CHAPTER 127
Deep Venous Thrombosis
Definition
Venous thromboembolism is a major cause of mortality and morbidity and is manifested by deep venous thrombosis (DVT) and pulmonary embolism. This chapter is limited to the discussion of DVT. DVT occurs when a fibrin clot abnormally occludes a vein in the deep venous system, predominantly in the lower extremities. The prevailing theory explaining the development of DVT is known as Virchow triad, which includes alterations in blood flow (stasis), vascular endothelial injury, and hypercoagulation disorder. The risk for development of DVT varies according to specific characteristics of the patient, the medical condition, or the surgical procedure (Table 127.1). Conditions that may increase the risk for development of DVT are advanced age, morbid obesity, varicose veins, prolonged immobility, pregnancy, malignant disease, stroke, inflammatory bowel disease, congestive heart failure, and previous DVT. Certain hereditary conditions may also predispose to development of DVT, such as deficiencies in protein C and protein S and familial thrombophilia. Acquired deficiencies of the natural anticoagulant system include antibodies directed against antiphospholipid and heterozygous factor V Leiden mutations [1].
Table 127.1
Risk Factors for Deep Venous Thrombosis
Patient Factors | Diseases | Procedures |
Age > 40 years Obesity Varicose veins Prolonged immobilization Pregnancy High-dose estrogen therapy Tamoxifen Bevacizumab Previous deep venous thrombosis |
Thrombophilia Antithrombin III, protein C, protein S deficiency Antiphospholipid antibody, lupus anticoagulant Malignant disease Major medical illness Trauma Spinal cord injury Paralysis |
Pelvic surgery Lower limb orthopedic surgery Neurosurgery |
Modified from Sokolof J, Knight R. Deep venous thrombosis. In Frontera WR, Silver JK, Rizzo TD Jr, eds. Essentials of Physical Medicine and Rehabilitation, 2nd ed. Philadelphia, WB Saunders, 2008.
Patients can be categorized according to their risk for development of DVT [2] on the basis of the type of surgical procedure; orthopedic patients carry the highest risk [3] (Table 127.2). It is believed that orthopedic procedures carry such a high risk for DVT because the mechanical destruction of bone marrow during most orthopedic procedures causes intravasation of marrow cells and cell fragments and elevations of plasma tissue factor [4]. Plasma tissue factor is a potent trigger of blood clotting [5] and is found in high concentrations in bone marrow and the adventitia surrounding the major blood vessels and the brain, which places neurosurgical patients at great risk for development of DVT. After neurosurgery, the incidence of DVT has been reported to be as high as 50% [6]. Risk factors that increase the rates of DVT in neurosurgery patients include intracranial surgery, malignant tumors, duration of the surgery, and presence of paresis or paralysis of the lower limbs [7]. Patients can remain in a hypercoagulable state up to 5 weeks postoperatively [8]. In addition to surgical patients, victims of orthopedic and neurologic trauma are at great risk for development of DVT, especially if long bone fracture or paralysis is sustained. Patients who suffer injury to the spinal cord are at high risk for DVT because of stasis and hypercoagulability.
Symptoms
Venous thrombosis often occurs asymptomatically. Symptoms of DVT may include ipsilateral lower extremity edema, fever, extremity warmth, and pain. Symptoms do not rule in or rule out DVT but can serve only as a trigger for further diagnostic inquiry.
Physical Examination
The classic signs of DVT are tenderness, ipsilateral swelling, and warmth. A palpable cord can sometimes be felt, which reflects a thrombosed vein. In the past, emphasis was placed on the presence of Homan sign and calf tenderness in making a clinical diagnosis of DVT; however, these physical examination findings have been found to be nonspecific with poor positive predictive values [9]. Significant asymmetric calf edema is an important sign and can be determined by taking the circumferential measurement of the calf 10 cm below the tibial tuberosity. A 3-cm difference in calf girth is considered a significant clinical difference. When it is massive, the swelling can obstruct not only venous outflow but arterial inflow, leading to phlegmasia cerulea dolens due to ischemia. Here, the leg is usually blue and painful.
Like symptoms, physical examination findings are not sensitive or specific. In more than 50% of the instances of DVT, physical examination findings are normal.
Functional Limitations
DVT rarely causes functional compromise, except calf pain during walking. Absolute bed rest is generally not indicated, and early walking is safe in patients with acute DVT and may help reduce acute symptoms [10]. However, patients should suspend their lower extremity exercise program until they are fully anticoagulated.
Diagnostic Studies
The Wells prediction rules (Table 127.3) are a group of clinical characteristics that are useful in estimating the pretest probability of DVT. High-quality evidence exists to support the validity of these rules, and their use is recommended as a practice guideline by the American Academy of Family Physicians and the American College of Physicians [11]. They are easily implemented before more definitive testing is performed on patients [12].
Table 127.3
Wells Prediction Rules: Clinical Evaluation Table for Predicting Pretest Probability of Deep Venous Thrombosis
Clinical Characteristic | Score |
Active cancer (treatment ongoing, within previous 6 months, or palliative) | 1 |
Paralysis, paresis, or recent plaster immobilization of the lower extremities | 1 |
Recently bedridden > 3 days or major surgery within 12 weeks requiring general or regional anesthesia | 1 |
Localized tenderness along the distribution of the deep venous system | 1 |
Entire leg swollen | 1 |
Calf swelling 3 cm larger than asymptomatic side (measured 10 cm below tibial tuberosity) | 1 |
Pitting edema confined to the symptomatic leg | 1 |
Collateral superficial veins (nonvaricose) | 1 |
Alternative diagnosis at least as likely as deep venous thrombosis | − 2 |
From Wells PS, Anderson DR, Bormanis J, et al. Value of assessment of pretest probability of deep-vein thrombosis in clinical management. Lancet 1997;350:1795-1798.
Clinical probability: low, ≤ 0; intermediate, 1-2; high, ≥ 3. In patients with symptoms in both legs, the more symptomatic side is used.
Invasive and noninvasive diagnostic tests are available to screen for DVT. These include contrast venography, compression ultrasonography, impedance plethysmography, D-dimer testing, and magnetic resonance venography.
Contrast venography is an invasive test that is considered the “gold standard” for the diagnosis of DVT and is the only test that can reliably detect DVT isolated to the calf veins, the iliac veins, and the inferior vena cava (Figs. 127.1 and 127.2). The drawbacks to venography are its technical complexity, the requirement for the use of contrast media, the risk of allergic reaction, and the patient’s discomfort. Therefore it is not recommended as an initial screening test.
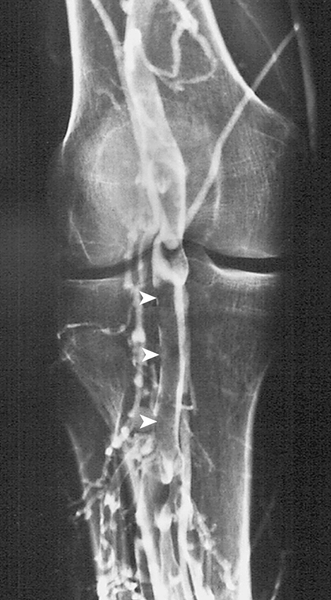
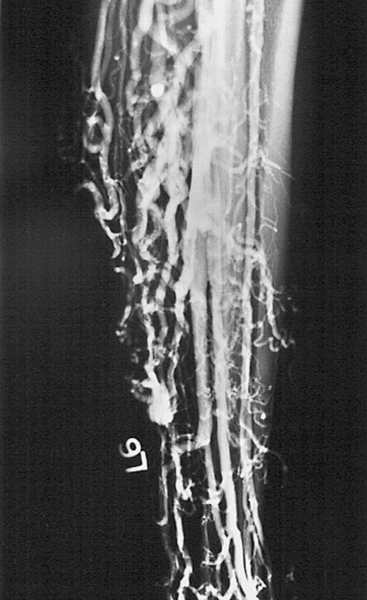
Compression ultrasonography (real-time, B-mode venous) is the procedure of choice for the investigation of patients with suspected DVT. Venous ultrasonography allows direct visualization of the vein lumen. The inability to compress the lumen of the vein is the main criterion for a positive test result. Other adjunctive findings include vein distention, absence of flow, echogenic signals within the vessel lumen, and visualization of filling defects by color Doppler studies. Systematic reviews have demonstrated high sensitivities and specificities for the diagnosis of DVT in the proximal lower extremity by ultrasonography. However, sensitivities are poor for determination of the presence of calf vein thrombosis. Visualization of calf veins by ultrasonography is technically more difficult and less reliable than diagnosis of venous thrombus in the area between the trifurcation of the popliteal vein and the femoral vein in the groin.
Impedance plethysmography, a noninvasive diagnostic test for detection of blood vessel occlusion, determines volumetric changes in the limb by measuring changes in its girth as indicated by changes in the electric impedance of mercury-containing polymeric silicone tubes in a pressure cuff. The method is based on the principle that any circumferential rate of change in a limb segment is directly proportional to the volumetric rate of change, which in turn reflects occlusion of venous and arterial blood flow. However, the technique does not accurately identify the presence or absence of partially obstructing thrombi in major vessels [13]. Impedance plethysmography has limitations including the possibility of false-positive results because of arterial insufficiency and muscle tension [14].
D-dimer assay has emerged as a method to help predict the presence of DVT. D-dimer is a degradation product of the cross-linked fibrin blood clot and is typically elevated in patients with DVT. However, D-dimer levels may also be elevated in a variety of nonthrombotic disorders, including recent major surgery, hemorrhage, trauma, malignant disease, and sepsis. Because of the high sensitivity and low specificity of the D-dimer assay, it is a good tool for exclusion of DVT if the test result is negative. However, a high level does not have as much clinical value.
The first step in the diagnostic approach is the determination of risk. Patients can be separated by clinical criteria into high-, moderate-, and low-risk categories. All symptomatic patients thought to have DVT should, at the very least, undergo venous ultrasound imaging of the proximal venous system. Patients at moderate or high risk should have the ultrasound study repeated in 1 week. DVT also may be ruled out on the basis of a negative result of the D-dimer assay. If the D-dimer assay result is positive, a follow-up ultrasound study in 1 week is indicated.
Magnetic resonance venography is as accurate as contrast venography for the diagnosis of DVT. This was illustrated in a study that evaluated 85 patients thought to have DVT, all of whom underwent both magnetic resonance and contrast venography [15]. Although the diagnostic accuracy of magnetic resonance venography is comparable to that of contrast venography, outcome data are lacking. In addition, the present high cost of magnetic resonance venography makes it unlikely that it will gain prominence as a noninvasive test for DVT. However, magnetic resonance venography is a useful approach when contrast venography is required but precluded because of allergy to contrast media.
Treatment
Initial
The most appropriate choice of prophylaxis depends on the clinical scenario and the risk-benefit profile for a particular patient. The prophylactic options that are currently available for hospitalized medical patients include low-dose unfractionated heparin (UFH), low-molecular-weight heparin (LMWH), fondaparinux, aspirin, warfarin, intermittent pneumatic compression, and graduated compression stockings [16]. UFH and LMWH have been shown to reduce venous thromboembolic risk in hospitalized medical patients, but neither agent altered mortality [17]. On direct comparison, LMWH was more effective than UFH in preventing DVT [17]. Large meta-analyses comparing LMWH with UFH in general and orthopedic surgery have shown that LMWH is safer and more efficacious than UFH [18].
Fondaparinux has been approved by the Food and Drug Administration for the prophylaxis of DVT in patients undergoing surgery for hip fracture, hip replacement, or knee replacement; for extended DVT prophylaxis after hip fracture surgery; and for patients at risk for thromboembolic complications after abdominal surgery [19]. The approved dose for prevention of postoperative DVT is 2.5 mg subcutaneously once daily (in adults over 50 kg; prophylaxis is contraindicated in patients <50 kg), to be initiated 6 to 8 hours after completion of surgery.
Antiplatelet agents such as aspirin reduce the risk of DVT and pulmonary embolism in some patients; however, there is little evidence that antiplatelet agents have a significant effect on the prevention of venous thromboembolic events in medical patients. Also, low-dose aspirin did not significantly reduce the rate of recurrence of venous thromboembolism but resulted in a significant reduction in the rate of major vascular events, with improved net clinical benefit [19]. The 2012 American College of Chest Physicians guidelines did not recommend the use of aspirin, either alone or in combination, as prophylaxis against DVT in any medical patient group [20].
Low-dose warfarin is better than aspirin or placebo in patients with hip fractures but may not be satisfactory in preventing DVT after elective hip and knee surgery. The use of warfarin can be associated with a transient hypercoagulable state in the first 36 hours after administration because it causes a rapid decline in protein C levels before the anticoagulant effect, which does not occur until 36 to 72 hours after drug administration.
Mechanical DVT prophylaxis can be achieved with intermittent pneumatic leg compression, intermittent pneumatic foot compression, or graduated compression stockings. Intermittent pneumatic leg compression provides increases in peak velocity and flow in the common femoral vein and is better than placebo in preventing DVT. Intermittent pneumatic foot compression is a high-pressure system that exerts compression limited to the foot. These devices are best for patients who undergo lower extremity orthopedic surgery and cannot be fitted with the intermittent pneumatic leg compression devices. The intermittent pneumatic foot compression offers no advantage over LMWH in the prevention of DVTs, except for a lower rate of bleeding complications. Graded compression elastic stockings work by increasing venous blood flow velocity. Knee-length stockings are sized to fit, and they deliver graduated pressure of 40 mm Hg at the ankle, 36 mm Hg at the lower calf, and 21 mm Hg at the upper calf. Recent clinical practice guidelines from the American College of Chest Physicians recommend the use of either intermittent pneumatic compression or graduated compression stockings over no mechanical prophylaxis in acutely ill hospitalized medical patients at increased risk for thrombosis who are bleeding or are at high risk for major bleeding [20].
The optimal duration of pharmacologic prophylaxis varies with individual risk and clinical situation. Current standard of care is to stop prophylaxis 7 to 10 days after a surgical procedure or when medical patients are ambulating freely. After major orthopedic surgery, prolonged prophylaxis of 4 to 6 weeks is most advantageous. In patients with spinal cord injuries, prophylaxis is best maintained for 6 to 10 weeks.
In someone who presents with a DVT, the primary goal of treatment is to prevent extension of the clot, recurrence of the thrombosis, acute pulmonary embolism, and development of late complications of DVT (e.g., post-thrombotic syndrome, chronic thromboembolic pulmonary hypertension, and chronic venous insufficiency). The cornerstone of medical treatment of DVT is anticoagulation therapy. Anticoagulation therapy is indicated in patients with symptomatic proximal lower extremity DVT because pulmonary embolism may occur in up to 50% of untreated individuals [21]. One study showed death in 26% of patients who had clinically suspected pulmonary embolism and did not receive anticoagulation, compared with no deaths in the treatment group [22]. Current recommendations per the 2012 American College of Chest Physicians evidence-based clinical practice guidelines for antithrombotic and thrombolytic therapy are that patients with DVT or pulmonary embolism should be treated acutely with LMWH, fondaparinux, intravenous UFH, or dose-adjusted subcutaneous UFH [20]. An analysis of 16 systematic reviews of clinical trials revealed that there is high-quality evidence to support the use of LMWH over UFH in the treatment of established DVT [23]. Furthermore, the risk of major bleeding during initial therapy appears to be reduced with these agents compared with UFH [23]. When UFH is used, the dose should be sufficient to prolong the activated partial thromboplastin time to 1.5 to 2.5 times the mean of the control value, or the upper limit of the normal activated partial thromboplastin time range [24].
Many medical centers have adopted weight-adjusted nomograms to increase the likelihood of obtaining a therapeutic anticoagulation effect early. The nomogram that has been found to achieve the most rapid acquisition of the target activated prothrombin time is an initial bolus of 80 units/kg of UFH followed by an infusion rate of 18 units/kg per hour. Activated prothrombin time should be checked every 4 to 6 hours until a therapeutic range of 1.5 is achieved. The duration of UFH treatment ranges between 4 and 10 days. Patients with a large iliofemoral vein thrombosis or major pulmonary embolism require a 7- to 10-day course of heparin, with a delay in the initiation of warfarin until the activated prothrombin time is in the therapeutic range. Studies demonstrate that a 4- to 5-day course of heparin with warfarin administered within 24 hours of heparin initiation in patients without major pulmonary embolism of large proximal clots was as effective as 9 to 10 days of UFH alone.
LMWHs are fragments of UFH produced by either chemical or enzymatic depolymerization. LMWHs display improved bioavailability, dose-independent clearance, and more predictable dose response compared with UFH. Therefore, these agents can usually be given once or twice daily subcutaneously in weight-adjusted doses without laboratory monitoring. The U.S. Food and Drug Administration has approved two LMWHs, dalteparin (Fragmin) and enoxaparin (Lovenox), for perioperative DVT prophylaxis (Table 127.4). There are two other LMWHs available for use in DVT treatment: tinzaparin (Innohep) and nadroparin. Enoxaparin and tinzaparin are approved for DVT treatment in the United States and Canada. Dalteparin and nadroparin are approved for this use only in Canada. Ardeparin (Normiflo) is another LMWH previously used for DVT treatment, but it was later withdrawn from the market. Enoxaparin can be used for hospitalized patients with DVT with or without pulmonary embolism and for outpatient treatment of DVT without pulmonary embolism.
Table 127.4
U.S. Food and Drug Administration–Approved Uses of Low-Molecular-Weight Heparins
Name | FDA-Approved Indications | Dosage |
Dalteparin (Fragmin) | DVT prophylaxis after hip replacement | 5000 units SC daily; start 2500 units SC ×1 dose 4-8h postop; allow >6h between first and second doses |
Enoxaparin (Lovenox) | DVT prophylaxis after knee surgery | 30 mg SC q12h |
DVT prophylaxis after hip surgery | 30 mg SC q12h or 40 mg SC daily | |
DVT prophylaxis after abdominal surgery | 40 mg SC daily | |
Inpatient treatment of acute DVT with or without PE | 1 mg/kg SC q12h or 1.5 mg/kg SC daily | |
Outpatient treatment of acute DVT without PE | 1 mg/kg SC q12h |
From Sokolof J, Knight R. Deep venous thrombosis. In Frontera WR, Silver JK, Rizzo TD Jr, eds. Essentials of Physical Medicine and Rehabilitation, 2nd ed. Philadelphia, WB Saunders, 2008.
DVT, deep venous thrombosis; PE, pulmonary embolism; SC, subcutaneously.
Unmonitored outpatient therapy with LMWH is thought to be as safe and effective as in-hospital intravenous administration of UFH in patients with proximal DVT [25]. Like patients receiving UFH, those treated with LMWH should begin taking warfarin within 24 to 48 hours. LMWH can be discontinued after a minimum of 5 days, provided the international normalized ratio (INR) has been therapeutic for 2 consecutive days.
Thrombolytic therapy has a limited role in the treatment of DVT. It has been suggested that pharmacologic lysis of DVT could prevent post-thrombotic syndrome if complete lysis can be achieved before valve destruction occurs. However, thrombolysis, whether it is given systemically or by catheter, is expensive, the risk of bleeding complications is high, and the evidence of additional benefit is not convincing. Thrombolysis should be reserved for patients with massive iliofemoral thrombosis or unstable cardiac or pulmonary disease with no contraindications to thrombolytic therapy.
After initial treatment with LMWH, long-term anticoagulation therapy to prevent recurrent DVT is needed.
Oral anticoagulation with warfarin should prolong the INR to a target of 2.5 (range, 2-3), which generally is considered effective in preventing recurrent DVT, and risk of bleeding is lower than with higher INR levels. If oral anticoagulants are contraindicated or inconvenient, long-term therapy can be undertaken with adjusted-dose UFH, LMWH, or fondaparinux. Because of ease of use, especially in the outpatient setting, LMWH or fondaparinux is preferred to UFH [26].
Treatment duration ranges between 3 and 6 months; the short duration is reserved for those who had some risk factor (e.g., immobility) before surgery. Indefinite therapy is preferred in patients with a first unprovoked episode of proximal DVT, who have a greater relative risk of recurrent venous thromboembolism and a relatively lower concern about the burdens of long-term anticoagulant therapy [26].
The risk of recurrent DVT is serious on cessation of anticoagulation treatment. There is some evidence to suggest that post-treatment residual thrombus increases the risk of recurrent DVT and mortality [27]. The monitoring of serial D-dimer levels has been shown to be useful in determining the likelihood of DVT recurrence [28]. Treatment is indicated for at least 12 months in patients with recurrent DVT or for individuals with continuous risk factors for thromboembolic disease, such as malignant disease [25]. Untreated calf vein thrombosis does not commonly result in clinically significant pulmonary embolism unless the thrombus extends into the proximal venous segments, which occurs in about 25% of the cases. It is safe to monitor calf thrombi with serial venous ultrasound examinations or impedance plethysmography and to initiate therapy only if the thrombus extends into the popliteal or more proximal veins. Treatment of superficial venous thrombosis is usually not indicated. Pregnant women with DVT are classically treated with subcutaneous UFH or LMWH at full doses until delivery. Current recommendations indicate that the patient should be switched to UFH 2 weeks before the expected delivery, and a 4- to 6-week course of warfarin should be completed after delivery. Warfarin freely crosses the placental barrier, and therefore it is contraindicated during pregnancy but is safe for the mother and nursing child after delivery.
Rehabilitation
There is no strict contraindication to therapeutic exercises and ambulatory activities after DVT, but it is recommended that these activities be suspended until the patient is in the therapeutic range for heparin or has been receiving LMWH for 24 hours. On the other hand, physical and occupational therapy ordered immediately after high-risk surgical procedures can greatly improve a patient’s postoperative mobility and lessen the chance for development of DVT.
Procedures
Inferior vena caval filters are indicated in patients with DVT who have an absolute contraindication to anticoagulation, high risk of bleeding, or recurrent DVT despite adequate anticoagulation. Caval interruption has been found to be effective in preventing subsequent pulmonary embolism; however, this is counterbalanced by the increased incidence of recurrent DVT. Patients who have significant but temporary contraindications to the use of anticoagulants who receive caval interruption devices should begin taking anticoagulation medication as soon as possible after placement.
Surgery
Surgical removal of acute DVT by thrombectomy or embolectomy is rarely used. It should be considered only in patients with massive thrombosis and compromised arterial circulation who do not respond to or who have an absolute contraindication to thrombolytic therapy.
Potential Disease Complications
If untreated, proximal DVT is linked with a 10% immediate risk of fatal pulmonary embolism and approximately 20% higher risk for development of a severe post-thrombotic syndrome 5 to 10 years later [29] (Fig. 127.3). There are two predominant patterns of DVT: an ascending pattern, with DVT arising in the calf veins; and a descending pattern, with DVT occurring initially in the iliac or common femoral vein. The descending pattern more commonly results in pulmonary embolus. Post-thrombotic syndrome is a condition characterized by chronic edema and debilitating pain and can lead to ulceration, infection, or, in rare cases, amputation. Heparin use and the wearing of graduated compression stockings have been associated with a lower risk for development of post-thrombotic syndrome.
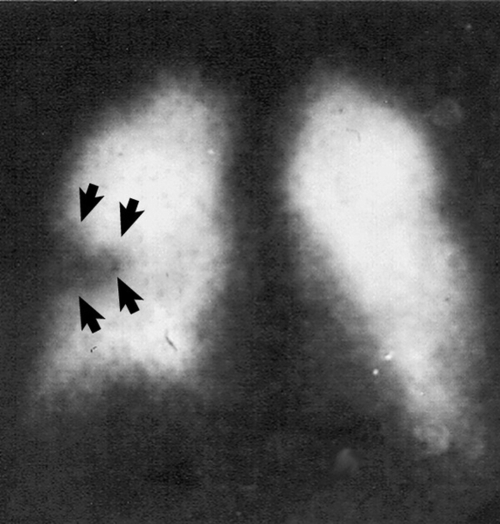
Potential Treatment Complications
All pharmacologic anticoagulation agents alter the hemostatic mechanisms to some extent by decreasing blood coagulation or platelet function, and all carry a risk of bleeding. Both UFH and LMWH are associated with similar increased risk of bleeding complications. Heparin-induced thrombocytopenia is a potential complication of heparin use and can be seen slightly less commonly with LMWH compared with UFH because of the lower affinity of LMWH for platelet binding. A diagnosis of heparin-induced thrombocytopenia is made when there is a 50% reduction in platelet count or a presence of antiplatelet antibodies. Once the diagnosis is made, all heparin products are contraindicated. Skin necrosis is a rare complication of warfarin use, and it can be prevented if high-dose warfarin is delayed until the activated prothrombin time is therapeutic with heparin. Complications from caval interruption include problems related to the deployment of the device: hemorrhage, hematoma, femoral artery injury, femoral nerve injury, infection, and site pain. Another potential complication is pulmonary embolism from embolization of the device itself or from failure of the device to capture an embolus. A clot-laden caval interruption device can impede venous flow and lead to lower extremity venous stasis and edema. Osteoporosis and risk of bone fracture are associated with long-term use of UFH [30], but the risk may be less with LMWH [31]. Contraindications to the use of warfarin include advanced liver disease, alcoholism, poor compliance with follow-up, poorly controlled hypertension, major bleeding, and pregnancy [32].