratory), a nutritionist, an exercise physiologist, and a social worker [18]. A psychiatrist or psychologist may also be part of the team. The main role of the physical or respiratory therapist is to carry out the prescribed exercise program (Table 149.4), whereas the occupational therapist evaluates the patient’s environment and ADLs and makes recommendations for adaptive equipment to optimize ADLs. The respiratory therapist also teaches breathing exercises as well as proper use of aerosolized medications and oxygen. An exercise physiologist prescribes and monitors the therapeutic exercise program. The nutritionist formulates the nutritional goals and educates on proper diet. The social worker assesses any need for home services and counsels and instructs in coping strategies, especially if a psychiatrist or psychologist is not part of the team. All members’ assessments are integrated into specific treatment plans.
Table 149.4
Types of Exercise [1,2,8,12,13,24,25]
Type of Exercise | Example |
Ventilatory muscle training | Inspiratory resistive exercise: maximum sustained ventilation, inspiratory resistive loading, inspiratory threshold loading, sustained hyperpnea |
Strength training | Upper extremity exercise: pulleys, elastic bands, supervised circuit training, weightlifting with low resistance |
Lower extremity exercise: supervised circuit training, weightlifting with low resistance | |
Endurance training | Upper extremity exercise: unsupported upper extremity activities ranging from activities of daily living to athletic activities, supervised arm cycling, low-impact aerobics, pool therapy |
Lower extremity exercise: incremental treadmill program, supervised walking, cycling and stair climbing program, low-impact aerobics, pool therapy |
Setting
Most PR programs are carried out in the outpatient setting, although inpatient and home settings are also possibilities. Inpatient- and outpatient-based programs facilitate interaction with a more complete PR team in a safe environment. Inpatient PR is usually reserved for patients with severe impairments and multiple comorbidities or for difficult-to-transport severely disabled patients. Home-based rehabilitation is more convenient for the severely disabled, but team interaction is limited. PR is beneficial irrespective of setting [1,18–20]. Indeed, home-based PR can be of equivalent benefit to hospital-based programs [20]. Telemonitoring may now allow a hybrid of home- and hospital-based monitoring as well as enhanced clinical data collection and more prompt interventions from the PR team. Domiciliary telemonitoring and home-based PR may decrease hospitalizations for respiratory complications [21]. Whereas efficacy of PR for patients with IPF is limited, it seems that many would benefit. Swigris [22] reported an improved sense of well-being, a greater sense of control, more energy, and a better general outlook on life. Outpatient PR programs also resulted in improvements in 6-minute walk distance and health-related quality of life for IPF patients, although pulmonary function and oxygenation did not improve [23–25]. Home-based programs may be equally effective [26]. Larger controlled studies are needed.
Rehabilitation Treatment Modalities
Because there are insufficient resources to provide PR to all symptomatic patients, attention to the following increases the likelihood of success: the patient’s respiratory condition should be stable on prescribed medical therapy including supplemental oxygen, with demonstrated adherence to the medical regimen before enrollment in PR; underlying medical conditions (e.g., cardiac disease or psychiatric illness) should be stable; and no medical conditions or physical impairments should be present that would interfere with the PR process [1]. The patient also needs to be able to understand the educational content of the program and be motivated and willing to devote the necessary time. PR dropout rates are highest among current smokers [27,28].
Although cigarette smoking is sometimes considered a contraindication to PR, clinical and physiologic gains can be similar in smokers and nonsmokers [27–29]. A trial of smoking cessation can be offered as an index of the patient’s motivation for PR [29].
Morbidity due to trapping of airway secretions may be relieved by the many secretion mobilization systems now available, but there is no clear evidence that one system works better than any other. The least expensive and simplest methods to supplement airway secretion mobilization efforts, such as use of a flutter value, positive expiratory pressure mask, and chest vibrators, are probably as effective as the expensive chest vibrating and oscillating devices [30]. Supplemental respiratory therapeutic secretion mobilization methods that can be taught include chest percussion and postural drainage, huffing, and active cycle of breathing. The last is the most inexpensive technique of airway secretion mobilization because no assistive device is used. The patient simply breathes slowly and shallowly at lung volumes well below functional residual capacity. He or she gradually increases tidal volumes to approach functional residual capacity and, once reaching it, takes a deep breath and “huffs” out secretions. Other important strategies for these patients are to administer and to monitor compliance with medications.
Carefully prescribed maximally intense anaerobic exercise over 30- to 45-minute periods provides the greatest benefits for reducing dyspnea and respiratory rate and increasing exercise tolerance, maximum oxygen consumption, 6- and 12-minute walk distance, ADLs, work output, mechanical efficiency, and possibly gas exchange while decreasing anxiety and depression [31–35].
For advanced patients who cannot tolerate high-intensity training, low-intensity training can be prescribed on the basis of objective or subjective measures. Objective measures involve calculating or measuring the maximal oxygen consumption or maximum heart rate. If open-circuit spirometry and metabolic cart are available, specific target intensity may be 50% of peak rate of oxygen uptake. Heart rate parameters may be most useful for patients with cardiac conditions. Several formulas are used. One is the desired exercise intensity multiplied by the maximum predicted heart rate. Hence, if the desired exercise intensity is defined as 60% of maximum predicted heart rate (HR), then
< ?xml:namespace prefix = "mml" />
Another is the Karvonen formula. For the target HR range of 50% to 85%:
Initial targets can be 50% (range, 50% to 80%) of either objective measure or the level tolerated by the patient [36].
When objective measures are not applicable, as in the case of patients taking negative chronotropic medications (e.g., beta blockers or calcium channel blockers) and heart transplant patients, subjective measures may be more predictive of exercise tolerance. In addition, because patients are often limited by exertional dyspnea, subjective measures may be more desirable [36].
Subjective measures of exercise tolerance, such as the Borg Rating of Perceived Exertion Scale or dyspnea rating scale, provide patient feedback based on symptoms alone. The Borg Rating of Perceived Exertion Scale from 6 to 20 is linearly related to heart rate. This is illustrated by multiplying the chosen scale number by 10 to obtain the estimated predicted heart rate. For example, when the patient chooses the number 10 on the scale to describe exertion symptoms, heart rate is estimated by the following equation:
The original Borg scale uses this method [36,37].
Daily activities in mobility and exercise are tailored to the patient’s form of mobility and baseline level of function. Mobility and endurance exercise programs can include walking, stair climbing, low-impact aerobics, stationary bicycling, and pool activities. For mobility, work, and recreational pursuits, assistive devices to improve ADLs can include wheelchair, walker, or cane. Strength training increases ADLs, mobility, and specific occupation-related tasks. Intermingled with endurance, strength, and task-specific training are energy conservation techniques that provide the patient with more energy efficient methods to perform daily activities. Increased endurance for exercise can occur independently of changes in ventilatory muscle endurance. The patient is made responsible for a progressive program to reinforce adherence and independence.
Breathing retraining goals modify the breathing pattern to decrease the work of breathing and to improve cough. Pursed-lip breathing and diaphragmatic breathing decrease the respiratory rate, coordinate the breathing pattern, and tend to prevent collapse of smaller bronchi. Air shifting is performed several times per hour. It involves a deep inspiration that is held with the glottis closed for 5 seconds. The air shifts to lesser ventilated areas of the lung and may help prevent microatelectasis. The subsequent expiration is through pursed lips. Pursed-lip breathing aids in relaxation as well. Other relaxation exercises, such as Jacobson exercises and biofeedback, can be used to decrease tension and anxiety [38,39].
For hypercapnic patients, interspersing periods of respiratory muscle rest with exercise of specific respiratory muscle groups is a principle of PR. Rest can be achieved by overnight use of nasal bilevel positive airway pressure, which improves daytime gas values, increases vital capacity, decreases fatigue, and increases well-being and quality of life [40,41].
After the acute rehabilitation period, continued surveillance and attention to abstinence from smoking, bronchial hygiene (Table 149.5), breathing retraining, physical reconditioning, oxygen therapy, and airway secretion mobilization have been shown to reduce hospital admissions, length of hospital stays, and cost [42,43]. The benefits of PR therapeutic exercise on exercise performance and quality of life are greatest during the first year and last up to 5 years [44–46].
Table 149.5
Pulmonary Hygiene Options [11,14,43]
Inhaled Treatments
Bronchodilators
Mucolytics
Methods of Airway Secretion Elimination
Oral, nasal, or transtracheal suctioning
Chest percussion and postural drainage
Positive expiratory pressure breathing
Flutter mucus clearance devices
Mechanical vibration devices to the chest wall
Intrapulmonary percussive ventilation with aerosolized medications
Mechanical insufflation-exsufflation applications
Autogenic drainage
Manual assisted cough
Abdominal binder
So far, there is not enough evidence to support improved survival with PR. As reported by Troosters and colleagues [47], in a pooled analysis of some small studies, 1-year to 18-month mortality risk was 7.8% in the PR group and 9.9% in the control group. The pooled odds of dying in the PR group was 0.69 compared with the control group. This suggests that PR reduces the short-term risk of dying by 31%, although this was not statistically significant. In 2011, a Cochrane review concluded that PR after an acute exacerbation of COPD was safe and associated with a reduction in mortality [48].
Surgery
Lung volume reduction surgery is an option for patients with predominantly upper lobe emphysema and low baseline exercise capacity. Results from the National Emphysema Treatment Trial demonstrated that lung volume reduction surgery in this subset of patients improved exercise capacity and quality of life and reduced mortality compared with a similar group of patients treated medically and that this benefit was maintained on a 5-year follow-up assessment [49]. Lung volume reduction surgery is contraindicated in patients with low FEV1, homogeneous emphysema, or very low carbon monoxide diffusing capacity because of the increased risk of death after surgery [50]. More recently, lung volume reduction by bronchoscopic techniques has been introduced, and studies of the effectiveness of this approach are ongoing.
Lung transplantation is an option for selected patients with COPD or IPF. Appropriate patients for lung transplantation have severe disease as defined by a low FEV1% predicted (< 25%), resting hypoxemia, hypercapnia, and secondary pulmonary hypertension. Referral for lung transplantation should be considered for COPD patients with progressive deterioration despite optimal treatment. After lung transplantation, quality of life and functional capacity have been demonstrated to improve [51]. Appropriate IPF patients for transplantation have a lung diffusion capacity for carbon monoxide of less than 39% of predicted, a decrement in FVC of more than 10% during 6 months of follow-up, a decrease in oxygen saturation below 88% during a 6-minute walk test, and honeycombing on HRCT. Five-year survival rates after lung transplantation in IPF are about 50%, with improvement demonstrated in oxygenation and spirometry, lung volumes, and diffusing capacity [52].
Potential Treatment Complications
Treatment complications can result from oxygen toxicity, barotrauma from ventilator use, and patient comorbidities such as concomitant cardiac or atherosclerotic peripheral vascular disease and pharmacologic treatment. Routine evaluation of a patient’s medication profile by the treating clinician is necessary. Immobility due to muscle weakness or acute illness can also exacerbate pulmonary secretion stasis and cause deep venous thromboses, cardiac deconditioning, skin ulceration, and bone decalcification. Each individual’s progress with mobilization, exercise, and daily activity facilitation programs is monitored, and prescriptions are modified accordingly.